Abstract
The fusion of biomaterial science with clinical practice in oculoplastic and orbital surgery, particularly in the reconstruction of the posterior lamella of the eyelid, the lacrimal system, orbital floor fractures, and the development of implants for anophthalmic sockets, represents a frontier where materials meet surgical techniques. This review, which spans research from 2015 to 2023, delves into the application and integration of biopolymers and functional biomaterials in these complex areas. The discussion begins by reviewing the key anatomy of the external ocular surface, lacrimal system, and orbit. It then summarizes the various current surgical approaches for treating diseases affecting the external ocular surface and orbital involvement, with an emphasis on the associated challenges. The discussion continues with a comprehensive overview of the advantages and disadvantages of current and emerging biomaterials, including synthetic and natural polymers, used in reconstructive surgeries. These include applications for eyelid structure reconstruction, lacrimal system repair, orbital bone fracture repair, and orbital socket reconstruction. Throughout the review, the pathophysiology and challenges associated with these reconstructive procedures are explored, with an emphasis on surgical nuances and the ongoing pursuit of optimal reconstruction techniques. Finally, this review serves as a valuable resource for familiarizing clinicians with current knowledge and generating future hypotheses. It concludes that no evidence-based guidelines currently exist in oculoplastic surgery regarding the use of biopolymers in reconstructive procedures. Further research is needed to evaluate the efficacy and reproducibility of these biopolymers.
Keywords
biopolymers, orbital implants, eyelid surgery, lacrimal systemIntroduction
The field of oculoplastic surgery encompasses numerous surgical interventions that can be summarized into three fields: the orbit, the eyelids, and the lacrimal system. Eyelid reconstructions are amongst the most performed surgical interventions globally [1]. Oculoplastic surgical traumas are non negligeable; from 2006 to 2015 in the United States, 4.2 million emergency department visits were shown to be related to primary oculoplastic diagnosis, in which 70% were trauma-induced diagnosis, and nearly half of those cases required a surgical intervention [2]. Orbital floor fracture incidence was shown to have increased by nearly 50% from 2006 to 2017 in the United States [3]. The economic burden of eyelid laceration repair was shown to have further increased by nearly $30 million, accounting for approximately $1,600 per person in the United States [4]. Over the past years, efforts have been deployed to reduce the associated costs with surgical interventions. Current modern medicine, shifting its practice towards sustainable alternatives, has shed light in the past years on the use of polymer composites to leverage the economical, sustainable, and safety challenges, and further provide better patient care [5]. Current breakthroughs in medicine involve the use of polymer composites for orbital implants, posterior lamellar eyelid reconstructions, and as well as the lacrimal system [5–8]. In addition to being a great sustainable alternative to current surgical synthetic materials, polymer composites have shown high biocompatibility and low immunogenicity [9, 10]. The aim of this comprehensive literature review is to provide an overview of the most recent studies involving the use of polymer composites in the field of oculoplastic surgery. To better comprehend the challenges associated with current surgical techniques, the anatomy of the eyelid, lacrimal system, and orbits are first presented. Secondly, the most current surgical approaches for eyelid reconstruction surgeries, dacryocystorhinostomy (DCR), and orbital surgeries are discussed along with their respective challenges. Finally, this review discusses the most novel polymer composites in these surgical settings and proposes future perspectives regarding their possible role in a clinical setting.
The anatomy of the ocular external surface, lacrimal system, and orbit
Eyelid and lacrimal system anatomy
The eyelids and lacrimal system are crucial structures of the eye with various roles such as tear film distribution, tear drainage, protection of the ocular surface, and cosmesis [11]. The average dimension of the adult palpebral fissure varies from 28–30 mm horizontally and 10–12 mm vertically [12]. The eyelid is anatomically divided into two lamellae: the anterior and the posterior lamellae (Figure 1).
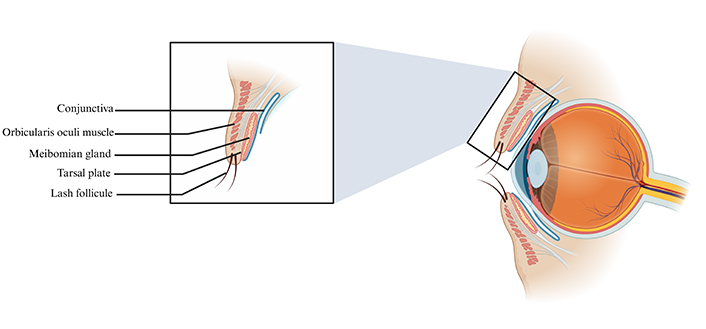
Schematic illustration of the eyelid structures. The eyelid structures encompass the conjunctiva, orbicularis oculi muscle, meibomian gland, tarsal plate, and lash follicle. Created in BioRender. Hocini, A. (2024) https://BioRender.com/z59t140
The anterior lamella of the eyelid is composed of very thin skin and the orbicularis oculi muscle. Given the extremely thin aspect of the eyelid’s skin, it is more flexible than surrounding skin, allowing for repetitive blinking movements [13]; unfortunately, this unique characteristic also poses significant challenges for skin grafts. Furthermore, although the eyelid skin is deprived of surface lipids, the presence of large superficial corneocytes (i.e., terminally differentiated keratinocytes) allows for adequate hydration [13]. The orbicularis oculi muscle beneath the skin is divided into the orbital and palpebral portions. The orbital portion of the orbicularis oculi muscle allows for tight eye closure. The medial portion of the orbicularis oculi muscle plays an important role in the lacrimal pump mechanism [14].
The posterior lamella consists of the tarsus, the orbital septum, and the underlying conjunctiva. The tarsal plate is made up of dense connective tissue, encompassing type I, III, and IV collagen, chondroitin, and keratan sulfate [15, 16], and serves as a structural support for the eyelids. The extracellular matrix of the tarsal plate consists of a collagen-elastin network and highly contributes to the biomechanics of the eyelid tarsus [16]. Finally, the meibomian glands are located within the tarsal plate and are vital for lipid layer synthesis in the tear film [17]. Post-secretion modifications and damages to the meibomian glands can alter the lipid components and synthesis capacity respectively, therefore destabilizing the tear film and leading to dry eye syndrome [17].
Drainage of tears occurs through the lacrimal system. Pathologies involving the lacrimal system at different levels, such as nasolacrimal duct obstruction (NDO) and ectropion’s, can lead to disrupted tear film drainage. The lacrimal system encompasses the lacrimal gland, the punctums, the lacrimal sac, and the ducts and canals interconnecting the lacrimal system (Figure 2) [18].
