Abstract
Hydrogel-based drug delivery systems (DDS) offer promising alternatives for treating ocular diseases by overcoming the limitations of traditional therapies, such as low bioavailability, frequent administration, and invasiveness. Hydrogels, with their high biocompatibility and ability to respond to external stimuli, can provide sustained and targeted drug delivery. This review highlights the unique properties of hydrogels, including their swelling behavior, porosity, and mechanical strength, making them suitable for various ocular applications. The classification of hydrogels based on cross-linking methods, origins, and stimuli responsiveness is discussed, emphasizing their potential in drug delivery for dry eye disease (DED), glaucoma, corneal alkali burns, and neovascularization. Notable advances include thermosensitive and pH-responsive hydrogels, which have shown promising results in preclinical studies. Despite these advances, most studies are still in preclinical stages, highlighting the need for rigorous human trials to validate the safety and efficacy of hydrogel DDS. Collaborative efforts among researchers, pharmacologists, and ophthalmologists are essential to translating these innovations into clinical practice, ultimately improving patient outcomes in ocular disease management.
Keywords
Ocular drug delivery systems, hydrogels, intravitreal delivery, ophthalmology, retinal diseases, conjunctival deliveryIntroduction
The World Health Organization reports that 2.2 billion people are afflicted with vision impairment and in almost half of these cases, vision impairment can be prevented or treated [1]. Globally, vision impairment imposes significant financial consequences, with the estimated cost of productivity to be 411 billion USD [2]. However, effective management of ocular pathologies can be challenging given the eye’s multiple systems of barriers. Although current pharmaceutical and surgical interventions can mitigate certain pathologies, they also come with various limitations such as low bioavailability, frequent administrations, invasiveness, and increased risks of infection [3]. To overcome the limitations of traditional treatment strategies, many researchers have adopted hydrogel-based drug delivery systems (DDS) as a potential solution [4]. These delivery systems are highly advantageous as they have high biocompatibility, are non-invasive, and can be engineered to respond to specific external stimuli [5]. This review discussed the unique composition and properties of hydrogels, followed by innovative applications of hydrogels as alternate therapies for ocular diseases.
Overview of hydrogels
Definition & properties
Hydrogels are hydrophilic, cross-linked polymer networks that can absorb significant amounts of water [6]. Upon exposure to aqueous environments, hydrogels can exhibit swelling behavior without compromising structural integrity [7]. The unique swelling response of hydrogels renders them highly useful for a wide variety of applications [8]. In the pharmaceutical industry, hydrogels have garnered popularity because of their ability to trap within the cross-linked matrix a wide array of therapeutic agents that range in size and water solubility [9]. Sustained and targeted drug delivery can easily be achieved by modifying hydrogels’ various features such as swelling rate, porosity, biocompatibility, and mechanical strength [10]. Additionally, many hydrogels have been engineered to respond to specific changes in the environment [10]. Given that many ocular diseases are characterized by changes in pH, temperature, or ion concentrations, hydrogels can be highly effective in detecting abnormal physiological changes and providing timely pharmaceutical interventions. Figure 1 provides a comprehensive summary of the applications of hydrogels in ophthalmology.
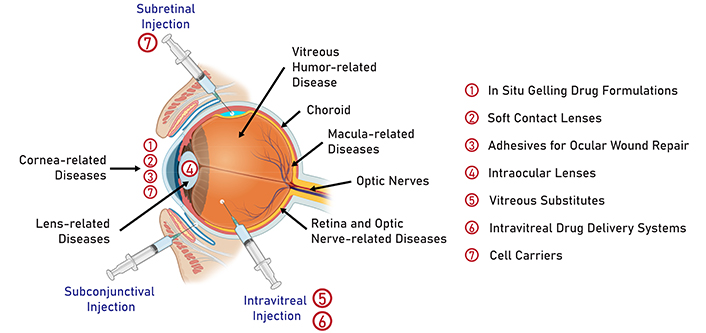
Common routes of drug administration in ophthalmology. Numbers 1 to 7 on the right denote the application of hydrogels in the treatment of anterior and posterior segment eye diseases. Created in BioRender. Nguyen, L. (2024) https://BioRender.com/g77e803
Classifications of hydrogels
The highly versatile nature of hydrogels calls for multiple classification methods that can account for the diverse physicochemical features of hydrogels (Figure 2). This section highlights the main classification methods that apply to ophthalmic applications.
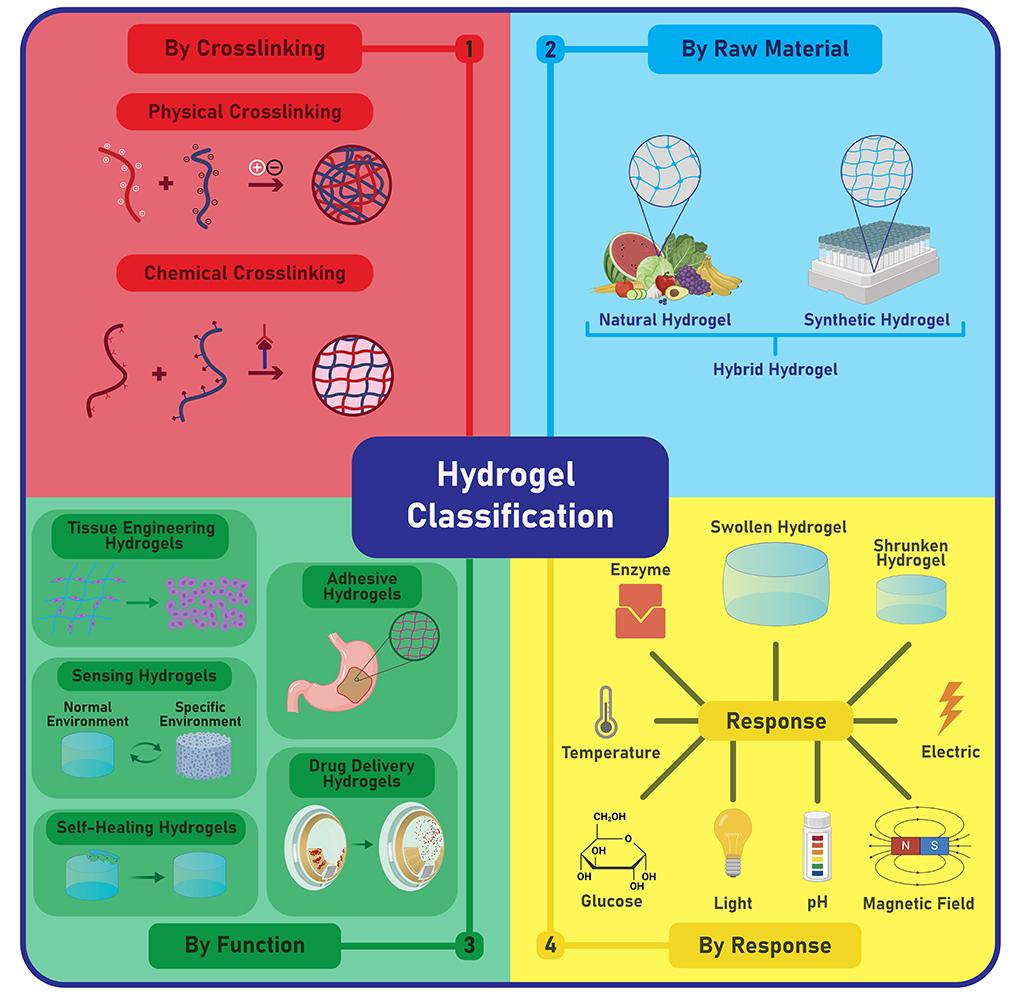
Hydrogels can be classified based on cross-linking methods, raw materials, functionality, and responses to external stimuli. This review focused solely on drug delivery hydrogels and classified these through three methods: cross-linking, raw materials, and responses to external stimuli. Created in BioRender. Nguyen, L. (2024) https://BioRender.com/h28k738
Based on the cross-linking method, hydrogels can be divided into either physical or chemical categories [11]. Physical crosslinking involves non-covalent interactions such as hydrophobic interactions, hydrogen bonds, ionic bonds, or Van der Waals force [11]. Because no toxic crosslinking agents are needed, physical hydrogels are generally biocompatible in vivo. However, the weak interactions among polymeric chains render physical hydrogels highly sensitive to changes in environmental conditions [12]. While this is a desirable feature for the creation of reversible, stimuli-responsive hydrogels, it is also a concern as the rapid breakdown of the hydrogel can limit long-term clinical applications [12]. In contrast, chemical crosslinking occurs through covalent bonding, hence the superior mechanical strength and permanent structure of chemical hydrogels [13]. Moreover, precise modulation of hydrogel features (e.g., porosity, degradation rates, swelling behavior) can be achieved via various chemical mechanisms such as free radical polymerization, condensation reactions, or addition reactions [13]. However, careful purification of potentially toxic crosslinking agents and by-products is required to enhance the biocompatibility of chemical hydrogels [8].
