Abstract
Chronic rhinosinusitis (CRS) is a prevalent and burdensome condition worldwide, characterized by inflammation of the paranasal sinuses. Ideally, instead of treating CRS, we would identify ways to prevent the development of this chronic condition. Occupational exposures may be an excellent target for prevention. Occupational exposures have been shown to play a critical role in the pathogenesis of multiple lower airway diseases, such as asthma, silicosis, asbestosis, and hypersensitivity pneumonitis. However, evidence for the association between occupational exposures and the development of upper airway disease, like CRS, is less well-defined. This manuscript examines the association between occupational exposures and CRS. A scoping review of the literature following Preferred Reporting Items for Systematic Reviews and Meta-Analyses (PRISMA) guidelines identified 19 relevant studies. The populations examined and the methods and criteria used for defining CRS diagnosis and occupational variables significantly varied between the studies. Diagnosis of CRS was most often determined by self-reported symptoms or medical record review. Occupational variables ranged from employment status to occupation type to specific exogenous compounds encountered. Overall, substantial evidence demonstrates a general association between occupational exposures and CRS diagnosis; however, limitations in study methodologies, including variations in CRS diagnostic criteria, occupational exposures, assessment methods, and populations, hinder drawing more specific conclusions. Moving forward, rigorous research methodologies and standardized criteria are essential to draw conclusions supported by multiple studies. Critical components of future studies should include large, diverse populations, use of consensus CRS diagnostic criteria, and inclusion of many specific and quantitatively defined exposures. Ultimately, such efforts can help inform preventative measures and interventions for CRS, thus mitigating the burden of CRS on individuals and populations worldwide.
Keywords
Chronic rhinosinusitis, CRS, occupation, occupational exposureIntroduction
Chronic rhinosinusitis (CRS) is a burdensome condition characterized by inflammation of the paranasal sinuses and nasal passages lasting 12 weeks or longer. CRS impacts an impressive number of people worldwide, with a prevalence ranging from 5–10% depending on diagnostic criteria [1]. Common symptoms of CRS include rhinorrhea, nasal congestion, hyposmia, and facial pain, which can negatively impact patient quality of life, often leading to sleep and mood disturbances [2, 3]. Furthermore, CRS represents a significant economic burden with $4–12.5 billion in healthcare costs, 11.5 million missed workdays, and another $12.8–13 billion in indirect costs due to loss of productivity, among other factors, in the United States [4–6].
CRS is a heterogeneous disease encompassing various subtypes with diverse underlying pathogenic mechanisms. While the exact etiology remains unknown, dysregulation within the immune system, particularly involving Type 1 T-helper cells, Type 2 T-helper cells, and Type 17 T-helper cells, is thought to play a central role in its pathogenesis. These cells contribute to the inflammatory cascade within the sinonasal mucosa, possibly contributing to epithelial barrier dysfunction and impaired mucociliary clearance, all of which are implicated in the pathogenesis of CRS [7, 8]. However, the exact relationship between this immune response and other factors, such as predisposition factors and triggers, remains unclear. This presents a significant challenge in managing and especially preventing disease.
There has been significant research linking exposures to lower airway disease. For example, common ambient air pollutants such as particulate matter (PM), ozone, and nitrogen oxides have been associated with increased incidence and disease severity of asthma, bronchitis, and chronic obstructive pulmonary disease (COPD), especially in vulnerable populations [9–11]. As the entrance to the lower airway, the nose and sinuses are similarly exposed; however, less evidence describes the association between environmental and occupational exposures and CRS. Of the commonly studied air pollutants such as carbon monoxide, lead, nitrogen oxides, ozone, sulfur oxides, and particle pollution (PM), PM has been the most well-studied in association with CRS, although other pollutants such as nitrogen oxides, ozone, and sulfur dioxide have also been found to be associated with increased CRS incidence [12–14]. Mechanistically, several in-vitro studies have corroborated these clinical associations by demonstrating that PM and ozone exposure can cause nasal epithelial cell barrier disruption and provoke a local pro-inflammatory response [15–17].
In addition to ambient air pollution, unique compounds encountered in occupational environments can negatively impact the upper and lower respiratory tracts and contribute to disease. Again, there is significant data to suggest that occupational exposures, like animal proteins, chemicals, and metals, can exacerbate lower airway disease [18–20], but the role of occupational exposures in CRS is more limited. This paper explores the current evidence regarding occupational exposures and their impact on CRS to identify key concepts and limitations and suggest future research strategies.