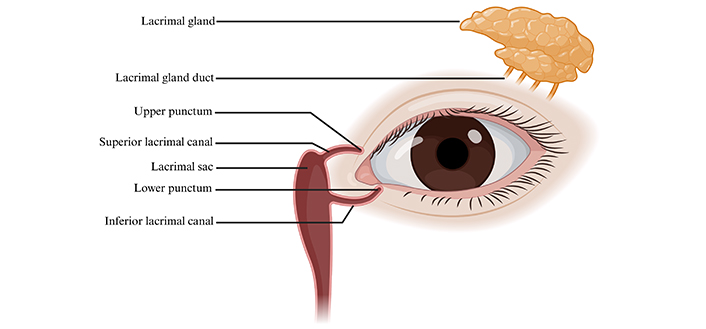
Schematic illustration of the lacrimal system. The lacrimal system structures consist of the following: lacrimal gland, lacrimal gland duct, upper punctum, superior lacrimal canal, lacrimal sac, lower punctum, and inferior lacrimal canal. Created in BioRender. Hocini, A. (2024) https://BioRender.com/o05x888
The main lacrimal gland can be divided into two lobes: the orbital lobe, which is twice as large as its other division, and the palpebral lobe [19]. The orbital lobe lies in the lacrimal fossa while the palpebral lobe lies under the levator palpebrae. The excretory ducts of the main lacrimal gland open into the superior conjunctival fornix. In the lamina propria of the conjunctiva, we can find the glans of Krause and the glans of Wolfring, two subdivisions of the accessory lacrimal glands. The ducts of the accessory lacrimal glands both open onto the conjunctiva surface [20]. The puncta are located near the medial canthi; they serve as the entry point for the lacrimal tears to be drained. Both canaliculi meet at the lacrimal sac. The valve of Rosenmüller is a mucosal flap that prevents tear reflux from the lacrimal sac to the canaliculi [21]. The lacrimal sac lies in the lacrimal sac fossa and runs down vertically to become the nasolacrimal duct. This duct then empties into the inferior nasal meatus. The valve of Hasner, found within the nasolacrimal duct, prevents retrograde displacement of tears originating from the nasal cavity [22].
Anatomy of the orbit
Orbital injuries are often associated with major traumas, where motorcycle collisions account for most cases [23]. However, the mechanism of injury was shown to differ according to age groups and ethnicity [24]. Elderly people were shown to be more prone to have fall-induced orbital injuries, whereas Hispanic and African-American people were shown to have assault-related orbital injuries [25, 26]. Blow out fractures (i.e., fracture to one or more bones surrounding the eye) are the consequence of isolated facial blunt traumas [27]. The orbits are susceptible to injury given their complex anatomy involving soft tissues and 7 bones (Figure 3) [28].
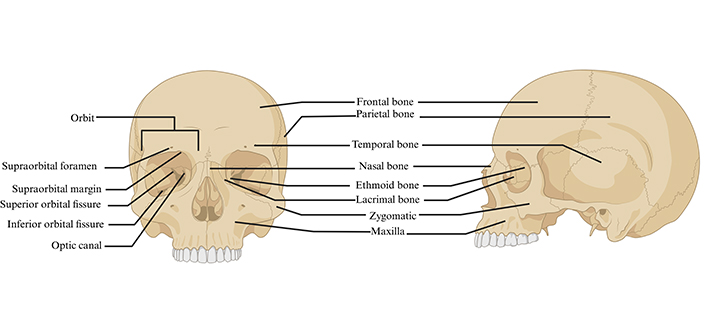
Anterior and lateral view of the human skull structures and orbit anatomy. Created in BioRender. Hocini, A. (2024) https://BioRender.com/u78q526
Current surgical approaches for ocular external surface diseases and orbital involvement and their challenges
Ptosis repair
The prevalence of ptosis was shown to range from 4.7% to 13.5% based on the study population [29]. The pathophysiology of ptosis encompasses a wide array of etiologies [30], such as aponeurotic ptosis [31], neurogenic ptosis [32], third cranial nerve palsy [33], Horner syndrome [34], myogenic ptosis [35], myasthenia gravis [36], chronic progressive external ophthalmoplegia [37], oculopharyngeal muscular dystrophy [38], and traumatic ptosis [39]. Surgery is an effective way to treat this condition and is indicated in cases of visual field abnormality secondary to ptosis or, in some cases, for aesthetic purposes [40]. Depending on the etiology of the ptosis, different surgical techniques can be used (Table 1). The levator aponeurosis advancement procedure consists of an incision through the eyelid skin, followed by dissection of the orbital septum to identify the dehisced or disinserted aponeurosis. The levator is then advanced and sutured to the upper one-third of the tarsus [41]. Conversely, the Muller’s muscle-conjunctival resection (MMCR) technique, involves everting the upper eyelid to expose the conjunctiva to subsequently resect the Muller’s muscle and excess tissue [42]. The frontalis flap is another surgical procedure to treat congenital or acquired ptosis, with the aim of elevating the frontalis muscle to the superior one third of the tarsus with sutures [43]. However, given that this technique is not reversible, it is not indicated in patients with poor levator function secondary to paralytic or myogenic causes, in which post-operative success is dependent upon reversibility and height adjustments [43]. Conversely to this technique, the frontalis sling method utilizes a sling material to elevate the frontalis muscle [44]. Different materials can be used to create the sling, with autogenous fascia considered the material of choice due to its lowest recurrence of ptosis and complications [45]. Other materials used include banked fascia [46], nylon monofilament [47], polyester [48], PTFE [47], polypropylene [49], ETHIBOND [50], and silicone [51]. Recurrence rates vary from one material to another, with PTFE and nylon monofilament yielding the highest complication and recurrence rates [47]. Furthermore, inflammatory reactions, such as mycobacterial infections [52] and orbital inflammation [53], can occur at the site of insertion of the material and have been shown to vary depending on material choice [54, 55]. Overall, each current surgical technique for ptosis repair comes with disadvantages. There is therefore a crucial need to improve surgical techniques to provide better outcome long term.
