Abstract
In recent decades, atopic diseases, such as atopic dermatitis (AD), allergic asthma (AA), allergic rhinitis (AR), and food allergy (FA) have been estimated rapidly increasing in prevalence. These diseases are characterized by the presence of specific immunoglobulin E (sIgE) and often relate to each other and develop in sequence (the so-called “atopic march”). AD may be the first early manifestation in infants followed by FA often within the first year of life. Moreover, AD is a risk factor for developing sensitization to airborne allergens later in life that can cause clinical manifestations of AA and AR. According to the dual-allergen exposure hypothesis, allergic sensitization to food allergens is promoted through cutaneous exposure, rather than the oral route. Moreover, there is evidence that exposure to food allergens, in particular peanuts, in the airway would also lead to food sensitization. The most frequent route of sensitization for inhalant allergens is still debated. Of note, a recent case report supports the development of sensitization to cat dander through a cat bite. Our review aims to provide an overview of current knowledge and unmet needs in the pathophysiology of respiratory and FAs.
Keywords
Allergy, mechanisms, skin barrier, airwayIntroduction
The discovery of allergy dates back to 450 BC, when Greek doctors, using the methods of Hippocrates, identified a disease defined as “atopy” [ἄλλος (állos, “other”) + ἔργον (érgon, “work, activity”), i.e. “different or strange reaction”] for the definition caused by commonly harmless substances [1, 2]. Atopy was considered rare but, already in the Roman period of 30–40 BC, it appeared to be quite widespread. In particular, in this historical period, the first “diagnoses” of allergy were reported by Svetonius referring to eye swelling which prevented vision caused by contact with horses [2]. However, most substances with allergenic activity are not directly visible, and we had to wait until the years 1700–1800 to discover the etiologies of allergy, such as pollens and dust mites. Bostock discovered pollen allergy, which was defined as hay fever in 1819 [3]; in 1915, Voorhorst discovered the role of dust mites, and specially of Dermatophagoides species as triggering allergen [4]. Over time more and more allergenic sources have been identified, varying from biologic sources with very complex compositions (e.g., pollens, house dust mites, and foods), to single molecules such as chemicals (occupational allergy) or drugs (drug allergy) [5–7]. It is reasonable to imagine that new causes of allergy may be discovered in the future.
Furthermore, a dramatic rise in the prevalence of allergy has been reported in the last decades, as well as the development of increasingly effective treatments and management for allergy based on a better knowledge of underlying immunopathomechanisms [8].
In particular, intact skin and mucosal barriers play a key role in maintaining tissue homeostasis which protects tissues from harmful agents, whereas a defective epithelial barrier may facilitate allergic and autoimmune conditions [9].
This review focuses on the recent insights in the context of the pathomechanisms underlying allergy to airborne and food allergenic sources.
From the hygiene hypothesis to the skin-barrier hypothesis
The development of allergic diseases results from the interaction between genetic and environmental mechanisms. The increase in these diseases has occurred in relatively too short a time to be justified by genetic mutations for which considerable importance has been given to environmental factors linked to the modern lifestyle including changing dietary patterns, changing intestinal commensal bacteria, and vehicular pollution [10]. Studies suggest that environmental factors can produce epigenetic changes in gene expression and disease risk that may be potentially heritable across generations.