Methods
Search methodology, inclusion criteria, and data collection
A scoping literature review was performed following Preferred Reporting Items for Systematic Reviews and Meta-Analyses (PRISMA) guidelines [21]. A research librarian queried three databases, PubMed, EMBASE, and Scopus, for relevant articles. Inclusion criteria included studies that analyzed the effects of occupational domains on the prevalence, incidence, or severity of CRS. For inclusion, CRS had to be defined/diagnosed by methods such as the European Position Paper on Rhinosinusitis and Nasal Polyps (EPOS) symptom criteria [22], the International Consensus Statement on Allergy and Rhinology (ICAR) [23], endoscopic findings, computed tomography (CT) scan findings, International Classification of Diseases (ICD) codes in medical records, or the need for endoscopic sinus surgery (ESS). Exclusion criteria included articles that were non-English, non-original (e.g., review articles, editorials), basic science, lacked occupational domains, lacked a clear definition of CRS, or focused on non-CRS sinus disease. In-depth inclusion and exclusion criteria and the complete database search strategies are in the supplementary file (Supplementary material). Two authors (A.S.M, C.P.W) independently screened all identified articles in Covidence for initial inclusion. A third author (E.H.B) acted as an arbiter for any disagreements.
Data extraction, analysis, and quality assessment
PRISMA search methodology was followed for the study (Figure 1) [21]. Three investigators (A.S.M, C.P.W, E.H.B) extracted and described data using Microsoft Excel. Metrics extracted from each study included publication journal, publication year, publication country, study design, country of study population origin, sample size, age of study population, occupational variables, study methods used for measuring occupational variables, study criteria for defining CRS, analysis method, CRS outcomes, and limitations. Using the Oxford Centre for Evidence-based Medicine—2011 Levels of Evidence guidelines, each article was assigned a “level of evidence” based on the study design [24].
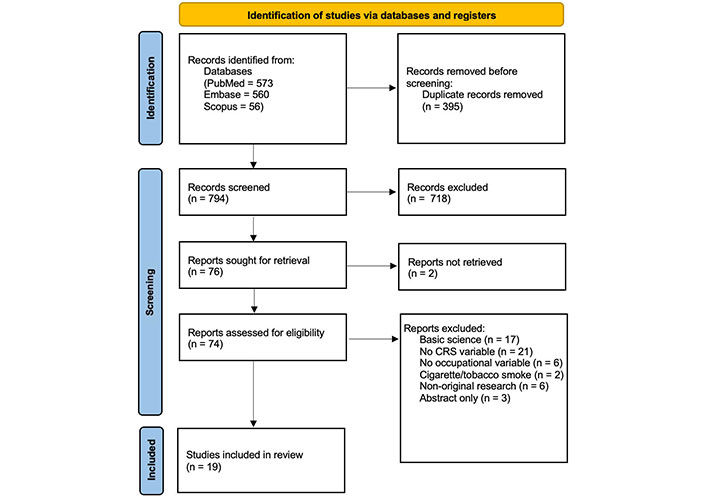
Preferred Reporting Items for Systematic Reviews and Meta-Analyses (PRISMA) flow diagram of literature search and study inclusion
Results
The literature query resulted in 1,189 total records. After removal of duplicates, 794 unique abstracts were screened. Nineteen studies met criteria and underwent data extraction (Figure 1). Study designs included prospective cohort, retrospective cohort, nested case-cohort, cross-sectional, and case-control, all of which were deemed to have level 3 evidence.
The 19 articles examined several types of occupational variables, ranging from occupational statuses like employed vs. unemployed to occupation types such as sales workers, agricultural workers, and homemakers, to specific exogenous compounds encountered through occupation like cooking fumes, paint/varnish, and metal smoke. Some studies further broke down occupational exposure into levels of exposure based on the duration of the exposure. Most of the studies determined these occupational variables by participant self-reports through surveys or interviews (11/19 articles, 57.9%) [25–35]. Other methods included abstraction from medical records or health databases (4/19, 21%) [36–39], employment records (3/19, 15.8%) [40–42], and confirmation via subject legal representatives (1/19, 5.3%) [43].
Diagnostic criteria for CRS varied among studies. Ten of the 19 studies (52.6%) followed, or partially followed, the EPOS or the ICAR for CRS diagnosis [25–28, 33–35, 39, 40]. Both of these require patients to have two or more symptoms of nasal congestion/blockage, nasal discharge, facial pain/pressure, and smell loss lasting more than 12 weeks, one of which must be either nasal congestion/blockage or nasal discharge, and evidence of objective disease on nasal endoscopy or CT scan [22, 23]. More specifically, seven of these ten used surveys that only covered EPOS symptom criteria [25, 26, 28, 29, 34, 35, 40]. Two studies required full EPOS criteria, including objective evidence [33, 39], while one study abstracted the CRS diagnosis and objective evidence from medical records but specified that the diagnosis had been previously made based on EPOS criteria [27]. Of the remaining nine studies, two (10.5%) diagnosed CRS based on objective findings alone [36, 41]. Three (15.8%) abstracted CRS diagnoses from medical records without specification of diagnostic criteria [30, 38, 43]. Three (15.8%) abstracted CRS diagnoses from medical records but required objective evidence to confirm the diagnosis [32, 37, 42]. One (5.3%) asked participants if they had a CRS diagnosis via survey [31]. More information about study characteristics can be found in Table 1.