The hydrogels’ origins constitute another method of classification. Specifically, hydrogels can be derived from either natural, synthetic, or hybrid polymers [3]. Natural polymers refer to polysaccharides, proteins, or other biopolymers that are naturally found in biological systems [14]. Common natural polymers include agarose, alginate, silk fibroin, chitosan, and hyaluronic acid [14]. Given their natural origin, this type of polymer is extremely biocompatible, biodegradable, and can be obtained from renewable sources [15]. However, applications of natural polymers are limited as they often lack mechanical durability. Conversely, synthetic polymers, or man-made polymers, can often be customized to meet specific chemical, physical, and mechanical needs [16]. Examples of common synthetic polymers include polyethylene glycol (PEG), poly(N-isopropyl acrylamide) (PNIPAAm), and poly(hydroxyethyl methacrylate) (PHEMA) [17]. The controlled production process of synthetic polymers represents a major advantage as it minimizes the variability of polymers between production batches [17]. However, many synthetic polymers lack biocompatibility or biodegradability, hence the increased risk for adverse immune responses. Given the distinct advantages and limitations of each polymeric type, many researchers have resorted to combining both natural and synthetic polymers to create a hybrid hydrogel that is durable and highly compatible for ocular use [18].
Lastly, smart hydrogels can be categorized according to their responses upon exposure to varying external conditions [19] (Figure 3). In ocular medications, commonly used stimuli-responsive systems include thermosensitive hydrogels, ion-sensitive hydrogels, and pH-sensitive hydrogels [20]. Ther-mosensitive hydrogels adopt a liquid state at a room temperature of 25°C and transition to a gel state at human physiological temperatures of 37°C [20]. Ion-sensitive hydrogels undergo sol-gel transition when there is a change in the ionic concentration of the environment [21]. Precisely, the reagents in the hydrogel and the biological fluid may carry opposite charges, hence the strong ionic forces that lead to sol-gel conversion [21]. In some cases, the quantity or type of cations present in the polymer can determine the gel’s viscosity or the rate at which sol-gel conversion takes place [22]. pH-responsive hydrogels swell in response to changes in environmental pH levels [22]. For instance, polymers containing acidic groups undergo gelation at pH above their pKa values [22]. The rising pH levels of the environment can deprotonate the polymers, resulting in excessive polymer-polymer repulsion that activates gel formation [21]. In contrast, polymers with basic moieties experience gelation as external pH decreases [23]. Essentially, the highly favorable polymer-polymer bonds can prompt the hydrogel to undergo a physical transformation [23].
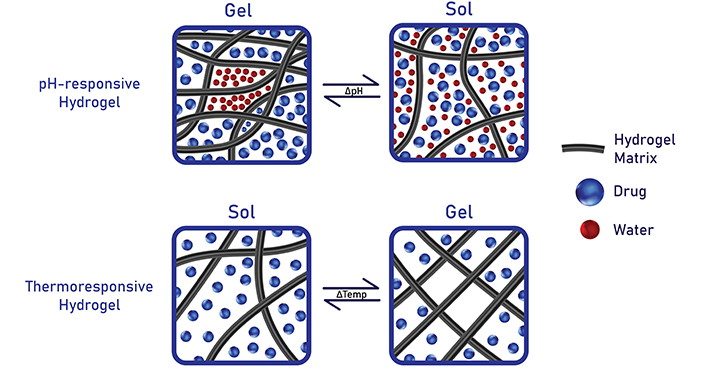
Stimuli-responsive hydrogels can swell or de-swell upon exposure to environmental changes such as pH, temperature, or ions. Created in BioRender. Nguyen, L. (2024) https://BioRender.com/y64e509
Hydrogel in ocular surface drug delivery
The conventional method of drug delivery to the eye involves topical administration of ophthalmic formulations (Figure 1). However, traditional eye drops have limited bioavailability due to the eye’s many systems of barriers [24]. Descriptions of the different precorneal and corneal barriers have been elucidated in past studies [25–27] (Figure 4). Briefly, rapid loss of drug solution can occur due to blinking, tear turnover, or nasolacrimal drainage. Meanwhile, in the outermost layer of the cornea, the epithelial cells possess tight junctions and efflux pumps that promote drug loss either by preventing paracellular drug permeation or inhibiting drug entry into the cell [28]. The challenges of bypassing such robust barriers are often compensated by patients having to instill eye drops multiple times a day or using formulations with higher drug concentrations [29]. The inconvenience of current topical medications has inspired many researchers to study the use of hydrogels as an alternate therapy for many ocular diseases. Essentially, the viscous nature of the gels constitutes a major advantage in prolonging ocular residence time, thereby increasing the efficacy of disease treatment. In this section, we will review the most recent applications of hydrogels as ocular surface DDS (Table 1).
