Abstract
Aim:
The purpose of this study was to digitally quantify the consistency and variation of printed resin crown patterns produced by different 3D printers, aiming to evaluate their clinical relevance and accuracy. This was accomplished by evaluating patterns manufactured using three different 3D printers and resin materials, assessing their fit and adaptation through digital scanning and analysis. The objective was to determine the internal and marginal variations of printed crown resin patterns and to identify the printer and material combinations that produce the most consistent and clinically acceptable results.
Methods:
A prefabricated typodont tooth was prepared for a crown and scanned using an intraoral scanner. From this scan, a crown was designed and the resulting STL file was exported. This 3D file was manufactured using resins indicated for castable patterns on 3 different printers: FotoDent® Cast with the Carbon M2 printer, Form 3 Castable with the Form 2 printer, and Siraya Tech Cast with the ELEGOO Mars 2 Pro 3D printer. Finally, the crown resin patterns were scanned using an intraoral digital scanner (3Shape TRIOS 3) scanner, and crown adaptation was digitally quantified in GeoMagic software. Clinically relevant significance was determined (marginal gap < 50 µm) between resin patterns printed on different printers.
Results:
Form 3 Castable/Form 2 printer had the lowest mean internal variation at all measurement areas, 21.07 μm at the occlusal, 104.23 μm at the margin, and 37.98 μm at the axial. FotoDent® Cast/Carbon M2 had the largest marginal variation while Siraya Tech Cast/ELEGOO Mars 2 Pro had the largest occlusal variation. Within each material, the largest internal variation was at the marginal area while the lowest value was in the occlusal area.
Conclusions:
All printed crowns displayed clinically acceptable ranges, but there was a statistically significant difference in the fit between all printers.
Keywords
3D printers, CAD-CAM, cast patterns, dental crown, marginal gap, resinIntroduction
The advent of digital workflows within dentistry has brought efficiency and predictability in all areas, particularly in the fabrication of full-coverage restorations. Traditional methods, often labor-intensive and prone to variability, are being replaced with digital approaches. This is demonstrated with the direct milling of restorations using monolithic materials, such as lithium disilicate or zirconia. Historically, computer aided design-computer aided manufacturing (CAD-CAM) workflows in dentistry primarily involved subtractive manufacturing (SM) techniques. As materials develop, additive manufacturing (AM) has begun to take over [1]. Typically, this is demonstrated by the direct printing of an object. Alternatively, indirect methods of fabrication can be utilized with AM by creating a pattern to be used as a mold [2]. This mold can then be utilized to create the product. Applied to dentistry, indirect AM methods may be used to produce crown patterns for pressed ceramic or cast metal restorations or copings [3].
To date, the most common method of AM resins in dentistry is selectively curing of liquid resin through targeted light-activated polymerization. While many types of resin printers exist, the steps of each can be defined as designing, printing, and post-processing [4]. The designing step includes both the design of the restoration and the nesting step to prepare for printing. When the product is printed, it is not fully polymerized, in order to finalize the product, it must go through a series of post-processing steps. While these vary between machines and resins, they generally include a cleaning step to remove the residual resin and support structure, and an additional exposure to ultraviolet (UV) light to more completely cure the printed resin [4].
Regardless of fabrication technique, a well-adapted margin is critical to the success of the restoration. Open or unsealed margins may allow for plaque accumulation, ingress of bacteria, and potential recurrent caries. While the number often varies, McLean and von Fraunhofer [5] are often cited, who stated that the marginal gap should be measured at 120 μm or less to be considered acceptable. The marginal gap is defined as the vertical space between the finish line of the restoration and the finish line of the tooth [6]. As the AM process varies from previous crown fabrication techniques, it is important to ensure that crowns fabricated with 3D printing meet clinical standards.