Key surgical approaches for ptosis repair
Surgical technique | Key features | Advantages | Disadvantages | References |
---|---|---|---|---|
Levator advancement |
|
|
| [30, 40, 41, 56–61] |
Muller’s muscle-conjunctival resection (MMCR) |
|
|
| [42, 61–63] |
Frontalis flap |
|
|
| [64–66] |
Frontalis sling |
|
|
| [44–51, 67] |
Tumor excision
Eyelid tumors can be benign, with multiple examples including papillomas [68], seborrheic keratosis, chalazion [69], nevus [70], or cysts [71]. Malignant tumors include basal cell carcinoma (BCC) [72], being the most common, squamous cell carcinoma (SCC) [73], sebaceous gland carcinoma [74], or Merkel cell carcinoma [75]. Surgical excision with tumor free margins is the gold standard to treat malignant tumors [76]. Ensuing surgical excision, multiple approaches can be used to repair the eyelid. An upper lid defect of up to 20% in young patients and 30% in older patients can be addressed with direct closure [77]. For larger eyelid defects, grafts can be used. An anterior lamellar defect can be reconstructed using a full-thickness skin graft using upper or lower eyelid skin, posterior auricular skin, preauricular skin, or supraclavicular skin [77]. Tarsoconjunctival grafts taken from healthy eyelids are the gold standard for posterior lamina reconstruction [78]. Other eyelid repair techniques include various types of flaps (Table 2).
Summary of eyelid reconstructive techniques following tumor excision
Surgical technique | Key features | Advantages | Disadvantages | References |
---|---|---|---|---|
Autografts |
|
|
| [78, 79] |
Cutler-Beard flap |
|
|
| [80–84] |
Rotational rhomboid flap |
|
|
| [85–87] |
Mustardé rotational flap |
|
|
| [88–90] |
Tripier flap |
|
|
| [91, 92] |
Hughes tarsoconjunctival flap |
|
|
| [93–95] |
Cutler-Beard technique
The bridging flap, also known as the Cutler-Beard technique, is a surgical approach primarily used to repair large defects in the eyelid, in cases such as the resection of a sebaceous gland carcinoma [80]. This intervention requires the placement of a full-thickness cutaneo-conjunctival lower eyelid bridge flap over the upper eyelid after tumor excision [81]. The bridge is then separated a few weeks after the surgery [82]. Unfortunately, multiple limitations and complications are associated with this specific technique, such as the lack of upper eyelid lashes post-reconstruction, vision impairment prior to bridge separation, necrosis of the flap, lower eyelid ectropion, cicatricial ectropion, and upper eyelid retraction [96]. Additionally, the pressure from the bridging skin flap can cause erosion or damage to the cornea. Finally, dehiscence of the graft can occur due to the application of opposite forces by the upper and lower eyelids.
Rotational rhomboid flap
The rotational rhomboid flap can be useful to repair eyelid defects, especially in the medial canthus area [85]. A rhomboid shape defect is created with the virtual long axis, in which the flap can be rotated in multiple directions to cover the defect [86]. The challenge with this technique is ensuring that the stress on the skin is well distributed and no modification of the canthal height is made [86].
Mustardé rotational flap
The Mustardé rotational flap, along with the bridging flap, can also be used for large defects following a tumor excision [89]. In this procedure, a septal cartilage mucoperichondrial autograft is used to reproduce the tarsal plate [88, 89]. The Mustardé flap yields very good cosmetic outcomes because it preserves the texture and skin color of the patient, while ensuring adequate vascularization to the operated area [97]. However, the most common post-operative complication of this intervention is sagging of the lower eyelid and ectropion [88, 89].
Tripier flap
The Tripier flap can be to correct an ectropion after another reconstruction surgery like the Mustardé flap or the V-Y advanced flap [91]. It can also be a good option for correcting full-thickness eyelid defects. The preservation of muscle fibers within the flap provides for excellent tissue vascularization and aesthetic results. This surgery also tends to cause less damage to the facial nerve and keep the integrity of the orbicularis muscle fibers. Other variations of the Tripier flap can be utilized to reconstruct the upper eyelid or even the use of single-pedicle flap [92]. However, since the procedure relies on the properties of existing tissue on the patient, flap size is limited to the amount of excess skin available.
Hughes tarsoconjunctival flap
The Hughes tarsoconjunctival flap involves the creation of a tarsoconjunctival flap from the ipsilateral upper eyelid. Given the two-stage nature of the procedure, it poses similar limitations as mentioned earlier with the Cutler-Beard flap. Furthermore, a careful dissection of the levator muscle from the tarsoconjunctival flap needs to be done to avoid eyelid retraction. Other minor post-operative complications include flap necrosis, corneal abrasion on flap division, mucous discharge, or dry eye syndrome [93, 94].
Lacrimal system impairment
DCR is one of the most common surgeries and is indicated when there is an NDO, that being either congenital or acquired. This obstruction can happen anywhere along the path of the lacrimal duct. DCR involves inserting a silicone tube into the punctum through the canaliculus to reinforce lacrimal drainage. However, it is to be noted that the tubes can be made of different material sources [98]. Silicone stents are currently the most commonly used. However, to reduce the high cost and the risk of infection of silicone stenting, Baruah et al. [99] studied the use of polypropylene stenting. Polypropylene is cheaper, readily available, shows less tissue reaction, and reduces bacterial contamination [99]. This material is also non absorbable and shows good tensile strength [99], therefore providing a great alternative to silicone stenting. External DCR has been thought to be the gold standard in DCR surgeries given the limited technology available for an endoscopic approach [100]. However, with recent advances in technology, endoscopic DCR has shown great success rates and is currently the preferred approach by most surgeons [101]. External DCR describes higher risk of bleeding and wound infection [102]. Endoscopic DCR is beneficial for multiple reasons, such as minimal invasiveness, preservation of the lacrimal pump function, shorter surgical duration, and better cosmetic outcome [102].
Orbital involvement
Orbital bone fracture
Orbital floor fractures are amongst the most common site of injuries [103]. Data analysis from 2006 to 2017 has shown that the incidence of orbital floor fractures in the United States had significantly increased [3]. The primary goal in orbital fracture management is to maintain function and limit further damage on the globe. The majority of patients will benefit from a conservative approach [104]. However, in cases where surgery is necessary, this must be performed within 14 days of injury [104]. The indications for surgery are based on the clinical presentation. The presence of diplopia, enophthalmos (i.e., eye sinking deeper in the eye socket), and ocular motility dysfunctions are criteria for surgery [104]. Additional criteria based on orbital cavity volume and size of the fault area are considered [105]. Taken together, these clinical findings may be suggestive of a muscle entrapment.