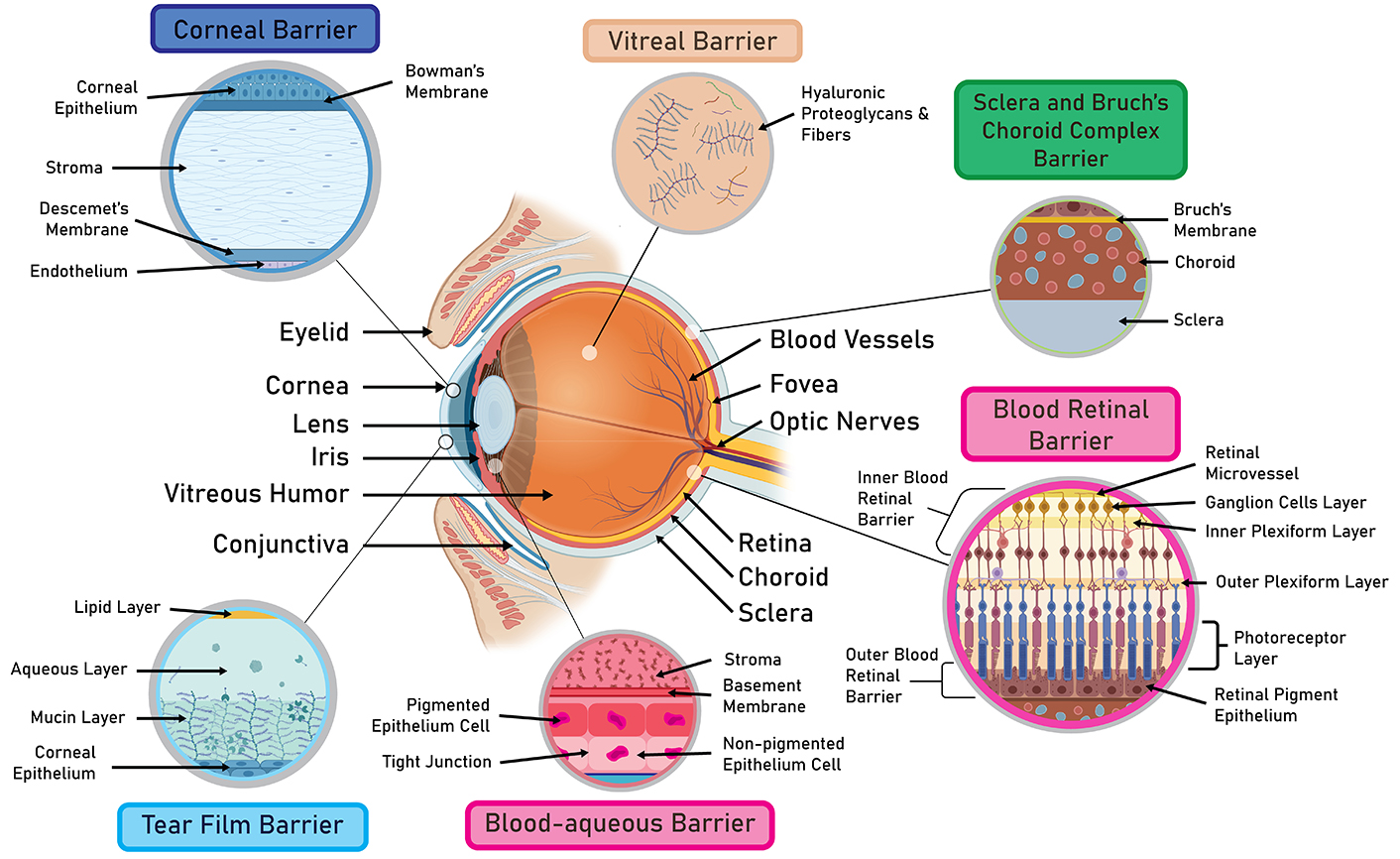
A comprehensive overview of ocular barriers. The robust system of ocular barriers greatly diminishes the clinical efficacy of current ocular therapies. As such, there is a pressing need to develop drug-delivery systems that can penetrate through these barriers and improve therapeutic efficacy. Created in BioRender. Nguyen, L. (2024) https://BioRender.com/k31m936
Summary of recent applications of hydrogels as ocular surface DDS
Disease | Hydrogel composition | Delivery route & active ingredients | Study phase | Release duration | Advantages & limitations | Reference |
Dry eye disease (DED) | Aldehyde-functionalized F127 | Multifunctional antioxidant Cu2-xSe nanoparticles | In vitro; in vivo (mice) | Up to 72 hours | Thermosensitive; scavenging of reactive oxygen species; reduced cell damage & apoptosis in cornea and conjunctiva; have not been tested on human patients with DED | [60] |
Phenylboronic acid-grafted carboxymethyl cellulose | Glutathione (GSH) | In vitro; in vivo (rabbits) | 50 hours | Reduce inflammation caused by preservative in eye drops (benzalkonium chloride); have not been tested on human patients with DED | [90] | |
Carbomer | Cyclosporine A | Phase III clinical trial | - | Once-a-day treatment for moderate to severe DED; short follow-up duration; long-term safety remains unknown; safety profile compared to other DED topical medications remains unknown; patients with surgery-related DED, drug-related DED, or contact lens wear are excluded from the study | [61] | |
Glaucoma | Hydroxypropyl methylcellulose | Curcumin | In vitro; in vivo | 7 days | Decrease inflammation & apoptosis; biocompatible; have only tested biocompatibility on normal animal models; have not tested on animals with glaucoma | [24] |
Chitosan | Pilocarpine | In vitro; in vivo (rabbits) | Within 4 hours | pH-Responsive; burst release that can suppress extreme IOP elevation; prevent damage of endothelium and retinal degeneration 4 hours post-application; the long-term safety profile remains unknown | [37] | |
Mono-methacrylated β-cyclodextrin (β-CD) monomer; HEMA | Puerarin; curcumin | In vitro | Under redox conditions: 5 hours; under non-reducing conditions: 6 hours | Highly sensitive to reduced GSH; need to be tested on glaucomatous animal models | [36] | |
Corneal alkali burn | Hydroxypropyl methylcellulose & sodium hyaluronate | Imatinib | In vivo (mice) | 6 hours | All ingredients used in the formulation are FAD-approved; simple process of formulation preparation; suitable for large-scale production; prompted corneal wound healing & alleviated inflammation; additional anti-scarring drug is needed to achieve greater treatment results | [41] |
Polyamino acid-based poly-S-nitrosothiols (PGlu-TEPA-SNAP) | Nitric oxide (NO) | In vitro; in vivo (mice) | More than 12 hours | Thermosensitive; effective therapeutic effects with minimal ocular complications compared to the traditional dexamethasone treatment; potential application in leptin-based or NO works for ocular therapy; potential application for reluctant corticosteroid therapy of injured cornea | [45] | |
Oxidized chitosan; silk methacrylate (SilMA) | Black phosphorus quantum dots | In vitro; in vivo (mice) | - | Photocurable; pH-responsive; reduced corneal fibrosis & promoted ocular surface reshaping; neutralized free radicals under acidic conditions | [46] | |
Oxidized chitosan; silk fibroin | DNase I | In vitro; in vivo (mouse) | 7 days | Photocurable; expedited wound repair through increased migration of corneal stromal cells; the precise mechanism of the neutrophil extracellular trap neovascularization cascade effect remains unclear | [47] | |
Corneal neovascularization | Poloxamer-407 (pluronic F127); oxidized sodium alginate | Bone morphogenetic protein 4 (BMP4) | In vitro; in vivo (rats) | More than 72 hours | Thermosensitive; applied 3 times a day; reduce inflammation & alleviate corneal edema; reduced production costs; biocompatible | [54] |
Self-assembling peptide | Aflibercept (Eylea); cell penetrating peptide | In vitro; in vivo (rabbits) | More than 25 hours | Neutralization of the negatively charged Eylea allowed the drug to penetrate the corneal stroma; prevent the formation of new abnormal vessels with minimal effect on normal vessels; potential application for treatment of diabetic retinopathy and AMD | [55] |
DDS: drug delivery systems; IOP: intraocular pressure; HEMA: hydroxyethyl methacrylate; DNase I: deoxyribonuclease I; AMD: age-related macular degeneration; -: no data
Glaucoma
Globally, glaucoma is the leading cause of irreversible blindness [24]. In glaucoma, degeneration of the optic nerve is caused by elevated intraocular pressure (IOP) [30]. The two most common forms of glaucoma are primary open-angle glaucoma (POAG) and angle-closure glaucoma, with African Americans more susceptible to the former and Asians more prone to the latter [7]. In POAG, the accumulation of fluid due to clogging of the drainage system leads to elevated eye pressure [31]. Known as the “silent thief of the night”, POAG often exhibits no symptoms in its early stages. Noticeable symptoms are only found in the later stages of POAG where a loss in peripheral vision is often followed by a loss in central vision [32]. In angle-closure glaucoma, the bulging of the iris obstructs the drainage angle of the eye, thereby blocking the circulation of fluid [33]. Currently, the backbone of glaucoma treatment is eye drops. However, the low bioavailability of conventional glaucomatous eye drops remains a concern. Additionally, because glaucoma pathogenesis also involves oxidative stress and pH disturbances, the traditional therapeutic strategy of only stabilizing IOP is insufficient [34]. Currently, extensive efforts have been deployed to develop new hydrogel-based formulations that incorporate antiglaucoma drugs and antioxidant agents. Given that glaucoma is a chronic condition that requires long-term management, synthetic hydrogels are often favored as they can be engineered to reach the anterior chamber, deliver drugs sustainably, and remain structurally stable over an extended period of time [35].
