Abstract
Nanoparticles (NPs) are at the forefront as they are providing unprecedented solutions to obstacles and issues in treating neurodegenerative diseases. Due to their size, surface characteristics, and ability to be functionalized, these carriers can directly deliver therapeutics across what is considered one of the main barriers to central nervous system (CNS) treatment, the blood-brain barrier (BBB). Through nano-technology, anti-disease agents such as Alzheimer’s, Parkinson’s, and Huntington’s therapies become more bioavailable, specific in action, and with fewer side effects. The NPs serve as molecular carriers that facilitate transport across the BBB by receptor-mediated transcytosis or by disruption of the barrier with a view to properly delivering drugs to the neural tissues. Some of the therapeutic applications of nanotechnology also present the concept of molecular medicine since the NPs are designed to deliver drugs in accordance with specific biomolecule signals. Besides the therapeutic applications, NPs replace the traditional contrast media for magnetic resonance imaging (MRI) and positron emission tomography (PET) scans for better diagnosis as well as disease tracking in the early stages. In addition, their effects on solubility increase the therapeutic potential of earlier useless compounds, and the preservation of bioactive molecules from degradation increases the therapeutic capacity of medications. Neurodegenerative disorders are marked by oxidative stress and inflammation that contribute to the disease severity; thus, liposomes, dendrimers, and polymeric NPs encapsulate antioxidants and anti-inflammatory compounds, so they target the areas most affected by the disease. Such sophisticated systems minimize the extension of neuronal deterioration and enhance the lot of such patients. The “theranostic” NPs allow for continuous diagnosis and treatment by containing both diagnostic and therapeutic features. These have created unprecedented opportunities to meet the unmet needs in CNS disorders and may revolutionize the evolution of managing neurodegenerative diseases and innovative neuroimaging procedures in the future.
Keywords
Nanoparticles in medicine, blood-brain barrier, neurodegenerative diseases, nanotechnology-based therapy, bioavailability enhancement & neuroimaging techniquesIntroduction
Nanomaterials are gaining recognition for their transformative potential in treating degenerative intellectual and developmental disabilities (IDDs), such as Alzheimer’s, Parkinson’s, and fragile X syndrome. These disorders, characterized by progressive cognitive, motor, and emotional decline, pose significant treatment challenges, particularly due to the complex nature of brain structures and the blood-brain barrier (BBB), which limits the delivery of therapeutic agents to neural tissues. Nanotechnology offers a promising solution, allowing for precise, targeted delivery of therapeutic agents across the BBB and directly to affected regions in the brain [1]. For instance, nanomaterials like polymeric nanoparticles (NPs) and metal-based NPs have been used in neurodegenerative disease models, demonstrating their capacity to reduce oxidative stress and inflammation two primary drivers of disease progression [2, 3]. Their high surface-area-to-volume ratio and modifiable surface properties make nanomaterials particularly effective at enhancing biocompatibility and providing targeted therapeutic effects, positioning them as essential tools in developing advanced IDD treatments [4].
In recent years, specific nanomaterials such as antioxidants-loaded NPs, phyto-nanotechnology-derived agents, and bioactive 2D nanomaterials have shown potential in mitigating neural damage and supporting the regeneration of brain tissues. These nanomaterials not only offer neuroprotection but also stimulate cellular repair mechanisms, making them suitable candidates for enhancing cognitive and motor functions in IDD patients [5, 6, 7]. Studies also demonstrate the anti-amyloidogenic properties of certain NPs, which can inhibit toxic protein aggregations commonly seen in diseases like Alzheimer’s, further highlighting their therapeutic versatility [8]. Moreover, regenerative nanomedicine is advancing with approaches that incorporate both inorganic and polymer-based NPs, tailored to provide sustained drug release and support long-term neural health [9, 10].