Already in 1989, Strachan [11] found an inverse relationship between the development of hay fever and the number of the family units and the birth-order hypothesizing that the increase in the prevalence of hay fever was to be attributed to a reduced number of infections in childhood. This concept has subsequently undergone some evolutions by attributing the increase in allergies not to the reduction of infections in the early stages of life but rather to a reduction in the colonization of the organism by commensal microorganisms [12]. Allergic diseases such as atopic dermatitis (AD), allergic asthma (AA), and allergic rhinitis (AR) present an alteration of the epithelial structure of the respective affected organs which causes a dysfunction of the barrier effect [13]. This dysfunction can favor the passage of external agents such as microorganisms and allergens responsible for the release of alarmin cytokines, such as thymic stromal lymphopoietin (TSLP), IL-25, and IL-33, with the development of a Th2-type immunological reaction [13]. Allergic diseases begin to appear frequently early in life and longitudinal studies have shown that they often manifest themselves in progression. The classic sequence (the so-called “atopic march”) from AD and food allergy (FA) to AA and AR (Figure 1) [14, 15], has not been recently highlighted in a large population of children [16]. AD generally represented the first manifestation of the atopic march although it does not necessarily evolve in following atopic diseases. Furthermore, only a few children who presented AD early in life developed asthma and AR until adolescence, and among those who presented all three atopic diseases, only a few developed them according to sequences known as the atopic march [16]. Cutaneous food allergen exposure in children with AD may promote immunoglobulin E (IgE)-mediated sensitization. Furthermore, Th2 memory cells, IgE, TSLP, IL-33, and IL-25 can reach the digestive and respiratory tracts through the blood circulation and lead up to the development of FA, AA, and AR if they encounter the allergen again (Figure 2) [15]. AD is very common in industrialized countries where it occurs in approximately 15–30% of children and 2–10% of adults [17–20]. Epidemiological studies have found that in 45% of affected children, AD appears in the first 6 months of age, in 60% within the first year, and up to 90% before the age of 5 years [14, 17, 21]. Tsakok and collaborators [22] in a systematic review reported that food sensitization was up to 6 times more frequent in patients with AD than those without AD. The early onset and severity of AD increase the risk of developing food sensitization, AA, and AR. Dharmage et al. [23] demonstrated that children with AD in the first two years of life had a significantly increased risk of developing AA and AR during 6–7 years. Moreover, many studies found that children with increased severity of AD had an increased risk of developing FA, AA, and AR [22, 24, 25]. Overall, current evidence supports a multimorbidity framework for atopic diseases, without a specific sequential atopic march progression [16].
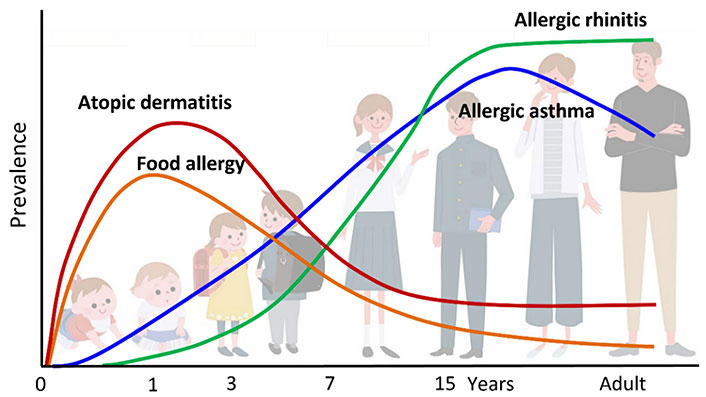
Classic atopic march. Atopic dermatitis (AD) generally occurs first, followed by immunoglobulin E (IgE)-mediated food allergy (FA), allergic asthma (AA), and allergic rhinitis (AR)
Note. Reprinted from “Current Insights into Atopic March” by Tsuge M, Ikeda M, Matsumoto N, Yorifuji T, Tsukahara H. Children (Basel). 2021;8:1067 (https://doi.org/10.3390/children8111067). CC BY.