Three main factors should be considered in orbital fracture management: the surgical timeframe (i.e., within two weeks), the surgical approach, and the choice of material for the reconstruction. The surgical approaches for orbital floor repair can be divided into two approaches: the transcutaneous (i.e., subciliary, subtarsal, and infraorbital) or transconjunctival approach [106, 107]. The transconjunctival approach is the preferred surgical method for orbital floor fracture repairs. The site of injury is accessed through an incision at the fornix of conjunctiva [106]. However, in many cases, a canthotomy is required to increase exposure rate, and is thus associated with a potential visible scar [108]. The subciliary approach consists on accessing the orbital floor through an incision at the lower lid [106]. One limitation of subciliary approach is its association with higher incidence of denervation of the pretarsal orbicularis [109]. In the subtarsal approach, the orbital floor is accessed through an incision below the eyelid margin in the subtarsal crease and extends laterally. This approach is preferred in comparison to the subciliary approach, given that it preserves the innervation of the tarsus [110]. The infraorbital approach’s advantage is a rapid and direct access to the orbital floor through an incision of the skin, orbicularis muscle, and periosteum [106].
Aside from surgical timeframe and approach choice, the third and final consideration for orbital floor fractures regards material choice for the reconstruction. A variety of materials have been discussed in the literature for this purpose [111]. Unfortunately, there is still controversies in which material is better in orbital fracture reconstruction. Current biomaterials can be categorized into several types. These include biological materials like bone grafts, cartilage and fascia; metals like titanium and cobalt alloys; polymers, that can be porous or non-porous and either absorbable or not absorbable; biological ceramics, like porous hydroxyapatite (HA); and composites, like titanium reenforce polyethylene (PE) to name one (Table 3) [112]. For many years, autologous bone transplants for orbital floor fracture repairs used to be considered as the gold standard [113]. Autologous bone transplant is achieved by harvesting a bone sample from a donor site, consisting of either the mandibular coronoid process, anterior maxillary wall, mandibular symphysis, rib, or scapula, and using it as a rigid support during the reconstructive surgery [114–117]. However, given the increased donor site morbidity, variability in resorption rates, and technical difficulty, treatment paradigm has shifted towards the use of allogenic and mainly alloplastic materials [113, 118]. Allogenic materials in oculoplastic surgery refers to tissues harvested from another individual for surgical purposes, whereas alloplastic materials are from a synthetic origin. The most common alloplastic material in maxillofacial surgery is titanium. Titanium osteosynthesis biomaterials are currently the gold standard in maxillofacial fracture managements [119]. Nevertheless, multiple challenges are also associated with the use of this biomaterial; titanium systems are challenging given the potential restriction in bone growth, sensitivity to temperature, mutagenicity, tactical discomfort associated with the plates and screws, and interference with radiological imaging techniques [120–122]. To leverage the challenges associated with these systems, numerous efforts have been deployed in the past years to develop high standard biopolymers for orbital floor fracture management. In the ensuing sections, we delve into the most recent and novel applications for orbital floor fracture repair, as well as their involvement in orbital bioimplants. However, with the recent advances in the use of biopolymers in medicine, a shift in the clinical interest towards these materials for orbital floor fracture management has been observed. Novel advances in this field will be further discussed in the subsequent sections.
Summary of current materials used in orbital bone fracture repair
Material type | Advantages | Disadvantages | References |
---|---|---|---|
Bone grafts(Calvarium, iliac crest, rib, maxillary sinus) |
|
| [112, 123–127] |
Titanium meshes |
|
| [112, 123–126, 128–130] |
Porous polyethylene sheets |
|
| [112, 123–126, 129, 131, 132] |
Composite of porous polyethylene and titanium meshes |
|
| [112, 124–126, 128–130, 132] |
Resorbable materials(thermoplastic and non-thermoplastics) |
|
| [112, 123–126, 129, 132] |
Preformed orbital implant |
|
| [112, 123–126, 129, 132] |
Globe rupture
In more severe cases involving damage to the eye globe, enucleation or exenteration can be performed. Both of these surgeries may be indicated in various scenarios that create a painful eye, such as trauma to the eye with loss of its content, endophthalmitis with phthisis, and keratoconjunctivitis complicated by globe perforation. These surgeries are also indicated to prevent the progression of an existing eye neoplasia [133].
Orbital exenteration is a radical and disfiguring surgical technique that involves the removal the entire orbital content and the surrounding structures, such as the muscles, fat, nerves and in some cases the eyelid [134]. In contrast, enucleation consists of the removal of the entire eye globe, but preserves the surrounding structures [135].
Once the globe is removed, an orbital implant needs to be inserted for aesthetic purposes and to restore eye volume. Multiple implants are currently being used, but controversies still exist concerning the best choice of implants (Table 4). The most common materials that are currently used are ceramics, autologous materials, silicone, PMMA, porous PE, and poly-HEMA [133]. Although numerous choices are available, these materials encompass various disadvantages such high cost, implant-associated infections, impediment on fibrovascular growth, fistula creation, delayed healing, and tumor recurrence [136].
Summary of current used materials for orbital socket implants
Material type | Advantages | Disadvantages | References |
---|---|---|---|
Ceramics(hydroxyapatite) |
|
| [133, 137–142] |
Autologous materials(Bone, fat, skin, cartilage, muscle) |
|
| [133–135, 143] |
Silicone |
|
| [133, 143, 144] |
Poly(methylmethacrylate) (PMMA) |
|
| [133, 143, 145–148] |
Porous PE (polyethylene)(Medpor) |
|
| [133, 143, 146, 149] |
Poly-HEMA (2-hydroxyethyl methacralate)(Alphasphere) |
|
| [133, 143, 146, 150] |
Overview of polymer composites
In the following sections, a comprehensive review of polymer composites will be presented. Due to some disadvantages highlighted among the various materials previously reviewed in this paper, polymer composites in medicine have experienced rapid growth in recent years. These new polymers are particularly significant and deserve attention, as they have been proposed as innovative alternatives for biomedical applications, including drug delivery, tissue engineering and regeneration, medical devices, and implants [151]. Their biocompatibility with human tissues, non-toxic nature, and their sustainable alternative have rendered biopolymers as an essential tool for modern medicine [152]. Polymer composites can be classified into different categories based on the classification system. They can be categorized based on their nature (biodegradable or non-biodegradable), their composition (i.e., based on the main material), their type (natural or synthetic) or their dimension. Novel classification systems favor a bi-parameter classification, outlining the type and nature of polymer composites (Figure 4). The biochemical properties of these biopolymers have been thoroughly reviewed, therefore they will not be covered in depth in this section [153].