Cheng et al. [24] employed hydroxypropyl methylcellulose (HPMC) hydrogels as a carrier for curcumin, a natural polyphenol with antioxidant, anti-tumor, anti-microbial, and anti-inflammatory effects. Although previous works have researched the use of curcumin in treating ocular hypertension, the limited precorneal retention time via topical administration remains a challenge. In this work, topical administration of HPMC-based formulation not only demonstrated sustained curcumin delivery for 7 days but also alleviated apoptosis, oxidative damage, and inflammation in H2O2-damaged cells. Additionally, the hydrophilic nature of HPMC enables the gel to serve as an effective ocular lubricant, thereby mitigating symptoms of dry eye disease (DED). Despite the promising findings, further examinations must be conducted on glaucomatous animal models to truly confirm the formulation’s effectiveness.
Xu et al. [36] combined β-cyclodextrin (β-CD) monomer and HEMA to form a hydrogel-based carrier for curcumin and puerarin. Extracted from wild kudzu roots, puerarin has been used widely in clinical settings as a long-term therapy for glaucoma and ocular hypertension. Nonetheless, the hydrophobic nature of puerarin remains a challenge in creating long-lasting topical formulations. In this study, the new hydrogel differs from conventional glaucomatous medications in that it is highly sensitive to reduced glutathione (GSH). Precisely, low GSH levels can deteriorate the disulfide bond found between the β-CD monomers and main chains in the hydrogel backbone, thereby prompting the rapid release of β-CD/drug complexes. Results from in vitro tests demonstrated that sustained delivery of both curcumin and puerarin lasted for 6 hours in non-reducing conditions and 5 hours in reducing conditions. While the redox-sensitive hydrogel carries significant therapeutic potential, the lack of in vivo experiments remains a concern.
Nguyen et al. [37] presented the use of pH-sensitive pilocarpine-loaded chitosan hydrogel for the treatment of acute glaucoma. Although acute glaucoma is not as prevalent as its chronic counterpart, the sudden rise in IOP can lead to permanent blindness shortly after onset. The current management method of acute glaucoma includes surgery, which is ineffective in hindering the progression of retinal degeneration and optic neuropathy. Meanwhile, current topical medications have such rapid drug clearance rates that they are incapable of mitigating extreme IOP elevation. Because the pH microenvironments of hypoxic tissue are lower than that of healthy ones, the novel gel can quickly release an IOP-reducing drug upon detecting changes in pH level. Moreover, the innovative delivery system also eliminates the need for patients to undergo surgical interventions. Despite the optimistic outcomes, the long-term safety profile of the gel must be determined before clinical translation.
Corneal alkali burn
Corneal alkali burn is a serious ocular injury that results from exposure to alkali substances such as ammonia, lye, or lime [38]. Given the ability of alkaline substances to saponify fatty acids in cell membranes, alkali burn can rapidly penetrate beyond the ocular surface, causing damage to deeper ocular structures [39]. Persistent, uncontrolled inflammatory stimulation following alkali burns can result in chronic epithelial defects, corneal scarring, and neovascularization, thereby leading to a compromise in corneal transparency and visual quality [40]. The current therapeutic regimen involves hourly administration of corticosteroids and antibiotic drugs to suppress inflammation, facilitate epithelial recovery, and prevent infection [38]. However, the high frequency of instillation and significant ocular irritation often lead to poor patient compliance and poor treatment outcomes [41]. Consequently, there is a pressing need to develop medications that can increase the retention time of drugs, achieve multi-targeting of the pathological process, and minimize ocular discomfort. Of all the types of hydrogels, natural hydrogels are used extensively in the creation of corneal burn treatment [42]. Given that the goal of any corneal burn treatment is to achieve rapid wound healing and tissue regeneration, natural hydrogels are able to serve this purpose in multiple ways. First, natural hydrogels are non-toxic and can promote a moisture-retentive environment, thus facilitating corneal cell regeneration while minimizing discomfort [43]. Second, natural hydrogels can easily be degraded by natural enzymes which allows the healing process to proceed smoothly. Lastly, natural hydrogels are transparent which allows for optimal visual acuity [44]. In this section, we will discuss the most recent applications of natural hydrogels in corneal alkali burn treatment.
Wang et al. [41] formulated hydrogels consisting of FDA-approved HPMC and sodium hyaluronate as a carrier for imatinib (IMB). Although IMB has previously been considered for use in ophthalmology, its poor water solubility hinders clinical translation. In the present study, the use of hydrogel presented several advantages compared to conventional IMB solutions. First, the improvement in aqueous solubility of IMB via hydrogel allowed for a sustained IMB release of 6 hours on the ocular surface. Second, in vivo tests on mice not only showed great compatibility but also a significant reduction in inflammation. Lastly, given the simple preparation process, the novel gel carries immense potential for large-scale production. However, it is important to note that an additional anti-scarring drug must be incorporated to achieve satisfactory anti-scarring effects.