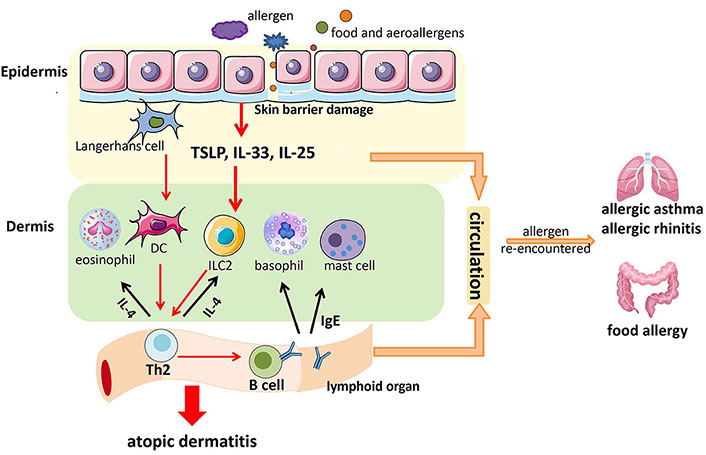
A possible mechanism through which food sensitization favors the development of food allergy (FA), allergic asthma (AA), and allergic rhinitis (AR). TSLP: thymic stromal lymphopoietin; DC: dendritic cell; ILC2: type 2 innate lymphoid cells; IgE: immunoglobulin E
Note. Reprinted from “Research Progress in Atopic March” by Yang L, Fu J, Zhou Y. Front Immunol. 2020;11:1907 (https://doi.org/10.3389/fimmu.2020.01907). CC BY.
Routes of sensitization to food and airborne allergens
Sensitization to foods can occur not only through the gastrointestinal tract and oral cavity but also by different routes, such as the skin or the airways [26]. Sampson et al. [26] stated that even though oral tolerance is a usual response to swallowed antigens, a breakdown in this process apparently occurred in recent decades, resulting in a growing prevalence of sensitization to food allergens.
On the other hand, several studies demonstrated that breastmilk contains airborne allergens as well as food allergens derived from the maternal diet [27]. Inhalant allergens from Dermatophagoides pteronyssinus 1 (Der p 1) and Blomia tropicalis 5 (Blo t 5) have been detected in human milk [27]. Baïz and coworkers [28] demonstrated that children breast-fed by mothers with high Der p 1 levels had an increased risk of allergic sensitization and asthma and AR at five years of age. The mechanisms involved in natural history and therapeutically induced tolerance are thought to include suppression of Th2 cells, generation of T regulatory cells (Tregs), reduced production of IgE by B cells, augmented IgA and IgG4 production by B cells, defeat of effector T-cell migration to tissues, induction of IL-10-producing dendritic cells (DCs), and downfall of basophil, eosinophil, and mast cell activation [29]. Albeit with some variations, the same mechanisms which were reported to be accountable for the development of immune tolerance to allergens through different routes, such as Hymenoptera venom injected into the skin and pollens or dust mites inhaled into the respiratory tracts, are likely to be applicable in oral tolerance.
Sensitization to food allergens
From tolerance to sensitization in the gastrointestinal tract
The gastrointestinal tract is continuously exposed to a wide range of non-self-antigens including both commensal bacteria (microbiota) and food proteins [30]. Under physiological conditions, ingested foods evoke an immunological response of tolerance which is characterized by an active suppression of cellular and humoral immune responses towards an antigen with which it had already come into contact via the oral route [31]. The immunological mechanisms leading to the development of food tolerance are not fully understood and are mainly based on experimental mouse models. In principle, oral tolerance to food antigens is based on the effective production of Tregs within the intestinal mucosa [26].
After food ingestion, most of the food proteins are degraded into small peptides which, together with the remaining intact proteins, pass the intestinal barrier and are then presented to the immune cells [26]. The ways of overcoming the epithelial barrier include paracellular diffusion, endocytosis through microfold cells (M cells) and goblet cells, and the intervention of DCs which extend their dendrites into the intestinal lumen capturing food antigens [32]. Within the mucosa, food antigens are captured by antigen-presenting cells (APCs) which migrate to the intestinal draining mesenteric lymph nodes (mLNs; gut-draining mLNs) where cell differentiation occurs of naive T cells into Tregs [30]. Although Tregs specific to food allergens develop and localize in the intestine, they can also be found in the circulation where they maintain systemic tolerance [30].
The major mechanisms by which Tregs lead to tolerance to food antigens include at least the following: 1) encouraging B cells to produce non-inflammatory IgA-type responses, 2) suppressing Th2-type responses, and 3) inhibiting pro-inflammation, such as mast cells, basophils, and eosinophils leading to a state of non-responsiveness to ingested antigens or oral tolerance [30].