Natural non-biodegradable polymer composites, such as bio-polyethylene terephthalate (PET), bio-PE, bio-polyamide (PA), and bio-poly(trimethylene terephthalate), are a great alternative to current synthetic sutures given their greater sustainability and environment friendly features. Furthermore, being biological products (i.e., plant-based or animal-based materials), they exhibit greater compatibility. However, current knowledge regarding their application in the field of oculoplastic surgery is sparse. In the past years, efforts have been deployed to develop natural non-biodegradable polymer composites in the field of manufacturing, for packaging and engineering (i.e., medical devices) [154]. However, advances in the field of oculoplastics are limited to none. Therefore, this comprehensive literature review will cover the application of the other three categories of polymer composites in the field of oculoplastic surgery. However, to be able to use these materials in reconstructive surgeries, they need to encompass certain general characteristics; the optimal polymer composite should be resorbable, biodegradable, resistant to infection, minimally reactive, cost effective, easy to manipulate, and easily accessible [155]. When used in the setting of an orbital floor fracture repair, they should in addition be osteoconductive, not induce capsule formation, and possess a half-life long enough to favor bone growth [155].
Novel applications of polymer composites in oculoplastic surgery
Materials for blepharoplasty and suturing
Natural polymer composites
Sutures are of great importance in reconstructive oculoplastic surgery. The tensile strength, biocompatibility and thermal stability are key features when engineering a suture. Furthermore, given the incidence of infection with blepharoplasty (0.2%) [156], the possibility to engineer a polymer composite with lower post-operative complication rates is highly sought after. Furthermore, biodegradable sutures are of interest given their greater esthetic outcome in eyelid reconstructive surgeries. The first approved natural polymer composite for suturing is the polyhydroxyalkanoate (PHA)-based suture from TephaFLEX [157], which was shown to induce no adverse effects one year post intramuscular implantation of the PHA-based sutures in animal models [158]. Since, numerous efforts have been deployed in developing new suture alternatives based on polymer composites for modern medicine [159]. Recently, de la Harpe et al. [160] have proceeded with the engineering of a monofilament bioabsorbable suture composed of gelatin, sodium alginate, pectin and glycerol, that has drug delivery capabilities. They showed great biocompatibility of the suture with no cytotoxicity, as well as sustained drug delivery for 7 days [160]. The suture was successfully biodegraded within 28 days [160]. Similarly, Liu et al. [161] have shown the potential of polylactic acid (PLA) sutures loaded with microspheres enclosed drugs to delivery drugs post-surgically in a sustained manner, while maintaining the optimal mechanical properties [161].
Collagen and chitosan-based polymer composites have also shown great potential as suture alternatives. Collagen nanofibrils (CoNF) confer great mechanical stability. CoNF were shown to promote tendon-derived cell proliferation in vitro [162]. In fact, wound healing is highly dependent on cell proliferation at the surgical site. Conversely, chitosan-coated silk sutures have also shown great antibacterial efficacy [163, 164], mainly against Staphylococcus epidermis and Candida albicans [165]. Although these results were obtained in fields not involving reconstructive eyelid surgeries, the same outcomes can be hypothesized. However, further studies in the field of oculoplastic are needed to better understands the visual and clinical outcomes of natural polymer composites as alternatives to standard sutures.
Synthetic polymer composites
Non-biodegradable sutures are of interest in oculoplastic surgery for specific applications, such as tarsorrhaphy’s (i.e., procedure consisting of approximating the upper and lower eyelid in conditions with corneal exposure, such as chronic lagophthalmos). Clinical efficacity of PE sutures have been demonstrated on numerous occasions [166]; PE is amongst the most studied polymer composites for various biomedical applications [167, 168]. However, a major challenge regarding their use concerns their long-term safety and biodegradation.
Materials for lacrimal system stenting
Natural polymer composites
Selvam et al. [169] compared the utilization of various polymers to support the morphological and physiological characteristics of rabbit purified lacrimal gland acinar (PLGA) cells for the development of a novel treatment for dry eye syndrome. They compared the efficacy of collagen 1, silicone, poly-D,L-lactide-co-glycolide, Thermanox®, and poly-L-lactic acid (PLLA), and concluded that PLLA was the most effective biopolymer among the others in supporting the growth of PLGA [169]. In 2009, the same authors continued their research and developed a microporous PLLA membrane (mPLLAm) to enhance scaffold properties for lacrimal acinar cells by aiming for improved permeability. The results were very promising and suggested that this biomaterial could be a promising avenue for the development a bioartificial lacrimal gland device [170]. A recent study created a decellularized lacrimal gland-derived hydrogel and compared its capacity to promote proliferation of porcine lacrimal gland epithelial cells to other biomaterials like Matrigel and collagen-1 [171]. After 24 h of hydrolyzing, the decellularized hydrogel led to better epithelial growth and functionality compared to other materials [171]. This in vitro experiment could potentially pave the way for the next generation of lacrimal gland engineering devices. In 2017, Hsiao et al. [172] decided to approach pathologies of the lacrimal glands with another angle; instead of finding a way to replace the gland, they decided to use a chitosan biomaterial to create a biocompatible environment to promote and mimic the branching structure formation of the lacrimal gland. The biomaterial works by increasing affinity of the lacrimal gland for hepatocyte growth factor (HGF), which is beneficial for the branching morphogenesis and structural development [172]. Hsiao et al. [173] also highlighted one of the main limitations of chitosan, which was the elimination of the polymer functionality after using certain pathway inhibitors such as PD98059 and LY2944002, inhibiting the MAPK and Akt/PKB pathways respectively [173]. Even though little research on this polymer exists, these two projects led by Hsiao et al. [173] could be the starting point for a new use of biopolymers in lacrimal gland regeneration.