Qin et al. [45] presented the use of ion-sensitive polyamino acid-based poly-S-nitrosothiols (PGlu-TEPA-SNAP) hydrogel in enhancing the therapeutic efficacy of nitric oxide (NO). Although NO is known as a potent regulator of various life processes, its fleeting half-life and limited diffusion render its medical applications difficult. In this study, body-temperature responsive hydrogel significantly enhanced the retention of NO carriers on the ocular surface, with NO delivery lasting for more than 12 hours. Moreover, the hydrogel’s cationic side chains not only improved its hydrophilicity but also enabled it to bind tightly to the negatively charged surface of the cornea. In addition, the in situ gel prompted rapid corneal wound recovery via leptin/leptin receptor-related signaling, ultimately prompting the regeneration of the epithelial cells. Most importantly, compared to conventional dexamethasone, the novel gel was able to achieve the same therapeutic effects without causing any ocular complications.
Zhang et al. [46] reported the creation of black phosphorus quantum dots (BPQD)-loaded pH-responsive gel consisting of silk methacrylate (silMA) and oxidized chitosan nanoparticles (Figure 3). Several advantages are offered by this formulation. First, the hydrogel’s sensitivity to acidic microenvironments around the inflamed tissues enabled the rapid release of BPQD, thereby suppressing the innate immune fibrosis cascade. Moreover, the ability of silMA to undergo photopolymerization following exposure to sunlight allowed it to assemble into a protective film on the ocular surface. In vivo experiments on mice also confirmed the hydrogel’s ability to neutralize free radicals, block downstream pathways, and expedite corneal wound recovery. Ultimately, findings from this work serve as a critical basis for the future development of antioxidant-based therapies.
In another study conducted by the same team of researchers, silk fibroin and chitosan-based hydrogels were used to deliver deoxyribonuclease I (DNase I), an endonuclease that regulates neutrophil extracellular trap (NET) [47]. Following alkali burns, a buildup of NETs is often found at the injury site which can aggravate corneal neovascularization (CoNV). Although liquid dosage forms of DNase I are available, multiple administrations are required to achieve therapeutic efficacy, thereby raising the risk of infection. Overall, the DNase I-loaded hydrogel not only maintained steady release for 7 days but also decreased NET production by 50% and neovascularization by 70%. Although future research is needed to elucidate the mechanism behind the NET neovascularization cascade, the present work represents an important stride in treating ocular complications after alkali burns.
Corneal neovascularization
CoNV is a vision-threatening condition that involves the abnormal growth of blood vessels into the otherwise avascular cornea [48]. Annually, there are roughly 1.4 million people who are affected by CoNV, with 12% of cases resulting in total blindness. The onset of CoNV can be induced by a wide variety of causes, including congenital diseases, contact lens-related hypoxia, inflammatory disorders, chemical burns, limbal stem cell deficiency, allergy, trauma, infectious keratitis, autoimmune diseases, and corneal graft rejection [49]. In CoNV, increased levels of vascular endothelial growth factor (VEGF) can promote angiogenesis, thereby leading to corneal opacification and decreased visual acuity [49]. One of the treatments for CoNV, despite its varying degrees of effectiveness, involves using anti-VEGF agents (e.g., bevacizumab, aflibercept). These agents can suppress the formation of new blood vessels [50]. Nonetheless, subconjunctival delivery of anti-VEGF drugs has several limitations such as rapid drug clearance, limited drug permeation, poor patient compliance, and potential inflammation/swelling around the injection site [51]. The limited efficacy of current anti-VEGF DDS has inspired researchers to investigate stimuli-responsive hydrogels as an efficient and non-invasive method of delivering anti-VEGF agents. Most importantly, given the short-term, surface-level nature of most CoNV treatments, natural hydrogels constitute an ideal candidate for delivering anti-VEGF agents [52]. Combined with their anti-inflammatory and moisture-retentive properties, natural hydrogels can be highly effective in reducing inflammation and blood vessel growth in the cornea [53].
Although previous discoveries have found that bone morphogenetic protein 4 (BMP4) plays a central role in suppressing VEGF-A production, conventional ophthalmic medications have yet to incorporate BMP4. Nan et al. [54] presented the first thermosensitive hydrogel-based formulation that includes BMP4. Specifically, the loading of BMP4 into the porous Pluronic F127/oxidized sodium alginate hydrogel allowed BMP4 to exert its therapeutic effects for more than 72 hours. Applying the BMP4-loaded hydrogel to the ocular surface alleviated corneal edema and repaired microvilli, intercellular junctions, and deformed apical junctional complexes. The low production costs of the gel also constitute another major advantage in clinical translation. However, the sol-gel conversion temperature at 31°–32°C remains a concern. Given the average human body temperature of 37°C, premature gelling could be an issue for CoNV patients who live in colder regions of the world.
Zhou et al. [55] reported the production of aflibercept-loaded eye-drop hydrogel mediated with cell-penetrating peptide. The proposed formulation offers several advantages compared to previous ones. First, the innovative use of cell-penetrating peptide facilitates the penetration of aflibercept into the corneal stroma. Meanwhile, the incorporation of hydrogel extended the aflibercept’s residence time on the ocular surface, further enhancing the drug’s therapeutic effects. Administration of the gel on rabbit CoNV models resulted in a significant reduction in the neovascularization density without compromising normal blood vessels. Given the gel’s prolonged delivery of more than 25 hours, future topical formulations incorporating biocompatible peptides and proteins could be developed to treat other ocular diseases such as diabetic retinopathy or age-related macular degeneration (AMD).
Dry eye disease
DED is a multifactorial disease that is characterized by tear film instability [3] (Figure 3). Common symptoms of DED include visual disturbances, ocular irritation, pain, redness, and burning sensation [56]. One of the cornerstone treatments for DED is the use of artificial tears [57]. However, the efficacy of topical artificial tears is limited by their rapid clearance through the lacrimal drainage system, necessitating frequent daily administration. This frequent use demands a high level of patient compliance and hand-eye coordination [58]. As DED is associated with the tear film containing excess ions (i.e., hyperosmolarity) [59], stimuli-responsive hydrogels have been substantially studied as potential DED treatments.
As oxidative stress damage is known to exacerbate DED, Ou et al. [60] developed an antioxidant Cu2−xSe-loaded thermosensitive gel (Figure 3). The new formula undergoes sol-gel conversion upon exposure to the increased temperature around the site of inflammation. The in situ gel functions as superoxide dismutase and GSH peroxidase, scouring the ocular surface for excessive free radicals. The marked ability of the gel to scavenge reactive oxygen species was known to last for up to 72 hours, and decreased cell damage and apoptosis in the cornea and conjunctiva were observed in mice. However, because mice tear film differs significantly from that of humans, further testing on human patients with DED is needed to validate the gel’s safety profile.
In a similar study aimed at mitigating oxidative stress, Cheng et al. [25] assessed the use of pH-responsive 3-aminophenylboronic acid-grafted carboxymethyl cellulose hydrogel as a carrier for GSH (Figure 3). In vitro, results confirmed the ability of the gel to decrease inflammation and apoptosis while promoting cell viability. Biocompatibility tests done on rabbits also indicated minimal ocular discomfort. Nonetheless, given rabbits’s low blinking and tear production rates, additional trials must be done on humans with DED to gauge the therapeutic effects of the gel accurately.