Major characteristics of 19 studies
Study [citation number] | Journal | Year | Country | Study type | Sample size | Population country | Population age | Occupational variables | Occupational measurement method | CRS criteria | CRS diagnosis method | Statistical methods | Oxford level of evidence |
---|---|---|---|---|---|---|---|---|---|---|---|---|---|
Occupation status, occupation type, or occupational compound | |||||||||||||
Koh et al. [31] | American Journal of Industrial Medicine | 2009 | South Korea | Cross-sectional (three-time points: 1998, 2001, 2005) | 1998: 20,8292001: 20,4682005: 18,266 | South Korea | Range 20–59 years | Occupation type: legislators and senior officials and managers, professionals, technicians and associated professionals, clerical workers, service workers, sales workers, skilled agricultural and forestry and fishery workers, plant or machinery operators and assemblers, elementary occupations, and homemaker | Interview & questionnaire response | Self-report of CRS | Interview & questionnaire response | Multivariable Poisson regression model | 3 |
Hox et al. [30] | Allergy | 2012 | Belgium | Case-control | 546 | Belgium | Range 18–65 years | Occupational substances: relevant or irrelevantCommonly reported substances: bleach, inorganic dust, paints, cement, thinner, ammonia, white spirit, fuel gas, and acetone | Survey | Prior ESS for CRS | Medical records | Multivariable Poisson regression model | 3 |
Thilsing et al. [35] | American Journal of Industrial Medicine | 2012 | Denmark | Cross-sectional | 3,099 | Denmark | Mean 48.1 years | Occupation type: blue collar or white collar (classified by ISCO-88 coding system)Occupational substances: gases, fumes, dust, and smoke; HMW agents, LMW agents, and mixed environments (classified by asthma-specific job exposure matrix) | Survey | EPOS symptom criteria | Survey | Generalized linear models for the binomial family | 3 |
Gao et al. [28] | Respiratory Research | 2016 | China | Cross-sectional | 10,633 | China | 0–14 years: n = 64415–34 years: n = 3,13635–59 years: n = 4,834≥ 60 years: n = 2,005 | Occupation type: clearance-related jobs, healthcare-related jobsOccupational substance: dust, poisonous gas, pets, carpet, and damp/moldy environment | Survey | EPOS symptom criteria | Survey | Univariate & multivariate logistic regression | 3 |
Hoffmans et al. [29] | PLOS One | 2018 | Netherlands | Cross-sectional | 8,347 | Netherlands | Mean 45.4 years | Occupation status: employed, unemployed, self-employed, not working because of poor health, full-time house person, full-time student, retired, and other | Survey | EPOS symptom criteria | Survey | Univariate & multivariate logistic regression | 3 |
Clarhed et al. [26] | Journal of Occupational and Environmental Medicine | 2018 | USA | Cross-sectional | 14,906 | Norway | Range 16–50 years | Occupational substance: cooking fumes, car/engine exhaust, strong acids, stone dust, flour/grain dust, wood dust, paper dust, metal dust, cleaning agents, super glue, paint/varnish, welding/metal smoke, sewage, hair care products, animals, moisture/mold/mildew, cold work, and physically strenuous work | Survey | EPOS symptom criteria | Survey | Univariate & multivariate logistic regression | 3 |
Veloso-Teles et al. [41] | Rhinology Online | 2018 | Portugal, Denmark | Cross-sectional | 316 (textile workers = 215; retail workers = 101) | Portugal | Textile workers mean 50 yearsRetail workers mean 41 years | Occupation type: textile workers and retail workersOccupational substance: dust | Interview | Lund Kennedy endoscopic score | Physician visit | Comparative statistics | 3 |
Velasquez et al. [39] | International Forum of Allergy and Rhinology | 2020 | USA | Retrospective cohort | 234 | USA | Mean 51.3 years | Occupational substance: VGDFFiM and diesel fumes | Medical records | ICAR—symptom and objective evidence criteria | Medical records | Comparative statistics | 3 |
Clarhed et al. [25] | Rhinology Online | 2020 | Netherlands | Cross-sectional | 7,952 | Norway | Range 16–50 years | Occupational substance: cooking fumes, car/engine exhaust, strong acids, stone dust, flour/grain dust, wood dust, paper dust, metal dust, cleaning agents, super glue, paint/varnish, welding/metal smoke, sewage, hair care products, animals, moisture/mold/mildew, cold work, and physically strenuous work | Survey | EPOS symptom criteria | Survey | Univariate & multivariate logistic regression | 3 |
Dietz de Loos et al. [27] | Rhinology Online | 2021 | Netherlands | Cross-sectional | 364 | Netherlands | Mean 56 years | Occupational substance: relevant or irrelevantCommonly reported substances: solvents, cleaning products, reactive chemicals, welding fumes/metal dust, combustion engine exhaust, medications, ammonia, flour, flowers, inorganic dust, latex, animals, and cement | Survey | EPOS symptom and objective evidence criteria | Medical records | Univariate & multivariate logistic regression | 3 |
Nynäs et al. [33] | Healthcare (Basel) | 2021 | Finland | Prospective cohort | 99 | Finland | Mean 44 years | Occupational substance: mold/moisture damage | Interview | EPOS symptom and objective evidence criteria | Physician visit | Descriptive statistics | 3 |