Synthetic polymer composites
Pinilla et al. [174] conducted a non-randomized prospective clinical trial to evaluate the effectiveness of fluoroscope-guided nasolacrimal stents of polyurethane on 86 patients with obstructive epiphora. Of the successfully placed stents, 40 (52.6%) were clinically successful, meaning they achieved resolution or net improvement of the symptoms [174]. Mild complications like epistaxis, palpebral hematoma, pain, or other less common complications were encountered in 19 eyes, and 33 stents became occluded after 24 months [174]. The study concluded that this technique was a good alternative for DCR, but yielded fewer effective results than the surgery itself [174]. Conversely, Baruah et al. [99] studied 51 cases of dacryocystitis and evaluated the effectiveness of polypropylene for stenting after DCR. The surgery was successful for 92.2% of the patients, which is comparable to the results of other studies [175–178]. The principal advantage of this polymer is its possibility to be a great alternative to silicone stents, considering its good efficacy and lower cost [99]. A study involving 91 patients even stated no significant differences between the success rate of silicone and polypropylene stenting for endoscopic DCR [179]. An important element to consider is the lack of consensus in the literature regarding the real efficacy of silicone stents [180–182]. Silicone stenting, when used for the treatment of epiphora, has been reported to have certain disadvantages such as its risk of bacterial infection, its poor efficacy due to its hydrophobic nature, or the surgical challenge associated with this stent due of the curved shape of the lacrimal duct [183]. The study conducted by Park et al. [183] tried to seek solutions to address these disadvantages of silicone stenting. After experimenting with different materials, they synthesized a shape memory polymer (SMP) with elastic proprieties that allowed self-expansion, made of 94% polycaprolactone (PCL)-6%PGMA [183]. This polymer demonstrated excellent biocompatibility, improved efficacy in tear drainage, and superior resistance to bacteria compared to the conventional silicone stent, suggesting that it could be a better alternative [183]. Dai et al. [184] created an in situ degradable lacrimal plug made of methacrylate-modified silk fibroin (SFMA) which serves as a network with indocyanine green fluorescence tracer nanoparticle (FTN) [184]. In vivo testing on rabbits with dry eyes demonstrated that this trackable lacrimal duct plug could be a very good approach for the treatment of this condition in the future, considering the net improvement of symptoms with better lacrimal fluid retention and no inflammatory response [184]. In 2011, Chaloupka et al. [185] described a promising solution to resolve the frequent complication of duct blockage or displacement associated with the implantation of rigid Lester Jones tubes for tear duct surgery. They created a conduit made from polyhedral oligomeric silsesquioxane-poly(carbonate-urea) urethane (POSS-PCU) and demonstrated its superiority to its predecessors by demonstrating its improved biocompatibility, structure, and functional abilities [185].
Grafts
Natural polymer composites
One of the biggest challenges in eyelid surgery is finding an adequate replacement material for the tarsus. Although, autografts and acellular dermal matrices (ADMs) are potential polymer composites for eyelid reconstruction surgeries, they have limitations; autografts may carry iatrogenic risks, and ADMs can cause infections and immunologic responses [186]. ADMs consist of connective tissue matrix—a collagen matrix—that does not exhibit immunogenic properties [187]. ADMs possess several beneficial properties that make them a suitable choice for eyelid reconstruction surgery. They provide rapid and long-term adherence to the wound surface, resistance to mechanical stress, and elastic properties making it able to adapt to ocular contours; other benefits of ADMs include the absence of antigenicity and toxicity, cost-efficiency, and extended shelf life as compared to other alternatives [188]. In a study involving 20 patients who underwent eyelid tumor resections, Ma et al. [189] explored the use of ADMs for eyelid reconstruction. They utilized ADMs as a substitute for the tarsal plate and the conjunctivae post-tumor resection. The study concluded that ADMs could be an effective alternative for posterior lamellar material. The results were promising: pedicle flaps survived in 90% of the patients, no tumor recurrence was noted, visual acuity and ocular movement were preserved in all patients, and half of the patients achieved excellent aesthetic results [189]. In addition to the long surviving time period, the authors mention that this polymer composite can assure stability of the eyelid, exhibit a good biocompatibility, and reduce complications related to the donor site [15]. Another study involving 21 patients also focused on reconstructive eyelid surgery following tumor resections [190]. It concluded that xenogeneic acellular dermal matrix shows encouraging results as a biomaterial for future tarsal plate replacement procedures [190]. Barmettler et al. [191] pursued a prospective randomized clinical trial in 2018 to compare the outcomes of the reparation of eyelid retraction using three different graft types: autologous auricular cartilage, bovine ADM, and porcine ADM [191]. The results showed significantly increased postoperative swelling in the auricular cartilage group compared to the other alternatives [191]. Although no other statistically significant differences in overall outcomes were observed among the three groups, the auricular cartilage grafts exhibited a higher inflammatory response. This finding suggests that clinicians may need to consider other criteria before using them and might prefer ADMs in order to avoid additional surgical sites and reduce surgical time [191]. Additionally, the absence of conjunctival surface with auricular grafts in ADM could potentially increase patient comfort.
Conversely, to imitate the eyelid tarsus, Sun et al. [192] applied fibroblasts, previously cultured from human eyelids, on chitosan hydrogel scaffolds. Using immunocytochemical staining, they demonstrated the scaffold’s ability to support and promote the growth and proliferation of human fibroblasts [192]. This innovation could potentially be a big step in the field of eyelid reconstruction, giving a personalized and biocompatible replacement to the tarsus. Another study by Drechsler et al. [193] investigated the use of compressed collagen for replacing the epithelial conjunctiva in fornix reconstruction surgeries. They found that this biopolymer had excellent strength and elasticity, but was also as malleable as the amniotic membrane (AM) [193], therefore providing promising avenues for this biopolymer for future advancements in conjunctival surgeries. Xu et al. [186] combined collagen and chitosan (COL/CS) to create a biphasic scaffold, and further integrated a COL/CS sponge with a poly(propylene fumarate)-co-2-hydroxyethyl methacrylate (PPF-HEMA) polymer network [186]. The PPF-HEMA layer acts as a structural base, while the COL/CS sponge retains water and imitates the conjunctival layer. This results in reduced inflammation and improved cell infiltration and proliferation [186]. We think that this multi-biomaterial approach could then be a great way for creating advanced scaffolds in eyelid surgery, using the unique key points of each biopolymer to compensate for the limitations of others.