While there is limited evidence regarding additively manufactured full-coverage restorations, the current literature is encouraging. For instance, one study showcased the superior adaptation achieved through 3D printing compared to milling, highlighting its potential in the fabrication of dental restorations [7]. Other studies had similar conclusions, determining that printed resin crowns showed improved marginal fit [8], dimensional accuracy [9], and intaglio surface trueness [10] when compared to milled crowns. Further studies found AM crowns to be comparable in fit to SM, including one manuscript that underscored the comparable outcomes between 3D printing and both milling and conventional analog techniques [11]. Additionally, recent research by Khaledi et al. [12] evaluated various CAD-CAM techniques for fabricating crown copings, including 3D resin printers, milling machines, and wax printers, with results for all methods found clinically acceptable. This is in line with findings from Ahlholm et al. [13], concluding that the printed patterns in their study had improved or similar fit to milled patterns. A contrasting conclusion from another study found that milled crowns had better-adapted margins to a statistically significant level, but both types of fabrication resulted in samples that had clinically acceptable margins (< 120 μm) [14]. Regarding indirect AM fabrication, one study created wax crown patterns via conventional techniques, as well as AM and SM, which were then conventionally invested and cast with dental gold. Their findings indicated that their CAD-CAM fabricated patterns resulted in a better-adapted restoration, with AM crowns showing the best adaptation [15].
Regarding achieving this accuracy of a fit and marginal gap, there is a growing evidence base on how the printing steps may play a role. Studies evaluated how the print angle at the nesting stage (design) plays a role. While the results of the best angle are somewhat conflicting, it appears that the nesting angle does impact the accuracy of the print and subsequent fit of the restoration. For single crowns, one article noted that the placement angle did not appear to affect the accuracy [16], while others agree that nesting at 90° is the least accurate [17, 18]. A 2024 review paper [19] evaluated the effects of post-processing protocols on the accuracy of printing resin crowns. The results indicated that there was an effect on the accuracy of fit when wash times were modified.
Evidence is rapidly developing, but still, there are many areas that are unexplored. The specific aim of this project was to analyze the consistency and variation of internal fit for printed crown resin patterns manufactured using different 3D printers. The results from this study may influence a clinician or technician’s choice in 3D printer due to the expected quality of printed patterns. The null hypothesis states that internal fit variation of printed crown patterns does not vary between printers or resins.
Materials and methods
A maxillary second molar typodont tooth was prepared following parameters for a cast gold crown [20] (Figure 1A) and was then scanned using an intraoral scanner following the clinical protocol (3Shape TRIOS 3, Copenhagen, Denmark). A crown was digitally designed to fit the scanned preparation using dental design software (3Shape Dental System, Copenhagen, Denmark) (Figure 1B–D).
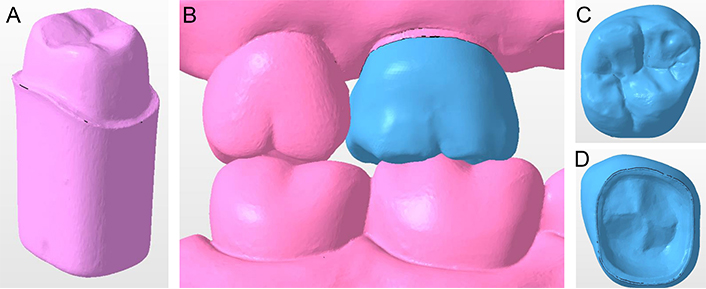
Designing the test crown. (A) A maxillary second molar typodont tooth prepared for a cast gold crown; (B) a crown was designed for the typodont preparation; (C) cameo surface of designed crown; (D) intaglio surface of designed crown
Note. Figure 1B adapted with permission from “A design file for 3D printed crowns made by Dr. Clark’s students” by Wendy Clark. c2024 (https://www.3dnatives.com/en/working3d-prosthodontist-3d-printing-220220244/). © 3Dnatives.
This design file was exported as a standard tesselation language (STL) and imported to each printer’s recommended nesting software, with the height parameter set to 50 μm (PreForm 3.33.1, Formlabs, Somerville, USA; CHITUBOX Basic 2.0, CBD-Tech, Shenzhen, China; Carbon 1.41 (Software), Carbon, Redwood City, USA), as was the manufacturer’s recommended setting for each of the resin/printer combinations. Formlabs and Carbon have pre-loaded settings for these resins in their software, and these were not altered. The recommended profile settings for the Siraya Tech Cast/ELEGOO Mars 2 Pro were imported into the CHITUBOX software (Table 1). The model was oriented with marginal surfaces protected and nested at an upward angle, as recommended in previous literature [17] (Figure 2A).