Tai et al. [34] | ENT Journal | 2024 | Korea | Cross-sectional | 26,335(control—24,054; CRS—2,124; CRS + asthma—157) | Korea | Control mean 49.8 yearsCRS mean 51.4 years | Occupation type: indoor occupation, outdoor occupation, and unemployed | Survey | EPOS symptom criteria | Survey | Multivariate Logistic regression model | 3 |
Military occupation | |||||||||||||
Balali-Mood et al. [36] | Human and Experimental Toxicology | 2011 | Unknown | Prospective cohort | 43 | Iran | Mean 50.6 years | Occupation type: veteransOccupational substance: sulfur mustard | Medical records | CT scan (normal, partial opacity, complete opacity, and partial and complete opacity) | Physician visit | Spearman correlation tests | 3 |
Elam et al. [38] | Military Medicine | 2022 | England | Case-control | 798 (CRS = 399; cerumen impaction = 399) | USA | CRS mean 30.98 yearsCerumen impaction mean 28.77 years | Occupation type: active-duty service membersOccupational substance: PM2.5, PM10, NO2, and ozone | Military Health Systems; EPA | Prior CRS diagnosis | Medical records | Conditional logistic regression | 3 |
Disaster response occupations | |||||||||||||
Cho et al. [37] | Respiratory Medicine | 2014 | Unknown | Nested case cohort | 179 (CRS = 76) | USA | Not specified | Occupation type: firefighterOccupational substance: WTC PM | FDNY medical monitoring and treatment program database | Prior CRS diagnosis with objective evidence | Medical records | Kaplan Meier | 3 |
Weakley et al. [42] | Occupational and Environmental Medicine | 2016 | USA | Retrospective cohort | 9,848 | USA | Mean 40.1 years | Occupation type: WTC disaster-response workersOccupational substance: WTC PM | Employee records | Prior CRS diagnosis with objective evidence | Medical records | Piecewise exponential survival models | 3 |
Liu et al. [32] | Frontiers in Public Health | 2017 | USA | Prospective cohort | 8,968 | USA | Median 39.6 years | Occupational type: firefighterOccupational substance: WTC PM | Survey | Prior CRS diagnosis with objective evidence | Medical records | Piecewise exponential survival models | 3 |
Putman et al. [40] | Occupational and Environmental Medicine | 2018 | USA, Belgium | Prospective cohort | 11,926(firefighters—10,112; EMS—1,814) | USA | Mean 39.7 years | Occupation type: firefighters, EMSOccupational substance: WTC PM | Employment records | Met one of the following criteria:
| Medical records | Multivariate Poisson regression model; Multivariate Cox regression model | 3 |
D’Andrea and Reddy [43] | Frontiers in Public Health | 2018 | Switzerland | Prospective cohort | 44 | USA | Mean 43.1 years | Occupation type: oil spill clean-up workersOccupational substance: crude oil | Referral from legal representatives regarding oil spill clean-up activities | Prior CRS diagnosis | Medical records | Descriptive statistics | 3 |
Major study characteristics are study and citation number, journal of publication (journal), publication year (year), country, study type, the sample size of study (sample size), the country in which the study population originates (population country), age of study population (population age), occupational variables, method the study used for measuring their occupational variables (occupational measurement method), criteria for defining CRS (CRS criteria), the method which they used to diagnose or retrieve information about CRS (CRS diagnosis method), the main statistical methods used to assess the relationship between CRS and the occupational variables (statistical methods), and the level of evidence as defined by Oxford criteria (Oxford level of evidence). CRS: chronic rhinosinusitis; CT: computed tomography; EMS: emergency medical services; EPA: Environmental Protection Agency; EPOS: European Position Paper on Rhinosinusitis and Nasal Polyps; ESS: endoscopic sinus surgery; FDNY: Fire Department of New York; HMW: high molecular weight; ICAR: International Consensus Statement on Allergy and Rhinology; ICD: International Classification of Diseases; ISCO: International Standard Classification of Occupations; LMW: low molecular weight; NO2: nitrogen dioxide; PM: particulate matter; VGDFFiM: vapors, gases, dust, fumes, fibers, or mists; WTC: World Trade Center
Occupation types or occupation status & CRS
Five studies assessed the relationship between occupation status or type (i.e. employed vs. unemployed; legislator vs. forestry worker) and CRS [28, 29, 31, 34, 35].
Hoffmans et al. [29] stratified participants by the following employment statuses: employed, unemployed, self-employed, not working because of poor health, full-time house person, full-time student, retired, and others. Among the entire population (n = 8,347), the prevalence of CRS was 16%, but they did not find any significant association between occupation status and CRS.
Tai et al. [34] distinguished participant occupation by employment status and setting using three categories: indoor occupation, outdoor occupation, or unemployed. Similar to Hoffmans et al. [29], they did not find any association between occupational variables and CRS in their population (n = 26,335).