However, while the benefits of nanomaterials in IDD treatments are promising, their use also brings potential challenges. The biocompatibility and long-term safety of these nanomaterials remain critical areas of investigation. Toxicological assessments are necessary to ensure that NPs do not cause adverse effects, particularly in sensitive organs or systems, and that they do not elicit undesirable immune responses [11, 12]. Additionally, future research must explore the specific challenges of using nanomaterials within neurodegenerative contexts, such as optimizing NP structures for safe and effective drug delivery [13]. Innovations in this field are increasingly focused on creating biocompatible nanomaterials that can be tailored to individual genetic and medical profiles, offering a pathway toward personalized treatment strategies [14].
Mental health familiarization defines “degenerative intellectual and developmental diseases” (MDDs) as long-term conditions that affect people’s cognition, emotions, and physical functioning. Some of these impairments may be realized soon after delivery or at any time in early life. Among these, some conditions remain fairly stable over time, while others progressively worsen [15].
Understanding the multifactorial etiopathogenesis of degenerative IDDs, such as Alzheimer’s disease (AD), Rett syndrome, and fragile X syndrome, reveals significant challenges due to the lack of treatments and cures for these diseases [16]. Cognitive impairment, one of the most common issues across various disorders, profoundly impacts the quality of life for individuals and their loved ones. These conditions impair motor, expressive and receptive language, memory, and other cognitive domains. Despite various therapeutic approaches, IDDs remain difficult to manage, with many therapies failing to halt or reverse disease progression. Of all neurodegenerative diseases, AD is perhaps the most well-known, particularly as it affects older adults.
AD has an unclear etiology, and limited treatment options currently exist, which contributes to the difficulty in diagnosing its early symptoms. Recent studies have suggested that the degeneration of the nervous system is linked to an accumulation of amyloid-beta (Aβ) peptides, which cannot be effectively cleared and may be produced in excess. These peptides can self-assemble, forming neurofibrillary plaques, toxic oligomers, and tangles [8].
As the evidence indicates, the accumulation of Aβ peptides leads to cellular damage, which encourages aggregation. Although these aggregates may initially elicit an immune response, they are ultimately toxic to neural connections. Neurofibrillary tangles are further compounded by the malfunction of the tau protein transport system. Reduced tau activity leads to a decrease in essential proteins and minerals, ultimately resulting in the degradation of brain cells. This phenomenon provides a plausible explanation for why plaques and tangles are the most prominent pathological hallmarks in AD, directly contributing to neuronal death and brain tissue atrophy [1]. While considerable evidence supports this model, it remains a topic of ongoing debate. Consequently, the affected brain regions deteriorate through a slow, progressive process [17].
The toxicological and biocompatibility assessments of metal NPs are crucial prerequisites for their use in biomedical applications, particularly in sensitive therapeutic areas such as degenerative IDDs. These evaluations are essential because metal NPs, while offering unique advantages in drug delivery, antioxidant activity, and cellular interactions, may also induce adverse effects that could compromise patient safety and treatment efficacy. For instance, metal NPs like cobalt and silver have demonstrated potential for oxidative stress modulation and immune response enhancement, but they also carry risks related to toxicity and bioaccumulation in vital organs, such as the liver, kidneys, and lungs, as noted by Umar et al. [18] (2024) in their research on cobalt NPs in albino mice. Understanding these interactions and any potential side effects is especially important when considering prolonged exposure or high-dose treatments, which may impact the nervous system or other critical biological functions.
Studies by Khan et al. [19] (2024) have explored the toxicity risks of silver NPs, revealing that even coated NPs designed to improve stability and minimize toxicity can pose risks if not carefully regulated. For example, amine-coated silver NPs were found to influence hematological and antioxidant parameters, emphasizing the need for controlled dosage and tailored surface modifications to avoid unintended cellular or systemic reactions. Such findings underline the complexity of biocompatibility in NP applications and the importance of rigorous preclinical assessments before any biomedical use. Rafique et al. [20] (2021) and Umar et al. [21] (2022) further reinforce the significance of understanding mental health impacts and chronic stress responses, which can be exacerbated by long-term exposure to certain NPs.