Whether food tolerance does not develop or if an existing tolerance is broken down, sensitization to food antigens can develop, which is characterized by a Th2 response with the production of specific IgE (sIgE) [33]. The mechanisms by which some subjects develop allergic sensitization towards some foods while the majority of subjects develop and maintain tolerance are not fully understood, but there is evidence that some factors can alter at least in part the normal balance existing between tolerance and allergy [33].
These adjuvant factors include gut microbiota status, intestinal pathogen infections, dietary factors, dose and timing of exposure to food allergens, and route of contact and penetration of the allergens [33]. For years it has been believed that FA develops exclusively in the intestine in children through breast milk or following an early introduction into the diet [34]. Du Toit et al. [35] demonstrated that in a large population of children at high risk of developing peanut allergy, the early introduction of peanuts into the diet was accompanied by a significantly lower prevalence of peanut allergy up to the age of 5 years. Furthermore, it has been highlighted that the preventive effect of FA with the early introduction of the food is allergen specific. In the Learning Early About Peanut Allergy (LEAP) study, it was found that the early introduction of peanuts had not led to the prevention of allergy to nuts or sesame seeds [36].
Transcutaneous food sensitization
Numerous studies have highlighted the growing importance of allergic sensitization through a dysfunction of the skin barrier. The skin, unlike the other epithelial surfaces, has a structure composed of two barriers: the stratum corneum (SC), which is exclusive to the skin, and the tight junctions (TJs) also present in the other epithelial surfaces [37]. Both the SC and the TJs moderate on the one hand the entry and reaction towards external agents such as microorganisms, allergenic proteins, irritants, and toxins, and on the other hand they prevent the outward leakage of water through the skin [transepidermal water loss (TEWL)]. Filaggrin (FLG), a barrier protein, plays an important role in the normal structural composition of the SC [15]. FLG loss-of function mutations have been frequently associated with AD which is characterized by barrier effect dysfunction and subsequent inflammatory lesions caused by stimulation of Th2 cells [38]. On the other hand, FLG developmental genetic mutations have not been detected in many patients with AD and over 50% of subjects with the mutation do not develop AD [39]. FLG genetic mutations increase the risk of severe AD and FA in individuals with AD [40, 41]. A strong association was found between AD, sensitization to food allergens, and FA [15].
The role of the FLG genetic defect in the development of FA was demonstrated by Brough et al. [42] in a population of almost 1,200 children. The authors measured the content of peanut antigens in house dust during the first year of life of the children and evaluated the development of sensitization by prick test and immuno-Cap towards Ara h 1, 2, and 3 at the age of 8 and 11 years. The conclusions were that environmental exposure to peanuts early in life is associated with an increased risk of sensitization to peanuts in children carrying the FLG gene mutation [42].
The prevalence of FA in patients with AD varies according to the time of onset and the severity of AD. In a population of more than 600 children, Hill and Hosking [43] demonstrated that as AD severity increased, adverse reactions to food increased. In the ALSoPAC study, the application of emollient creams containing peanut oil to the inflamed skin of children was found to be an independent risk factor for the development of allergic sensitization to peanuts [24].
Airway sensitization to food allergens
The dual allergen exposure hypothesis proposed by Lack et al. [24] suggests that food sensitization can occur through the impaired skin barrier. Kulis et al. [44] proposed that exposure to food allergens, such as peanuts, in the airway is a possible route of sensitization. Peanut protein has been found not only in the home environment but also in school cafeterias and classrooms, where children spend a lot of their time [45]. Inhalational exposure to peanut extract with house dust resulted in the production of peanut-sIgE and anaphylaxis in consequence of the peanut challenge [46]. The authors reported that exposure to peanut extract or dust alone did not cause peanut sensitization.