Gao et al. [28] examined healthcare and clearance-related jobs and found that individuals with clearance-related jobs had increased odds of developing CRS (OR 1.90 [1.09, 3.29]). Unfortunately, no definition or examples for clearance-related jobs were given. The prevalence of CRS in their entire cohort (n = 10,633) was 8%.
Thilsing et al. [35] examined CRS in blue-collar and white-collar jobs, as defined by the International Standard Classification of Occupations. The overall prevalence of CRS was 7.8% (n = 3,099). There was no significant association between blue-collar jobs and CRS prevalence compared to white-collar jobs. However, when stratified by sex, women in blue-collar jobs had a higher risk of CRS diagnosis (RR 1.64 [1.10, 2.43]), while men in blue-collar jobs had a lower risk of CRS diagnosis (RR 0.57 [0.42, 0.77]).
Koh et al. [31] were the most specific and detailed in their classification, specifying ten occupational categories (Table 1) at 1998, 2001, and 2005 time points. Significant associations between occupational categories and CRS varied by year and sex. Elementary occupations had an increased prevalence of CRS in males in 1998 (PR 1.68, [1.02, 2.77]) and 2001 (PR 3.07 [1.13, 8.32]) but not in 2005. Male plant or machine operators and assemblers had an increased prevalence of CRS in males in 2001 (PR 2.88 [1.13, 7.34]) and in 2005 (PR 1.76 [1.02, 3.05]). Unemployed males (M) and females (F) had an increased prevalence of CRS in 2005 only (M—1.81 [1.05, 3.14], F—2.07 [1.08, 3.96]). Lastly, male craft and related trade workers had an increased prevalence only in 2005 (PR 1.73 [1.03, 2.92]). Prevalence of CRS in the entire population by year and sex was 2.24% (M) and 1.81% (F) in 1998, 0.81% (M) and 0.58% (F) in 2001, and 2.35% (M) and 1.89% (F) in 2005. Consistent associations between occupation and CRS were not found in all years.
Occupationally-related compounds & CRS
Nine studies assessed the relationship between specific compounds that patients were exposed to due to their occupation and CRS [25–28, 30, 33, 35, 39, 41].
Two of these, Gao et al. [28] and Thilsing et al. [35] have previously been mentioned, as they also examined occupation type and CRS. Regarding occupationally related compounds, Gao et al. [28] examined the effect of poisonous gas, dust, damp or moldy environments, and carpets. Each of these exposures was associated with increased odds of CRS diagnosis (poisonous gas—OR 1.77 [1.13, 2.80]; dust —OR 2.21 [1.58, 3.01]; damp or moldy environment—OR 2.37 [1.48, 3.79]; carpet—OR 3.76 [2.23, 6.09]). Thilsing et al. [35] examined exposure to gases, fumes, dust, or smoke, and other agents. They found exposure to gases, fumes, dust, or smoke was associated with an increased relative risk of CRS diagnosis of 1.35 [1.01, 1.80]. They also noted an increased risk of CRS was associated with fish or shellfish exposure (RR 2.07 [1.41, 3.04]).
Clarhed et al. [25] published two studies two years apart (2018 and 2020) using the same population. They assessed the effect of a wide variety of specific compounds on CRS, which can be found in Table 1. In 2018, specific exposures associated with increased CRS prevalence included physically strenuous work (OR 1.4 [1.2, 1.7]), paper dust (OR 1.3 [1.1, 1.5]), metal dust (OR 1.3 [1.1, 1.6]), moisture/mildew/mold (OR 1.3 [1.1, 1.5]), cleaning agents (OR 1.2 [1.0, 1.3]), and animals (OR 1.2 [1.0, 1.5]) [26]. In 2020, they published a follow-up study that examined the same variables collected in 2018. The five-year cumulative CRS incidence rate for the entire population was 5.5%. Specific exposures that were associated with increased CRS prevalence included hair care products (OR 1.67 [1.09, 2.56]), superglue (OR 1.44 [1.06, 1.95]), strong acids (OR 1.42 [1.07, 1.89]), cooking fumes (OR 1.38 [1.07, 1.77]), and wood dust (OR 1.36 [1.02, 1.82]).
Velasquez et al. [39] examined exposures to vapors, gases, dust, fumes, fibers, or mists (VGDFFiM) and diesel fumes in a cohort of patients with CRS with Nasal Polyps (CRSwNP), CRS without Nasal Polyps (CRSsNP), and aspirin exacerbated respiratory disease (AERD). They further stratified the data based on CRS phenotype (CRSwNP and CRSsNP) and level of exposure based on a job exposure matrix. They found that 48.6% of CRSwNP had VGDFFiM exposures compared to 63.5% of CRSsNP patients (p = 0.03). Differences in prevalences of CRS phenotypes were not found with any level of diesel fume exposure. Lund-Mackay score was not significantly different between subgroups exposed to VGDFFiM and diesel fumes. However, CRS patients with any level of VGDFFiM or diesel fumes exposure had higher rates of ESS compared to nonexposed patients (VGDFFiM 34.5% vs. 20.6%, p = 0.0378; diesel fumes 36.8% vs. 25.8%, p = 0.0827). Additionally, CRS patients with any level of exposure to either compound had higher average steroid doses compared to non-exposed (VGDFFiM 1,095 mg vs. 644.1 mg, p = 0.015; diesel fumes 1,153.8 mg vs. 744.6 mg, p = 0.03) [39].