In a phase III clinical trial, Peng et al. [61] investigated the safety and efficacy of cyclosporine A-loaded pH-sensitive Carbomer gel on human patients with moderate to severe DED. Drastic improvements in tear secretion and DED symptoms were noted for 312 test subjects. Moreover, the novel formulation offers several advantages including a convenient once-a-day treatment regimen, high compatibility, and a fast onset time of 2 weeks compared to that of a cyclosporine A emulsion. Although the gel is a promising solution for long-term DED therapy, several limitations must be addressed. First, the follow-up period for all test subjects was 84 days, which is inadequate to determine the long-term safety profile of the gel. Second, although the gel exhibits extensive therapeutic effects, a comparison study between this gel and other conventional topical drugs must be done to fully understand the gel’s effectiveness. Lastly, because patients with surgery-related DED, drug-related DED, or contact lens wear are excluded from the study, it is unclear whether the same therapeutic effects will apply to these groups of patients.
Hydrogels in conjunctival drug delivery
The conjunctiva constitutes another barrier that impedes drug penetration and distribution within the eye [62]. Components that compose the conjunctival barriers include epithelium cells, blood vessels, and lymphatic vessels [63] (Figure 4). The conjunctival epithelium is the first line of defense against foreign substances and possesses tight junctions that restrict drug permeation [63]. Meanwhile, the numerous blood vessels and lymph vessels of the conjunctiva can absorb the drug and redirect it toward systemic circulation, thereby reducing ocular bioavailability [64]. In recent years, substantial studies have been devoted to creating medications that can both penetrate the conjunctival barriers and achieve sustained release. In this section, we will review the most recent applications (i.e., within 3 years) of hydrogels as conjunctival DDS (Figure 1) (Table 2).
Summary of recent applications of hydrogels as conjunctival DDS
Disease | Hydrogel composition | Delivery route & active ingredients | Study phase | Release duration | Advantages & limitations | Reference |
Glaucoma | Chitosan; sodium alginate | Subconjunctival implant; inner core: timolol maleate; outermost layer: levofloxacin | In vitro; in vivo (rabbits) | Levofloxacin: 9 days; timolol maleate: up to 17 days | Photothermal effect: release more drugs upon irradiation; multidrug delivery system with structures that resemble the different layers of a lollipop; cause eye discomfort after long-term use; long-term toxicity needs to be investigated | [64] |
Hyaluronic acid | Thin films in the conjunctival sac; timolol | In vitro; ex vivo (pigs) | Over 24 hours | Direct loading of drug into HA-based matrices; drug availability across the entire film surface; lack of in vivo experiments | [65] | |
Corneal alkali burn | PLGA-PEG-PLGA triblock polymer | Subconjunctival injection; adalimumab; aflibercept | In vitro; in vivo | 3 months | Thermosensitive; complete inhibition of corneal neovascularization; increased protection of the retina and optic nerve; minimal systemic exposure; the extent to which the drug delivery system affects therapeutic outcomes of TNF-α/VEGF inhibition is not clear | [66] |
DDS: drug delivery systems; HA: hyaluronic acid; PLGA-PEG-PLGA: poly(lactic-co-glycolic acid)-poly(ethylene glycol)-poly(lactic-co-glycolic acid); TNF-α/VEGF: tumor necrosis factor alpha/vascular endothelial growth factor
Glaucoma
Wang et al. [64] engineered a chitosan/sodium alginate hydrogel as a carrier for timolol maleate and levofloxacin. In this project, the proposed hydrogel has multiple unique features. First, given the researchers’ inspiration of the various layers of a lollipop, the hydrogel was constructed to carry different drugs. Precisely, timolol maleate was loaded in the inner core and the 3 layers surrounding the hydrogel’s core while levofloxacin was loaded in the outermost 5th layer. The innovative structure not only allows the hydrogel to prolong the release of antiglaucoma drug (i.e., timolol maleate) but also mitigates infections that are often involved in subconjunctival implants (i.e., levofloxacin). Secondly, heating of the hydrogel via near-infrared irradiation can increase the drug release rate and rapidly lower IOP, hence the highly customizable nature of the system. Moreover, in vivo experiments on rabbits also showed that the rabbits’ IOP was stabilized for 13 days, with the delivery of timolol maleate and levofloxacin lasting 17 days and 9 days, respectively. Although the new technology holds great potential, prolonged use of the implant could cause ocular discomfort. Moreover, the long-term toxicity and degradation of the hydrogel in the eye require further investigation.
Tighsazzadeh et al. [65] developed timolol-loaded hyaluronic acid films to be inserted into the conjunctival sacs. Compared to traditional eye drops, several advantages are offered by the films, namely increased local drug concentrations, sustained drug release (i.e., 24 hours), increased patient compliance, and uniform drug distribution across the entire cornea. However, given the lack of in vivo experiments, the true therapeutic efficacy of the system remains unknown. Therefore, future examinations must be conducted on glaucomatous animal models to determine the film’s effectiveness and biocompatibility.
Corneal alkali burn
Zhou et al. [66] suggested the use of thermosensitive poly(lactic-co-glycolic acid)-poly(ethylene glycol)-poly(lactic-co-glycolic acid) (PLGA-PEG-PLGA) triblock polymer hydrogel for the dual delivery of anti-VEGF antibodies and tumor necrosis factor alpha (TNF-α) inhibitors. While the use of neutralizing antibodies has been applied widely in clinical settings, achieving sufficient bioavailability remains a challenge. The proposed hydrogel-based system differs from traditional subconjunctival formulations in that it prevents the rapid breakdown of the antibodies in the subconjunctival space. Moreover, the use of biodegradable polymers as well as limited catalyst usage significantly minimizes the hydrogel’s risk for other side effects. Sustained delivery of the antibodies to the cornea, iris, and retina lasted for 3 months, thereby reducing the need for repeated injections. Most importantly, the combination therapy offers extensive neuroretinal protection whilst minimizing systemic exposure. Given the multi-ocular protection and prolonged retention time, this novel therapeutic approach represents an important stride in the treatment of severe ocular injuries.
Hydrogels in intravitreal drug delivery
Treatment of posterior segment eye diseases often entails intravitreal injections or the direct delivery of medication to the vitreous humor [62] (Figure 1). Compared to other routes of administration, intravitreal delivery is highly effective in bypassing several key ocular barriers such as the blood-retinal barrier or the sclera [52] (Figure 4). However, several drawbacks are associated with this technique, namely repeated monthly injections, poor patient compliance, and increased risks for endophthalmitis and retinal detachment [67, 68]. Currently, there is an urgent need to develop new DDS that can effectively reach the target site whilst demonstrating sustained therapeutic effects. In recent years, hydrogel DDS has been extensively explored to prolong the therapeutic duration of intravitreal medications (Table 3).