Sensitization to inhalant allergens
Custovic [47] in a review reports that despite contact with inhalant allergens (e.g., dust mites, epidermal derivatives of pets, and pollens), which occurs under physiological conditions at the level of the mucous membranes of the respiratory tract through inhalation of the air environment, it is unknown which is the most relevant sensitization pathway for inhalant allergens. The epithelium of the respiratory tract represents the first defense barrier against inhaled particles—such as allergens, microorganisms, and pollutants, preventing their diffusion within the respiratory mucosa. Most of the particles inhaled into the respiratory tract are trapped in the mucus layer covering the epithelium and then eliminated by mucociliary clearance. In addition to exercising the physical barrier function, the cells of the respiratory epithelium have a key role in the immunological, inflammatory, and repair responses following contact with environmental factors present in the inhaled air [48]. In longitudinal studies that have studied the temporal evolution of allergic diseases, it has been found that they tend to manifest themselves with a progressive sequence defined as atopic march. In this natural evolution, the first manifestation is represented by AD followed by the development of FA and subsequently of AA and AR. The dysfunction of the skin barrier present in AD favors the penetration of allergens and causes Th2-mediated sensitization and inflammation in some children. Mutation of the FLG gene is reported as the major risk factor for the development of AD. Although there is a clear relationship between AD and sensitization to food allergens by transcutaneous absorption, the relationship to inhalant allergens is controversial [47, 49]. Wärnberg Gerdin et al. [50] demonstrated that in a population of nearly 2,000 infants with skin changes such as eczema, dry skin on the cheeks and/or extensors, and a high TEWL at 3 months of age, development of sensitization to food allergens was seen at the age of 6 months in 8.9% while sensitization to inhalants occurred only in 1.9%. Chiu and collaborators [51] studied a cohort of children from the Prediction of Allergies in Taiwanese Children (PATCH) study with the aim of detecting the development of sensitization to the most common food and inhalant allergens from 0 to 4 years. The authors found that sensitization to food allergens began before inhalant allergens and increased markedly from the age of 6 months reaching the peak at 1.5 years of age. In contrast, the prevalence of sensitization to inhalant allergens was only 5% at 2 years but increased markedly after 2 years of age [51]. Miwa and collaborators [52] demonstrated that FLG is also expressed in the human nasal epithelium of the inferior turbinate, both in the stratified squamous epithelium in the anterior portion, and in the pseudostratified ciliate epithelium in the posterior portion, as well as in the maxillary sinus mucosa. Moreover, bacterial and viral infections can modify FLG gene expression in human nasal mucosa. We can hypothesize that, particularly in children where upper respiratory tract infections are more frequent, these may favor the development of sensitization to inhalant allergens by increasing the permeability of the nasal mucosa epithelium in association with a Th2-type immune response. Studies have revealed the presence of airway epithelial dysfunction in both asthma and upper respiratory tract disease due to disturbances in TJ formation [53]. On the other hand, it is also known that some environmental allergens, such as Der p and various pollens are endowed with cysteine and serine pro-teases which are able to break the bonds of TJs [54, 55]. Similarly, molds of the genus Aspergillus and Penicillium have serine protease activity. Moreover, the role of proteolytic activity has not been demonstrated for human disease [54, 56]. In addition to the direct effect of inhalant allergens, allergic sensitization can lead to epithelial barrier dysfunction as a consequence of asthma-associated airway inflammation mediated by a Th2-type response [56]. This alteration can be caused by the intervention of both Th2 cells and type 2 innate lymphoid cells (ILC2) through the secretion of IL-13 which, in addition, causes other features of the respiratory epithelium in asthma, such as mucus production [57]. Furthermore, the destruction of barrier TJs can be induced by environmental pollutants such as particulate matter 2.5 and cigarette smoke [58].