Print settings for Siraya Tech Cast/ELEGOO Mars 2 Pro
Parameter | Settings |
---|---|
Layer height | 50 μm |
Exposure | 15 s |
Number of initial layers | 5 |
Exposure for initial layers | 65 s |
Room temperature | 20–25°C |
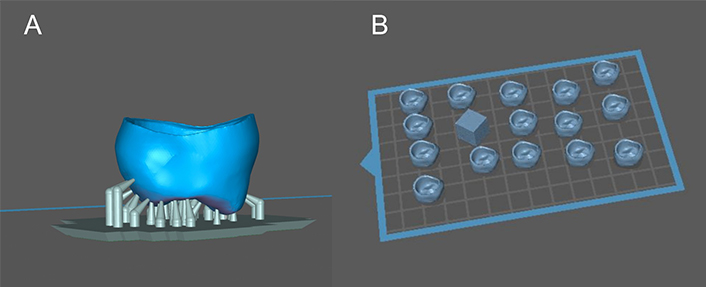
Crown patterns prepared for printing. (A) A crown nested in the printer’s recommended software (CHITUBOX, CBD-Tech); (B) the nested crown was duplicated for a total of 15 crowns, alongside 1 calibration cube
Light supports were then generated, located on the occlusal table and on the axial wall as needed. This nested crown was duplicated fifteen times on each printer’s build plate. A 1-cm reference cube was designed in open-source software (Tinkercad 2023, Autodesk, San Francisco, USA), and placed along with the crowns on each build plate (Figure 2B). Once nested, the print files were generated and sent to each printer. The crowns were fabricated utilizing three printers with different technology: (1) DLS (M2, Carbon, Redwood City, USA); (2) MSLA (Mars 2 Pro, ELEGOO, Shenzhen, China); (3) SLA (Form 2, Formlabs, Somerville, USA) printers (Figure 3). The resin materials were selected based on those that could be cast for a gold crown (Table 2). Fifteen crowns and one reference cube were printed on each unit, for a total of 45 samples and three cubes. The 1:1:1 cube was utilized as a reference for the crowns during data analysis and to ensure calibration of each printer.
Studied materials composition and machinery
Materials | Manufacturer | Machinery | Resin composition |
---|---|---|---|
Siraya Tech Cast | Siraya Tech(San Gabriel, USA) | ELEGOO Mars 2 Pro | Polyurethane |
FotoDent® Cast | Dreve Dentamid GmbH(Unna, Germany) | Carbon M2 | Methacrylates, initiators, pigments |
Form 3 Castable Wax Resin | Formlabs(Sommerville, USA) | Form 2 | Urethane dimethacrylate |
Post-curing processes were dependent on the specific resin used for each printer. The manufacturer’s instructions were followed as available for each printer:
FotoDent® Cast/Carbon M2 printer: After printing, the excess resin was allowed to drip off the samples for at least ten minutes before washing. The prints were washed with 99% isopropyl alcohol twice for 6 minutes each cycle. The samples were then cured in the corresponding curing unit (Dentamid PCU LED N2, Dreve, Unna, Germany), for 8 minutes with 1 minute gas pre-flow.
Form 3 Castable/Form 2 printer: After printing, excess resin was allowed to drip off the samples for at least ten minutes before washing. The samples were washed in 99% isopropyl alcohol twice for 5 minutes each cycle (Form Wash, Formlabs, Somerville, USA). This resin did not require post-curing.
Siraya Tech Cast/ELEGOO Mars 2 Pro printer: After printing, the excess resin was allowed to drip off the samples for at least ten minutes before washing. These samples were washed with 99% isopropyl alcohol for 3 minutes (Mercury Plus, ELEGOO, Shenzhen, China) and allowed to dry thoroughly. Once dried, the samples were placed in the curing station for 3 minutes, then flipped and cured again (Mercury Plus, ELEGOO, Shenzhen, China).
The printed crowns and reference die were scanned using an intraoral digital scanner (3Shape TRIOS 3, Copenhagen, Denmark). All scans were exported in STL format and imported into a three-dimensional analysis software (Geomagic Control X 2024, 3D systems, Morrisville, USA). To evaluate the internal fit, the prepped die surface was divided into occlusal, axial, and marginal areas (Figure 4). Each scan of the printed crown was imported into the software and aligned with the reference die scan. A 3D comparison was performed to assess the deviation between the intaglio surface of each crown (Figure 5).