Nynäs et al. [33] examined those with mold/moisture damage exposure, with a resulting prevalence of CRS of 11% for their entire population (n = 99).
Veloso-Teles et al. [41] assessed the impact of dust exposure on CRS, specifically by comparing textile (dust-exposed) vs. retail workers (non-exposed controls). They found that the Lund-Kennedy score of those with CRS was significantly higher in textile workers (3.43) compared to retail workers (1.76) (p < 0.001).
Finally, Hox et al. [30] and Dietz de Loos et al. [27] gathered data on occupationally related compounds but labeled each as relevant or irrelevant based on expert opinion. Hox et al. [30] found a linear relationship between the number of ESS and relevant occupational exposures. As the number of ESS increased, the proportion of those with relevant occupational exposures increased (χ2 = 12.74, p < 0.001). Odds of reporting an occupational exposure were also higher when comparing people with two ESS vs. one ESS (OR 1.63, [1.06, 2.51]) and three or more ESS vs. one or two ESS (OR 1.97 [1.14, 3.42]). Dietz de Loos et al. [27] reported that subjects with one or more ESS had a statistically significant increase in relevant occupational exposures than those without ESS (χ2 = 6.30, p = 0.04). In univariate analysis, occupational exposure was significantly associated with having at least one ESS (OR 8.5 [1.1, 64.6]).
Military occupation & CRS
Two studies assessed the relationship between occupational exposures and CRS, specifically in the military population [36, 38]. Balali-Mood et al. [36] studied veterans who had been exposed to sulfur mustard. They found a CRS prevalence of 55% in their population (n = 43). Elam et al. [38] examined the impact of ambient fine PM (PM2.5), coarse PM (PM10), nitrogen dioxide (NO2), and ozone exposure in active-duty service members based on their duty station locations. Notably, those with CRS were exposed to significantly higher levels of PM2.5 and ozone compared to a matched control cohort being seen for cerumen impaction (p < 0.001, p = 0.17). Still, a 1-year mean increase in any of the four pollutants (by 5 units for PM2.5, PM10, and NO2 or one interquartile range for ozone) was associated with increased odds of CRS (PM2.5 OR 3.15 [1.09, 9.68]; PM10 OR 1.19 [0.65-2.16]; NO2 OR 1.22 [0.53, 2.82]; ozone OR 1.66 [0.73, 3.74]).
Disaster response occupations & CRS
Five studies assessed the relationship between occupational exposures due to unique disasters and CRS [32, 37, 40, 42, 43]. Cho et al. [37], Liu et al. [32], Weakley et al. [42], and Putman et al. [40] focused on first responders to the World Trade Center (WTC) 9/11/2001 disaster who were exposed to harmful levels of PM among the burning debris and rubble. D’Andrea and Reddy [43] focused on workers aiding in the clean-up of the Deepwater Horizon oil spill off the Gulf of Mexico who were exposed to crude oil.
Cho et al. [37] examined a cohort of WTC workers referred for a subspecialty pulmonary evaluation due to respiratory complaints. They reported an overall CRS prevalence of 42% and a ten-year cumulative incidence of approximately 50%, with 18% being categorized as more severe disease based on the need for surgical intervention. Among their patient cohort, the average time from WTC to CRS diagnosis was 39–40 months.
Liu et al. [32] described the incidence and relative rates of CRS in male firefighters, stratified by exposure level based on arrival time to the WTC scene. They reported an increase in CRS incidence rate of 2.68% per year for those with any level of exposure. The higher the exposure level, the higher the incidence, with those at the highest level having an incidence rate increase of 2.79% per year. Relative rates showed a similar trend, with the highest relative rate occurring when comparing high (arrival on the morning of 9/11/2001) and low exposure (arrival on any day between 9/13/2001 and 9/24/2001) levels (1.90 [1.56, 2.31]).
Weakley et al. [42] also studied male firefighters with CRS outcomes stratified by exposure level. Ten-year cumulative incidence rates of CRS diagnosis were directly associated with exposure level, with the highest exposure level having a CRS diagnosis rate of 23.5%. Relative rates were also directly associated with exposure level, with the highest relative rate being 1.98 [1.64, 2.39] between the high and low exposure groups. Additionally, the authors noted that the incidence of CRS diagnosis increased dramatically starting five years post-9/11 and peaked between seven and ten years later, however, no specific change point was identified.