Over the past years, the AM has proven to be very effective and useful in various pathologies and situations such as socket contracture, cicatricial disorders like Stevens-Johnson syndrome [194], cryptophthalmos [195], conjunctivochalasis [196], and even as an adjuvant in skin grafts [197]. In 2017, Singh et al. [197] reported a case involving a 20-year-old woman with a junctional eyelid kissing nevus. Like other studies on the subject [198–200], the combination of skin grafts and AM grafting (AMG) led to very good functional and cosmetic outcomes. Rahal et al. [198] studied the midterm results following resection of ocular surface squamous neoplasia of the fornix. The surgical repair technique used a mucosal graft combined with AMG. Among the 83 eyes from the 76 patients in the study, only 23 (27.71%) underwent successful corrective surgery, partially explained by secondary complications such as symblepharon, cicatricial ectropion, pannus, and corneal decompensation [198]. This study concluded that this technique preserved the vision, anatomy, and function of the eye confirming the efficacy of this surgical procedure [198]. In another case report, Reed et al. [201] described a 20-year-old male who underwent surgery for a right upper eyelid laceration following a car accident. They utilized a combination of a skin graft and AMG, which yielded good cosmetic results; the authors went onto highlighting the importance of the AM which acts as a scaffold for the healthy surrounding skin to proliferate [201]. This novel technique proved to be advantageous for multiple reasons: It avoids contracture of the surrounding tissue and reduces the need for large skin graft, which is beneficial for extensive lesions where skin availability is limited. The procedure can also be done without sedation, it can be very useful in settings where an operating room is not available. Finally, the low risk of scarring makes it a technique of choice because it can occur with many other management techniques.
A case report by Borrelli et al. [202] described the management of a 49-year-old woman with an adenoid cystic carcinoma in the pterygopalatine fossa who underwent multiple radiotherapy and surgical treatments. The patient developed lagophthalmos due to seventh nerve palsy and lead to exposure keratopathy because of cornea exposure post-operatively. Due to the lack of healthy skin for autologous grafts, a decellularized porcine-derived membrane (tarSys) was selected to treat the lower lid retraction. Three months after the surgery, no lagophthalmos or symptoms of keratopathy were present, however, the long-term outcomes of the procedure were not reported. In 2013, a retrospective study was conducted by Liao et al. [203] to evaluate the efficacy of tarSys for the treatment of lower lid retraction in patients with graves ophthalmopathy. This study, which included 37 eyelids, concluded that tarSys was a good alternative for eyelid grafts. Another case report was published on a 54-year-old woman who underwent bilateral lower eyelid surgery using a porcine decellularized membrane graft [204]. Due to unilateral eye pain months after the procedure, the graft was removed after 15 months [204]. Pathological analysis revealed an inflammatory response, demonstrating that a host reaction can occur even in the theorical absence of antigens. Given that the literature on the use of decellularized grafts in humans is perhaps limited, these biopolymers should only be used as a second line of treatment or when other materials are unavailable or contraindicated.
Synthetic polymer composites
In 2004, Tan et al. [205] studied the use of porous PE lower eyelid spacers (Medpor LES) for the management of different causes of eyelid retraction. A Medpor LES was utilized in 32 patients for a total of 35 eyelids and the study results demonstrated a statistically significant clinical improvement 22 months post-surgery and a decrease of the following complications: palpebral aperture, lagophthalmos, increased L-MRD, and LSS [205]. However, other significant complications were reported in seven patients, consisting of implant exposure and reduced eye mobility, ultimately leading to the removal of the implant [205]. Eight patients also encountered minor complications requiring further surgical intervention such as transient lash loss, skin contour abnormalities, or margin ectropion. Although, the procedure yielded adequate results for 24 eyelids, 28% of the cases had satisfactory or poor outcomes. Due to the high number of complications and the frequent need for reoperation, this technique should be considered only when autologous grafts are not possible. The main advantage of this technique is to reduce surgical time and can be very useful for patients lacking skin to graft [206].
Xu et al. [207] further analyzed the potential of branched porous polyethylene for eyelid reconstruction, which offers a superior alternative for soft tissue replacement. They mainly focused on the elasticity properties of branched porous polyethylene with the aim of mimicking the eyelid’s tarsus. The study resulted in promising results. During subcutaneous implantation in rats, they observed a very mild inflammatory response, along with collagen deposition and fibro-vascularization [207]. Their trial on rabbits, demonstrated that the polyethylene had the same elasticity and repair properties of Medpor transplants used in the control group [207]. It also resulted in fewer eyelid deformities and a higher degree of fibrovascular tissue formation than the Medpor transplants [207]. This suggests that porous polyethylene could be a better option for in vivo eyelid reconstruction, particularly because of its non-toxic properties, addressing potential concerns about its use. However, to the best of the author’s knowledge, no trials involving human eyelid have been done. Poly(3-hydroxybutyrate-co-3-hydroxyhexanoate) (PHBHHx) is another synthetic polymer used in reconstruction of the eyelid. Zhou et al. [208] explored the use of PHBHHx as a substitute for the tarsus in comparison to ADMs. They found that both polymers were effective materials used for eyelid repair. Histological analysis two weeks post-implantation in rats revealed inflammation in this group, despite PHBHHx demonstrating good biocompatibility and elasticity [208]. Such findings could indicate that alternative polymers with lower inflammatory responses might be preferable. Other studies have also explored the employment of PCL for conjunctival reconstructions [209] or for tarsal reconstruction [210].
Mesh for orbital bone fractures
Natural polymer composites
Natural biopolymers (i.e., biomaterials derived from nature) that have demonstrated their efficiency and safety in orbital injury repairs mainly encompass gelatin film. Gelatin films have been used for over two decades in oculoplastic surgery. Derived from denatured collagen, gelatin films are efficient for the reconstruction of small orbital defects (< 5 mm), whereas in larger defects, it can be used to bridge two distinctive materials such as plates and meshes [211]. When compared to silicone rubber, gelatin films were shown to decrease the migration of implants, relieve the post-surgical inflammatory processes, and contribute to a better healing outcome [212]. Although natural biopolymers have excellent biocompatibility and biodegradability, few challenges exist with the use of natural biopolymers, such as limited availability and great complexity associated with its extraction and synthesis. Synthetic biopolymers can overcome these challenges and are advantageous for many reasons, such as their tunable properties and consistent structure [213].
The use of protein-based coatings on synthetic biopolymers have recently been contributing to the paradigm shift in reconstructive maxillo-facial surgeries, including orbital floor fracture injuries. Recombinant bone morphogenic protein (BMP) can be coated onto numerous biopolymers, where its addition was shown to favor osteointegration of synthetic biopolymers through material integration and surface charge modulations [214, 215]. Four types of biopolymer coatings can be employed: transforming growth factor (TGF) β, BMP-2, vascular endothelial growth factor (VEGF), and fibroblast growth factor (FGF) [216]. However, studies regarding their use in orbital injuries are limited.