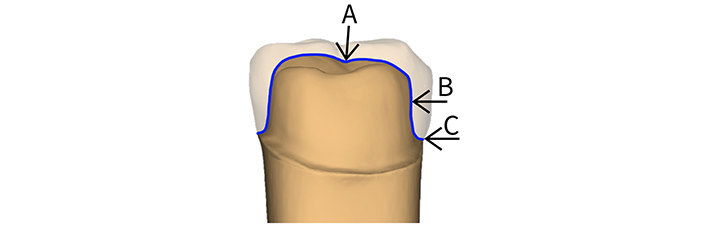
Three areas were evaluated for variation of fit (A: occlusal; B: axial; C: marginal)
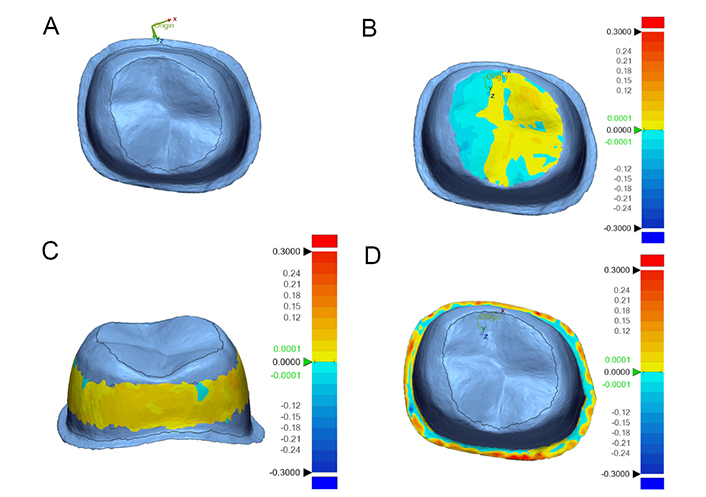
3D analysis of aligned printed crown pattern to the master Die (units in mm). (A) Trimmed master die; (B) color map of occlusal measurement area; (C) color map of axial measurement area; (D) color map of marginal measurement area
Internal fit deviation values were calculated (in µm) using color maps and the root mean square (RMS) method, which is the square root of the mean of the squares of a set of numbers calculated using the following formula [21, 22]:
where n was the total comparison points per each area, and D was the gap distance between the reference die and the crown scans.
Internal fit variation between the different printers and at the different areas was statistically analyzed using a two-way analysis of variance (ANOVA) and Tukey’s multiple pairwise comparison tests using a statistical software program (GraphPad Prism 10.2.3, Boston, USA). Significance was set at P < 0.001 [23].
Results
A statistically significant difference was found between the different printers and at the different measurement areas (P < 0.001). Form 3 Castable resin printed using the Form 2 printer had the lowest mean internal variation in all measurement areas. FotoDent® Cast printed on Carbon M2 had the largest marginal variation (125 µm) while Siraya Tech Cast resin printed using the ELEGOO Mars 2 Pro had the largest occlusal variation (Figure 6A). Table 3 shows the mean and standard deviation of tested materials by measurement area. Within each material, the largest internal variation was at the marginal area while the lowest value was in the occlusal area (Figure 6B) (P < 0.001).
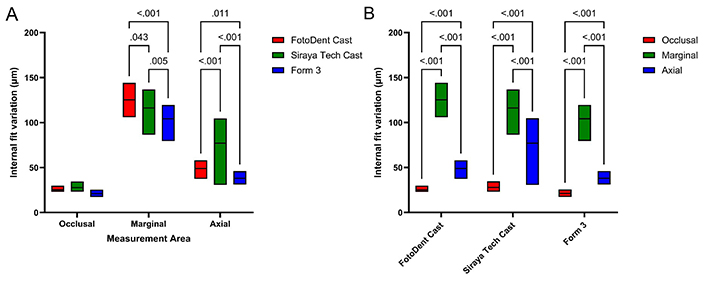
Internal fit variation of crown patterns. (A) Internal fit variation (µm) of printed crowns patterns at each measurement area; (B) comparison of internal fit variation (µm) within each material at different measurement areas
Mean ± SD (µm) of internal fit for each tested group
Group | Measurement area | ||
---|---|---|---|
Occlusal | Marginal | Axial | |
Siraya Tech Cast | 25.27 ± 1.88 | 125.27 ± 9.62 | 48.98 ± 6.26 |
FotoDent® Cast | 27.87 ± 3.65 | 116.19 ± 10.96 | 77.10 ± 23.39 |
Form 3 Castable Wax Resin | 21.07 ± 1.98 | 104.23 ± 10.08 | 37.98 ± 4.46 |
Discussion
This project aimed to analyze the consistency and variation of fit for printed crown resin patterns. The null hypothesis stated that internal fit variation of printed crown patterns would not vary between printers or resins. There was a statistically significant difference in fit variation among the different printers at the different measured areas. Thus, the null hypothesis was rejected.