Putman et al. [40] described the prevalence, incidence, and hazard ratios of CRS for firefighters and emergency medical service (EMS) workers, stratified by level of exposure based on arrival time, duration of work, and job task. Prevalence for firefighters and EMS were 23% and 9%, respectively. Incidence per 100 person-years for firefighters and EMS were 1.80 [1.72, 1.87] and 0.70 [0.62, 0.78], respectively. Both occupations had similar surgical rates for treatment (28% vs. 24%). Firefighters were more likely to be affected by CRS (HR 2.01 [1.63, 2.49]) than EMS. Compared to a one-month work duration, longer work durations were associated with an increased risk of CRS, with the highest hazard ratio of 1.47 [1.31, 1.65] at six months. Only a combination of digging and rescue work showed a significantly increased risk of CRS (HR 1.54 [1.23, 1.94]).
D’Andrea and Reddy [43] studied adverse health outcomes in workers who helped in the oil spill clean-up but presented limited data on CRS. The authors only provide a seven-year cumulative incidence rate of 91%.
Discussion
As the estimated global prevalence of CRS is 5–10% [1], it is critical to better understand predisposing preventable factors. Occupational exposures are an excellent target, as the relationship between occupational exposures and pulmonary disease is well recognized [9–11]. However, clear and specific evidence between occupational exposures and CRS is needed before implementing such strategies. We aimed to perform a scoping review to synthesize studies examining this relationship and summarize their evidence to better inform future research as a step toward identifying preventative measures. Our examination of 19 studies revealed a complex relationship between occupational exposures and CRS, influenced by several factors, including occupation types, specific compounds encountered, populations studied, and methodologies employed.
Study results must be interpreted only in the context of the populations examined in each specific study. Studies with more niche cohorts and smaller sample sizes reported higher rates of prevalence (42%, 55%, and 91%, respectively) [36, 37, 43]. Despite the small sample sizes, these results are still relevant and highlight the hazardous nature of the exposures in question and, thus, the danger of occupations such as military and disaster response. Studies with larger sample sizes but still unique populations are also not as generalizable but reinforce the fact that exposure to common ambient pollutants, especially PM, can contribute to CRS and emphasize that certain occupations, again usually military and disaster-response occupations like firefighters, may be at higher risk due to increased exposure quantity or duration [32, 38, 40, 42].
The most generalizable studies included those that examined large populations, but occupational details varied [25, 26, 28, 29, 31, 34, 35]. Of the studies with large sample sizes, four analyzed occupationally related compounds and found several compounds significantly associated with CRS diagnosis [25, 26, 28, 35]. These include a variety of exposures like gas, dust, damp environments, fumes, smoke, animals, cleaning agents, and strong acids that may be encountered more heavily in certain occupations like agriculture, construction, textile, and cleaning services. Additionally, Clarhed et al. [25] found an association between exposure to hair care products and cooking fumes and CRS, both of which can be occupationally related, as in barbers and chefs, and non-occupationally related, as encountered in everyday life. Other studies assessed occupation type and found associations with CRS, such as plant and machine operators [28, 31]. Lastly, some studies stratified their data by broad categories like employed, unemployed, blue collar, white collar, indoor job, and outdoor job [29, 34, 35]. None of the studies with broad employment categories found any significant associations with CRS diagnosis, most likely because the broad categories obscure any possible association, as the populations that fall into each category are too heterogeneous in their specific exposures (occupation type or compound encountered). So, despite their large sample sizes, their results are not clinically impactful when considering areas for intervention and prevention.
Four studies had relatively small sample sizes, and though they collected compound-specific data, compounds were lumped into larger categories (except for Nynäs et al. [33], who only examined moisture and mold exposure) [27, 29, 30]. These four studies found significant associations between compound exposure groups and CRS diagnosis, such as those exposed to vapors, gases, dust, or moisture. However, it is possible that only a few compounds within the larger group or a few occupations most heavily exposed to these compounds may be driving the association, or perhaps only the cumulative effect of all compounds drives the association. Given these possibilities, it is difficult to determine which occupations or work roles are specifically at risk, though some that certainly have exposure to such compounds include construction workers, textile workers, and mechanics. Additionally, two studies classified compounds as relevant or irrelevant based on expert physicians’ opinions [27, 30]. The lack of criteria for these decisions or a list of what was deemed relevant makes it difficult to draw or recommend interventions.
Beyond the general development of CRS, three studies assessed how occupational exposures relate to disease severity by examining objective findings, quality of life measures, the rate of ESS, and steroid doses [27, 30, 39]. Primary outcomes in two of these studies were the number of ESS performed on CRS patients, and they found that patients with more ESS were more likely to have reported relevant occupational exposure [27, 30]. This indicates that certain exposures may not only contribute to CRS development but also influence CRS disease and make it more difficult to treat. However, it is important to note that conclusions from these studies must be interpreted in the context of small sample sizes (n = 546, n = 364) and seemingly subjective determination of occupational exposure relevancy, as previously mentioned. Velasquez et al. [39] findings that any exposure to VGDFFiM, or diesel fumes resulted in a higher rate of ESS and steroid dosage support a similar conclusion. Interestingly, however, Velasquez et al. [39] also noted no significant differences in Lund-Mackay scores of patients with differing levels of exposure. This finding emphasizes that the relationship between exposure and severity of CRS may vary depending on how severity is specifically defined across studies.