Summary of recent applications of hydrogels as intravitreal DDS
Disease | Hydrogel composition | Delivery route & active ingredients | Study phase | Release duration | Advantages & limitations | Reference |
Retinal neovascularization | GelMA | Triamcinolone acetonide | In vitro; ex vivo | More than 90 days | The degradation process does not obstruct the trabecular meshwork; gelatin is an inexpensive material; no sudden release burst, reducing the risk of cytotoxicity; no measurements of kinetic release profile in vivo | [76] |
Pluronic 127 | Ranibizumab | In vitro; in vivo (rabbits) | More than 7 weeks | The first study to create a Schiff-based injectable; dynamic reversible gel-sol conversion can be squeezed out of a syringe easily; self-healing properties that protect the drug while preventing dispersed particles from obstructing aqueous humor circulation | [74] | |
PNM/PNF | Dexamethasone; anti-VEGF | In vitro; ex vivo (rabbits) | Dexamethasone: 35 days; anti-VEGF antibody: 13 days | Thermosensitive; prolonged degradation time under neutral pH; the optimal dosing regimen remains unclear; further preclinical and clinical trials are needed | [91] | |
Wet AMD | Peptide amphiphile molecule (E-PA) | Ranibizumab | In vitro; in vivo (rabbits) | 10–150 hours | Dosage can be adjusted for each patient by changing peptide concentration; biodegradable | [87] |
CaCl2 | anti-VEGF agent; betamethasone phosphate (BetP) disodium | In vitro; in vivo (mice) | 30 days | Antioxidant properties; the formulation is easy to prepare and is suitable for mass production; future clinical trials on human patients required; limited understanding of the mechanism behind BetP-based DDS | [88] | |
F127/F68 | Rhein; baicalein | In vitro; in vivo | At least 14 days | Thermosensitive; inhibit choroidal neovascularization through anti-angiogenic and anti-oxidative stress pathways; alternative therapy for wet AMD patients who are unresponsive to anti-VEGF antibodies | [89] |
DDS: drug delivery systems; GelMA: gelatin methacryloyl; PNM/PNF: polynuclear metalloids/proprioceptive neuromuscular facilitation; VEGF: vascular endothelial growth factor; AMD: age-related macular degeneration
Retinal neovascularization
Retinal neovascularization (RNV) is a potential complication of several ischemic retinal diseases including retinopathy of prematurity, retinal vein occlusion, and diabetic retinopathy [4]. Briefly, ischemic retinal diseases involve the lack of sufficient blood flow to the retina, thereby leading to nutrients and oxygen deprivation in retinal cells [69]. To compensate for such conditions, the retina upregulates signal molecules, namely VEGF, to stimulate neovascularization, or the formation of new blood vessels, in an attempt to continue nourishing the ischemic and hypoxic retinal cells [70]. While the formation of new blood vessels is generally essential for ocular development, ischemia-induced neovascularization poses significant risks. The newly formed blood vessels are fragile and prone to leakage, and they are often accompanied by fibrovascular tissue that can contract, leading to tearing and damage to the underlying retina. Serious consequences of ischemia-induced RNV include traction retinal detachment and vitreous hemorrhage [71]. Current treatment strategies for RNV primarily involve the use of anti-VEGF agents as well as laser photocoagulation [72]. Given that the treatment of RNV often involves the long-term inhibition of blood vessel formation, the ideal anti-VEGF carrier must be able to both reach the retina and achieve slow release of drugs. As such, anti-VEGF drug production often employs synthetic hydrogels as these hydrogels can be engineered to meet the needs for a deep, prolonged drug action [73].
Given the short intravitreal half-lives of many commercial anti-VEGF drugs, there is an urgent need to formulate an advanced DDS that can prolong the effects of anti-VEGF agents. To this end, Duan et al. [74] represented the first study to develop a thermosensitive gel injectable via Schiff-based reaction (Figure 3). Sustainable release of ranibizumab, an anti-VEGF antibody, was achieved for more than 7 weeks in a rabbit with persistent RNV. The intravitreal gel also has self-healing properties that not only protect the loaded drug from rapid degradation but also prevent dispersed particles from impeding aqueous humor circulation, minimizing the risk for glaucoma. Moreover, the dynamic, reversible gel-sol conversion allowed for easy clearance of the gel from the syringe.
In a different study, Ilochonwu et al. [75] reported a thermoresponsive Diels-Alder hydrogel that sustainably released a corticosteroid (i.e., dexamethasone) and an anti-VEGF agent for 35 days and 13 days, respectively. Despite the promising findings, the unknown optimal dosing regimen of the injectable warrants additional preclinical and clinical trials.
Prolonged intraocular delivery of triamcinolone acetonide, a corticosteroid, was observed for more than 90 days in vitro by Shen et al. [76]. Several advantages are conferred by this gelatin methacryloyl (GelMA)-based system. First, the eventual shrinkage and complete disappearance of the hydrogel prevents the risk of small fragments from obstructing trabecular meshwork. Second, the absence of sudden burst release minimizes the risk of cytotoxicity caused by the hydrogel. Lastly, the inexpensiveness of gelatin can minimize production costs in large-scale fabrication. However, as many enzymes in the vitreous are responsible for drug degradation, further in vivo tests must be performed to truly gauge the kinetic release profile of the hydrogel.
Age-related macular degeneration
AMD is a progressive, degenerative disease that most commonly affects the Caucasian elderly population [77]. In the early stages of AMD (i.e., dry AMD), drusen deposition, otherwise known as lipid depositions, is detected between the retinal pigment epithelium (RPE) and the Bruch’s membrane [78] (Figure 5). However, in some cases, dry AMD progresses to a more severe form known as wet AMD. A hallmark of wet AMD includes choroidal neovascularization (CNV), which refers to the sprout of new, abnormal blood vessels from the choroid into the subretinal or intraretinal space [79]. Due to the fragility and leakiness of abnormal blood vessels, subretinal fluid can accumulate on the macula, which is responsible for central vision, leading to rapid central vision loss [80]. Although the exact mechanism behind CNV development is not fully understood, it is believed that oxidative stress and inflammation in RPE cells can upregulate the production of VEGF, a potent stimulator of CNV [81, 82]. At present, intravitreal injections of anti-VEGF agents are the backbone of wet AMD treatment [83]. However, one-third of wet AMD patients are unresponsive to mono-VEGF target therapies [84]. Therefore, current research efforts are focusing on developing AMD hydrogel injectables that not only are long-acting but also address wet AMD through multiple pathways. Typically, synthetic hydrogels are most suited for AMD treatments as they can provide long-term, localized delivery of anti-VEGF agents to the retina [85, 86].