New approaches for the diagnosis of allergy
The development of new insights into the pathomechanisms of allergies, and in particular the role of epithelial barriers, has stimulated new research fields. As we reported above, epithelial barriers represent the first line of defense against foreign substances, preventing the entry of potentially harmful substances through the epithelium. In the case of epithelial barrier dysfunction, allergens can penetrate through the epithelium followed by the release of cytokines (such as TSLP, IL-33, and IL-25) that cause type 2 immune responses. Recently, the first report was published on sensitization to cat allergen through cat bite in a child [59]. In this case report, sIgE assays showed positive results for cat extracts and rFel d 1. Identifying a new route of allergic sensitization to cat dander after decades of studies may appear unlikely. However, it must be considered that asking for information on possible cat bites is not included in the anamnesis. Previously, Maeda and Akiyama [60] described a case of anaphylaxis after a cat bite in a 42-year-old woman already sensitized to cat allergen. These clinical reports consolidate the assumption that a cat bite could contain enough allergen to both trigger symptoms and develop possible sensitization. The intestinal epithelium has an important barrier function as on the one hand, it has to defend against microorganisms and toxins and on the other it has to exchange nutrients, water, and electrolytes [61]. Ogulur and collaborators [62] investigated if professional and household dishwashers and rinse aid agents may have an effect on gut barrier dysfunction. The authors showed no toxic effects after exposure to professional dishwasher detergent at all dilutions down to 1:250. In contrast, rinse aids caused cell death and impairment of epithelial barrier epithelium by destroying the TJs [62]. Baur and collaborators [63] demonstrated that exposure to triclosan on reconstructed human epidermis modifies keratinocyte function and increases skin barrier permeability.
A recent study showed that low levels of zinc in erythrocytes are associated with the development of allergic proctocolitis in children [64]. Zinc is an important trace element in the early stages of life both for the progress of the barrier function of the epithelia and of the immune system [65]. Previous studies had found a relationship between low zinc values and allergic diseases in children, such as AD and asthma, but the relationship with allergic proctocolitis had not been reported [66, 67]. Gunaydin et al. [64] found in a population of children with a mean age of (7.1 ± 2.9) months affected by allergic proctocolitis, intracellular zinc values were significantly lower than those in a control group of the same number and mean age.
Microbiome
Many studies have suggested that the increase in allergic diseases in recent decades is due to an alteration of the intestinal microbiota resulting from better hygienic and sanitary conditions, more frequent cesarean sections and use of antibiotics, and a diet low in fiber and high in fat and sugar. Moreover, Roduit et al. [68] demonstrated that strategies to increase short-chain fatty acid (SCFA) levels, particularly butyrate and propionate, in the first year of life are associated with a lower risk of developing FA, asthma, and AR between 3 and 6 years of life. These data suggest that prebiotics and postbiotics in the first year of life may help prevent the development of allergic diseases [69].
Conclusions
Understanding how sensitizations to food and inhalant allergens occur is an important prerequisite in order to be able to implement prevention strategies [70].
Skin barrier dysfunction in AD is a risk factor for developing FA and a possible onset of the atopic march. More data are also needed to better understand the role of microbiome and nutrition in order to provide specific and targeted preventive and therapeutic measures also in this context whether the rational and evidence will be supported. In the near future, new immunological and cohort studies are needed to better understand the pathomechanism of allergic diseases and implement proper preventive and therapeutic strategies at a population and individual level.
Abbreviations
AA: | allergic asthma |
AD: | atopic dermatitis |
AR: | allergic rhinitis |
FA: | food allergy |
FLG: | filaggrin |
IgE: | immunoglobulin E |
SC: | stratum corneum |
sIgE: | specific immunoglobulin E |
TJs: | tight junctions |
Treg: | T regulatory cell |
TSLP: | thymic stromal lymphopoietin |
Declarations
Acknowledgments
The authors thank Stanley Norman for the English revision.
Author contributions
GL, CI, and SA: Writing—original draft, Writing—review & editing. All authors have read and agreed to the final version of the manuscript.
Conflicts of interest
The authors declare that they have no conflicts of interest related to this manuscript.
Ethical approval
Not applicable.
Consent to participate
Not applicable.
Consent to publication
Not applicable.
Availability of data and materials
Not applicable.
Funding
Not applicable.
Copyright
© The Author(s) 2024.