Another limitation of the current literature includes the diagnostic criteria of CRS. These varied in using objective findings, subjective symptoms, or both in the diagnosis. The diagnostic criteria including both symptoms and objective findings is essential, as it embodies both the pathological changes and their impact on human functioning. Using one or the other does not fully characterize the CRS disease burden. Furthermore, common methods of abstracting data on CRS amongst studies differed, including self-reports via survey and medical record review. Self-reporting is of concern as there is a high risk of recall and response bias. Use of medical records may also be unreliable if there is no information on the type of physician giving the diagnosis, as some may not follow appropriate criteria.
In summary, moderate evidence supports an association between occupational exposures and CRS, with the strongest relationships being seen in military and disaster-response occupations. This data is corroborated by basic science studies that have hypothesized possible mechanisms by which such exposures can upregulate inflammatory pathways and impair mucociliary clearance, both of which underly the pathogenesis of CRS. Multiple in vitro studies have demonstrated that PM stimulates nasal epithelial cells, macrophages, and fibroblasts to release pro-inflammatory cytokines [44, 45]. One proposed mechanism by which this occurs is through the upregulated expression and release of specific microRNA (miRNA) in response to PM that promotes the M1 polarization of macrophages, propagating inflammation [16]. Similarly, exposure to diesel exhaust particles and Asian sand dust results in a similar release of pro-inflammatory cytokines from nasal fibroblasts, such as IL-6 and IL-8, although via different mechanisms [44, 46]. Asian sand dust has also been shown to work synergistically with microbes like Aspergillus fumigatus to increase microbial growth and biofilm formation, further stimulating an inflammatory response [47]. PM has also been shown to negatively impact mucociliary clearance. For example, exposure to PM2.5 and hog barn dust increases mucin-protein production, leading to thickened and viscous mucous and impaired ciliary action [48, 49]. Over time, chronic leads to a blunting of the mucociliary response [50]. Furthermore, PM, especially less than 2.5 microns, can disrupt the nasal epithelial barrier through oxidative stress, leading to the downregulation of tight junction proteins [15].
Unfortunately, the heterogeneity of populations, methodologies, and independent and dependent variables limits the ability to draw specific conclusions about the relationship between certain occupational exposures and the development of CRS. To better characterize these relationships, large studies with diverse populations and a clear definition of CRS that includes both objective evidence of disease and detailed exposures are needed. Occupational variables should be as inclusive and specific as possible, ideally including occupation type and exogenous compounds. Compound exposure may be inferred from occupation type, but methods would ideally be based on reliable databases or matrices relevant to the population location.
Conclusions
In conclusion, the relationship between occupational exposures and CRS is multifaceted and complex. While evidence supports a general association between occupational exposures and CRS, drawing specific conclusions remains challenging due to heterogeneity across studies. Moving forward, future research endeavors must address limitations such as small sample sizes, overly broad occupational categories, differing CRS diagnostic criteria, and different methods of gathering data. By adopting rigorous methodologies and standardized criteria, researchers can enhance our understanding of the relationship between occupational exposures and CRS diagnosis, ultimately informing preventative measures and interventions to mitigate the burden of CRS on the individual and population levels.
Abbreviations
CRS: | chronic rhinosinusitis |
CRSsNP: | chronic rhinosinusitis without nasal polyps |
CRSwNP: | chronic rhinosinusitis with nasal polyps |
EMS: | emergency medical service |
EPOS: | European Position Paper on Rhinosinusitis and Nasal Polyps |
ESS: | endoscopic sinus surgery |
NO2: | nitrogen dioxide |
PM: | particulate matter |
VGDFFiM: | vapors, gases, dust, fumes, fibers, or mists |
WTC: | World Trade Center |
Supplementary materials
The supplementary material for this article is available at: https://www.explorationpub.com/uploads/Article/file/100946_sup_1.pdf.
Declarations
Author contributions
ASM, CPW, and EHB: Conceptualization, Investigation, Writing—original draft, Writing—review & editing. CKC, BDT, CSE, BAS, and AJK: Conceptualization, Writing—original draft, Writing—review & editing.
Conflicts of interest
The authors declare that they have no conflicts of interest.
Ethical approval
Not applicable.
Consent to participate
Not applicable.
Consent to publication
Not applicable.
Availability of data and materials
The primary data for this review were sourced online from databases listed in the methods. Referenced articles are also accessible on the databases.
Funding
Research in this publication was supported by the National Institute on Deafness and Other Communication Disorders branch of the National Institute of Health under award number [T32 DC005360] for authors E.H.B. and C.P.W. The content is solely the responsibility of the authors and does not necessarily represent the official views of the National Institutes of Health. The funder had no role in study design, data collection and analysis, decision to publish, or preparation of the manuscript.
Copyright
© The Author(s) 2024.