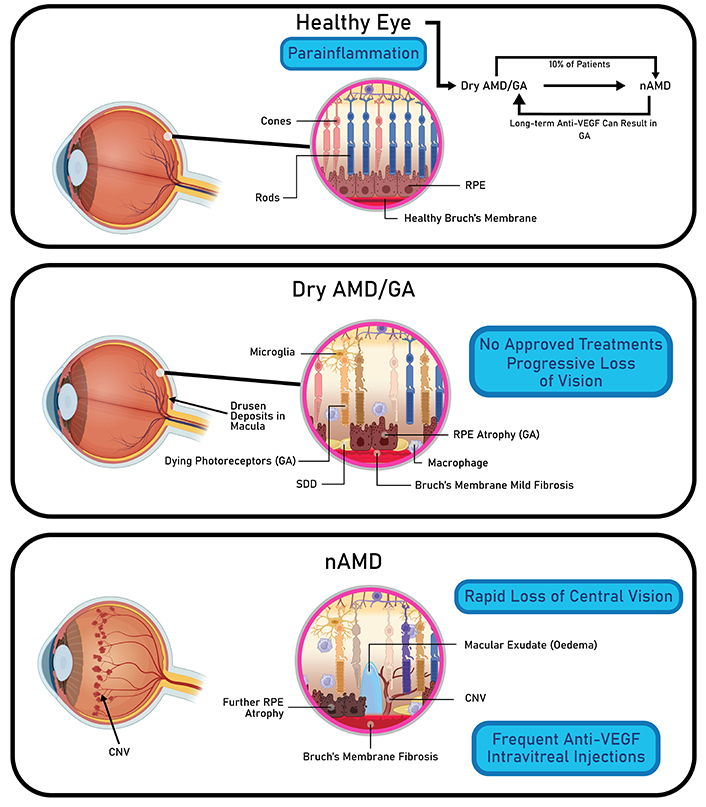
Pathophysiology of different AMD stages. Dry AMD is characterized by drusen deposits in the macula and progressive loss of central vision. Wet AMD is characterized by edema and CNV, leading to rapid loss of central vision. AMD: age-related macular degeneration; nAMD: neovascular AMD; GA: geographic atrophy; VEGF: vascular endothelial growth factor; RPE: retinal pigment epithelium; SDD: subretinal drusenoid deposits; CNV: choroidal neovascularization. Created in BioRender. Nguyen, L. (2024) https://BioRender.com/h87w827
Yaylaci et al. [87] reported the creation of self-assembled hydrogels composed of peptide amphiphile molecules as a carrier for ranibizumab. The innovative system offers several benefits. First, the negatively charged peptide amphiphile molecules naturally self-assembled upon exposure to electrolytes, eliminating the need to use a curing agent. Second, the fibrous nature of the peptide nanofiber hydrogel enabled drug concentrations to stay within the therapeutic range over an extended period of 10–150 hours. Finally, both the diffusion rate of the drug and patient-specific dose adjustment can be easily controlled through a modulation of peptide concentration. Essentially, the system’s tunable physiochemical properties, biodegradability, and biocompatibility render it a promising solution for the long-term treatment of wet AMD in clinical settings.
Gao et al. [88] proposed a hydrogel formulation composed of an anti-VEGF agent, CaCl2, and betamethasone phosphate (BetP), a clinical anti-inflammatory drug. Upon intravitreal delivery, the BetP-gel effectively suppresses both retinal angiogenesis and CNV. Moreover, given that oxidative stress is a key characteristic of wet AMD, the gel can scavenge for free radicals, thereby successfully attenuating inflammation. Compared to that of conventional anti-VEGF therapy, the effective time of anti-VEGF via BetP-gel was three-fold, greatly reducing both the drug dosage and administration frequency. In future studies, the mechanism behind BetP-gel must be elucidated for future clinical applications. In addition, further clinical trials are required to validate the gel’s prolonged therapeutic effects.
Su et al. [89] presented a different formulation that addressed CNV via anti-angiogenic and anti-oxidative stress pathways. Specifically, the combination of thermoresponsive F127 hydrogel and small-size microemulsions was used to deliver the active ingredients, rhein, and baicalein. Together, the reagents work synergistically to enhance cellular uptake and attenuate oxidative stress. Substantial accumulation of the medication was observed in the RPE layer of rats for at least 14 days. Given the ability of the medication to resolve wet AMD via a non-VEGF pathway, the novel system could serve as an effective replacement therapy for wet AMD patients who are unresponsive to anti-VEGF agents.
Challenges in translating hydrogel-based ocular delivery
While significant developments have been made in the field of hydrogel DDS, there are several barriers that impede the transition from research and development into clinical applications.
Biocompatibility & degradation
The absence of long-term biocompatibility and degradation data for hydrogels remains a significant concern. Due to the sensitive nature of ocular environments, it is imperative to verify that both the hydrogel materials and their degradation byproducts are non-toxic and non-inflammatory (Figure 4). Given that many hydrogel formulations are designed for long-term use, thorough and rigorous testing is essential to evaluate their long-term safety and compatibility with biological tissues.
Lack of human clinical trials & regulatory hurdles
Although hydrogels have shown promising results in animal studies, the lack of human clinical trials makes it difficult for hydrogel-based therapies to get approval from the FDA and other regulatory agencies. Due to the increased complexity of human ocular tissues compared to animal models, demonstrating equivalent clinical efficacy in human patients remains a significant challenge (Figure 4).
Challenges in large-scale manufacturing
Ensuring consistency in hydrogel formulations during large-scale production is a significant technical challenge. Due to the customizable nature of hydrogels (Figure 2), even small variations in polymer chemistry, cross-linking, or drug encapsulation can lead to inconsistent drug release kinetics and mechanical properties. Additionally, maintaining sterility during large-scale manufacturing is difficult, as hydrogels may degrade when exposed to heat or radiation. Therefore, alternative sterilization methods may be necessary to preserve the structure and functionality of hydrogels in the production of ocular therapies.
Conclusions
The field of hydrogel-based DDS is rapidly evolving and holds immense potential to overcome the limitations of conventional ocular therapies. Given hydrogels’ unique abilities to bypass key ocular barriers, sustain therapeutic effects, and minimize invasive interventions, increased patient compliance and enhanced treatment outcomes can be expected with this innovative technology. However, as most studies are in the preclinical phase, clinical applications of hydrogels are still in their infancy. To bridge the gap between bench and bedside, rigorous examinations of human models must be conducted to gauge the pharmacokinetic, efficacy, and long-term safety profile of the systems. Moreover, joint efforts and shared expertise between scientists, pharmacologists, and ophthalmologists are needed in the development of safer and more effective hydrogel DDS.
Abbreviations
AMD: | age-related macular degeneration |
β-CD: | β-cyclodextrin |
BetP: | betamethasone phosphate |
BMP4: | bone morphogenetic protein 4 |
BPQD: | black phosphorus quantum dots |
CNV: | choroidal neovascularization |
CoNV: | corneal neovascularization |
DED: | dry eye disease |
DDS: | drug delivery systems |
DNase I: | deoxyribonuclease I |
GSH: | glutathione |
HPMC: | hydroxypropyl methylcellulose |
IMB: | imatinib |
IOP: | intraocular pressure |
NET: | neutrophil extracellular trap |
NO: | nitric oxide |
PEG: | polyethylene glycol |
PHEMA: | poly(hydroxyethyl methacrylate) |
POAG: | primary open-angle glaucoma |
RNV: | retinal neovascularization |
RPE: | retinal pigment epithelium |
silMA: | silk methacrylate |
VEGF: | vascular endothelial growth factor |
Declarations
Author contributions
DT and KYW: Conceptualization, Writing—original draft, Writing—review & editing. LN: Visualization. SDT: Supervision.
Conflicts of interest
The authors declare that they have no conflicts of interest.
Ethical approval
Not applicable.
Consent to participate
Not applicable.
Consent to publication
Not applicable.
Availability of data and materials
Not applicable.
Funding
Not applicable.
Copyright
© The Author(s) 2024.