BMP-2 coating is mainly used for its strong osteoconductive power [216]. Its use in orthopedic surgeries is well known [217], however, studies encompassing orbital injuries are limited. Using polylacticglycolide acid copolymer coated with BMP-2 in a sheep model with an orbital floor fracture, Zheng et al. [218] have demonstrated the efficacity of the biomaterial in reconstructive surgery; upon absorption of the coated biopolymer, the defect was filled with a complex tissue (i.e., bone tissue, connective tissue, and mucosal epithelial layers), representative of the normal environment [218]. The use of additives and coatings is currently in emergence and additional studies along this topic are to be expected within the next years.
Orbital socket implants
Semi-synthetic polymer composites
Silicone has been used for decades in reconstructive surgeries for its cost-effectiveness, adherence capacity, enhanced permeability, and thermal and chemical stability [220–222]. However, given the significant implant-related complications, such as implant extrusion, socket edema, implant migration, and mechanical obstruction, current clinical practice has shifted away from the use of silicone implants [223, 224]. Semi-synthetic biopolymers have gained interest within the last five years given their similarity to the bone structure, their ability to effectively reconstruct orbital floor fractures, and the ability of mass production for these compounds.
HA compounds are known as ceramic materials and are mainly composed of calcium and phosphate ions, which confers its highly biocompatible and non-toxic properties [225]. However, challenges regarding the porosity, density, and composition of HA limits their use in single-based biomaterials [226]. HA-derived biopolymers’ efficacity in orbital floor and wall reconstruction surgeries has been demonstrated in conjunction with PLLA and PCL [227–229]. A recent retrospective study showed similar safety and efficiency of unsintered HA (uHA)/PLLA in comparison to PCL mesh for orbital wall fracture reconstructions [228]. However, one recent study has reported the presence of postoperative diplopia and enophthalmos in 12 patients who underwent orbital wall fracture repair with uHA/PLLA [230]. This outcome was reported to be induced by the extent and severity of the fracture. The Hess area ratio (HAR) is an objective and quantitative tool to measure eye movements and has shown great utility in assessing the post-surgical outcome in patients with orbital blowout fractures [227, 231]. When compared to silicone sheets or a combination of sheets, no significant differences in postoperative HAR was obtained with uHA/PLLA, suggesting that uHA/PLLA biopolymers are as effective as conventional materials for orbital floor fracture reconstruction [227].
Synthetic polymer composites
A study investigating the efficacity of PMMA implants in patients who underwent enucleation secondary to retinoblastoma’s demonstrated very little complications; implant migration was noted in only 28 (9%) patients and implant exposure and contracted socked was reported in 5 (2%) patients for each outcome [220]. These recent contributions to the novelty of PMMA implants and biopolymers are along the same findings reported nearly two decades ago [147].
Porous PE-Medpor-usage is not exclusive to eyelid reconstructive surgeries; the successful usage of Medpor as implants has also been showing great results [232–236]. In a recent 6-month follow-up study published this year, porous PE implants were shown to be associated with nearly absent complications: 1 patient (6%) exhibited implant migration, whereas 93% of participants reported a satisfactory outcome [234]. However, given that non-negligeable infection and implant exposure and distortion long-term complications have been reported with porous PE, the clinical decision to use these biopolymers in certain patients should be made with precaution [237–239].
Future directions in biomaterials research
A substantial amount of work has already been conducted on the study of these biomaterials, as reviewed in this paper. The authors have identified several gaps in the current literature and aim to highlight these areas to encourage further studies. It would be valuable to conduct further studies on the various drugs used in different eyelid and orbital surgeries, with a focus on their interactions with these new biomaterials. Studies employing numerical modeling approaches, such as the Finite Element Method or Multiphysics approach, would be highly beneficial for testing and evaluating differences of those biomaterials in the specific context of oculoplastic surgeries.
Conclusions
Since the first discoveries in biopolymers for orbital implants and reconstructive surgeries, numerous advances and challenges attributed to autologous graft transplants have been conquered [240]. The realm of the current practice is fulfilled with a variety of biomaterials and a challenge regarding the choice of surgical implants and grafts for reconstructive surgery exists. Novel advances have shifted their paradigm towards the development of biocompatible, effective, non-toxic, cost-effective, and sustainable biopolymers for reconstructive eyelid, lacrimal, and orbital surgeries. Although numerous preclinical studies have underlined the efficiency of these biomaterials, no scientific-based recommendations in oculoplastic exist regarding the use of biopolymers in reconstructive surgeries. Further studies and meta-analyses are required to outline the efficiency and reproducibility of these biopolymers. Nevertheless, this review bridges the clinical knowledge regarding the surgical approaches in oculoplastic surgery with the fundamental sciences behind the development of novel biopolymers. It not only serves as a tool for clinicians to familiarize with the current knowledge but also guides research hypothesis within the field given the raised challenges throughout the comprehensive literature review.
Abbreviations
ADMs: | acellular dermal matrices |
AM: | amniotic membrane |
AMG: | amniotic membrane grafting |
BMP: | bone morphogenic protein |
COL/CS: | collagen and chitosan |
CoNF: | collagen nanofibrils |
DCR: | dacryocystorhinostomy |
HA: | hydroxyapatite |
HAR: | Hess area ratio |
NDO: | nasolacrimal duct obstruction |
PCL: | polycaprolactone |
PE: | polyethylene |
PHA: | polyhydroxyalkanoate |
PHBHHx: | poly(3-hydroxybutyrate-co-3-hydroxyhexanoate) |
PLGA: | purified lacrimal gland acinar |
PLLA: | poly-L-lactic acid |
PMMA: | poly(methyl methacrylate) |
PPF-HEMA: | poly(propylene fumarate)-co-2-hydroxyethyl methacrylate |
uHA: | unsintered HA |
Declarations
Author contributions
MK: Visualization, Project administration, Writing—original draft, Writing—review & editing. KYW: Conceptualization, Writing—review & editing. AH: Visualization, Writing—original draft, Writing—review & editing. PD: Supervision, Writing—review & editing. All authors read and approved the submitted version.
Conflicts of interest
The authors declare that they have no conflicts of interest.
Ethical approval
Not applicable.
Consent to participate
Not applicable.
Consent to publication
Not applicable.
Availability of data and materials
Not applicable.
Funding
Not applicable.
Copyright
© The Author(s) 2024.