While all the printers were within the acceptable range for marginal gaps [5], it was still wider than many clinicians would prefer and more than one would expect when using conventional casting techniques. Given that this study evaluated castable resins, the next step in the clinical workflow would be to cast the patterns in a metal alloy or a castable ceramic. This casting procedure may impact the marginal adaptation further, either increasing or decreasing the gap. This would be an interesting follow-up study, to see how the margins would change. Additionally, if a gold alloy is used for casting a pattern, it could be burnished. This would reduce the marginal gap, perhaps making this data less clinically relevant.
One difference found in our study from other literature was that the marginal gap was larger than the axial and occlusal gap no matter the combination of printer and resin. This could be due to several factors that would need to be explored. Other studies evaluating indirect printing measured the fit only after casting/pressing in the final restoration material [8, 12, 13], but they did not measure the space at the printing step prior to the fabrication of the final restoration. Other studies measuring fit of printed crown patterns utilized resin materials meant for provisional or other use, not casting resins [3, 9, 10]. Casting resins often contain wax, which may impact the fit properties. Additionally, those may change after casting. It would be interesting for a future study to evaluate the fit of crown patterns before and after casting.
One consideration when purchasing printers is financial investment. The printers selected for this study vary in cost, for example: The manufacturer’s price of the ELEGOO Mars 2 Pro printer is several hundred times less than the Carbon M2.
Though done with the intention to reduce variables, a limitation of this study was that all crowns were nested at the same angle. The current evidence available determining nesting angle for crowns is based on resins for direct printing, not specifically casting resins. Future studies could determine if these resins should be treated differently. Other drawbacks include variations in material. There was not anyone castable material that was available for all three printers. While all printed resins vary in composition, castable resins have even more variation due to differing wax content. It is unknown how this may have affected the fit.
Another notable limitation is how this resin may be treated by clinicians and technicians may treat these resins based on their experience and the desired outcomes. Often, the designs are modified; increasing the cement space or bulking out the margin to allow for finishing after casting, for example. Modifications such as these are not noted in the directions for use or are evidence-based. Future studies could include investigating the results of design modifications on specific restorative materials in an effort to improve outcomes.
Conclusions
All the printed crown patterns demonstrated a clinically acceptable fit, considering an acceptable marginal gap threshold of 120 µm. Regardless of variations in printer technology and resin composition, the overall quality of the printed crowns meets the required clinical standards.
The marginal area exhibited the greatest variation in fit compared to the axial and occlusal areas. Though clinically acceptable, the precision of the marginal fit varies more significantly, which could impact the clinical outcomes if not carefully managed.
The Form 2 printer using Form 3 Castable Wax Resin achieved the most consistent fit with the smallest internal variation across all measured areas: 21.07 µm in the occlusal, 104.23 µm in the marginal, and 37.98 µm in the axial regions.
Further research is needed to explore how casting processes might influence the final marginal adaptation of printed crowns. Additionally, investigating design modifications and their impact on fit could provide valuable insights into improving the predictability and accuracy of printed dental restorations.
Abbreviations
AM: | additive manufacturing |
CAD-CAM: | computer aided design-computer aided manufacturing |
SM: | subtractive manufacturing |
STL: | standard tesselation language |
Declarations
Author contributions
WAC and IJDK: Conceptualization, Supervision, Writing—original draft, Writing—review & editing. MG: Conceptualization, Investigation, Writing—original draft, Writing—review & editing. TA: Validation, Investigation, Data curation. AM: Investigation, Writing—original draft, Writing—review & editing. All authors read and approved the submitted version.
Conflicts of interest
The authors declare that they have no conflicts of interest.
Ethical approval
Not applicable.
Consent to participate
Not applicable.
Consent to publication
Not applicable.
Availability of data and materials
The datasets that support the findings of this study are available from the corresponding author upon reasonable request.
Funding
Not applicable.
Copyright
© The Author(s) 2024.