Furthermore, to achieve safe and effective biomedical applications, it is imperative to balance the therapeutic potential of metal NPs with their biocompatibility. Advanced nanomaterials like cobalt carbonate and silver carbonate NPs, as explored by Umar et al. [22] (2024), demonstrate therapeutic promise in wound healing and antimicrobial applications. However, achieving this balance requires comprehensive toxicological profiling, including studies on bioaccumulation, systemic toxicity, and potential immunological impacts. This rigorous approach ensures that metal NPs can be integrated into medical therapies without compromising patient health. Thus, before incorporating metal NPs into treatments for IDDs or similar applications, a thorough evaluation of their toxicological profile and biocompatibility is not only advisable but essential to harnessing their benefits safely in clinical settings.
The illustration in Figure 1, represents the BBB and the following parts of it: endothelial cells, tight junctions, the basement membrane, astrocyte end-feet, and neurons. The BBB is an important gatekeeper that separates the brain microenvironment from the bloodstream and regulates the entry or exclusion of molecules, maintaining homeostasis within the neural ecosystem while excluding material that would be toxic to the brain. Pericytes and astrocytes are involved with the maintenance of the BBB as well as the appropriate signaling between the blood and the surrounding brain cells. This was a complicated network that was critical in maintaining the overall health of the brain and moderate in regulating the surrounding environment.
The purpose of this section is to highlight some episodic changes associated with AD to define its various stages. Research has shown that antibody-induced mitochondrial disruption, resulting from unconventional reactive oxygen species (ROS) generation, is a major contributor to the disease [1]. Despite numerous bioactive compounds already evaluated in clinical trials, the application of targeted drugs to manage AD remains a promising area, as these compounds have shown potential but are yet to be fully utilized in therapeutic industries [17].
One primary challenge in treatment is the difficulty of delivering drugs across the BBB, a complex molecular structure composed of specialized capillary endothelial cells with unique features like tight junctions and limited transport pathways, which restricts drug delivery [4]. This barrier is characterized by a limited number of transport avenues and closed cell junctions, making it highly selective. Treatment reduces the frequency of pinocytosis and the number of intracellular fenestrae in these endothelial cells [15].
The brain’s endothelial cells express various efflux transport proteins, such as P-glycoprotein, multidrug resistance protein, and breast cancer resistance protein, which further restrict drug penetration into the brain [15]. To address this challenge and improve the targeted delivery of drugs across the BBB, NPs have been developed as promising vehicles. These NPs exhibit the potential for selective biodistribution, avoiding phagocytic cells, as evidenced by findings that show specialized cells, such as Kupffer cells, can filter smaller particles [23].
NPs’ prolonged circulation in the bloodstream enhances the likelihood of steady drug delivery to the BBB, increasing the concentration gradient across capillary walls and thus facilitating NP transport across endothelial cells [2]. Additionally, the lipid solubilization ability of certain drugs facilitates their passage through the BBB, keeping endothelial layers fluid and modulating tight junction interactions among cells. As a result, these particles increase sedative uptake via phagocytosis, carrier-mediated transport, transcytosis, and endocytosis, ultimately releasing the drug into the brain’s endothelial cells [24].
Effective surface functionalization is crucial to NP efficacy. Techniques like polyethylene glycol (PEG) coating, transferrin coating, and amine coating enhance the particles’ performance, while architectures such as cytotoxic fullerenol conjugates, conjugated polymers, and chitosan improve drug targeting [5]. Innovations like LipoBridge help open the BBB’s tight cross-sections temporarily, and advancements such as angiopep-based peptide carriers facilitate therapeutic delivery [25].
Previous studies have demonstrated the efficacy of cerium oxide (CeO2) NPs in ROS removal through Ce3+ and Ce4+ oxidation states in transgenic mice, aiming to increase public awareness of these advancements [26]. Triphenylphosphonium is another example, targeting mitochondria to enable focused delivery of NPs across the BBB, a significant barrier in treating neurological diseases, including IDD [27].
Recently, the BBB has been recognized as an effective barrier, excluding hazardous substances and therapeutic molecules alike from reaching intracranial targets. Nanotechnology now offers potential solutions, such as NPs, nanofibers, and nanocomposites, which represent a new class of materials capable of addressing this barrier [28]. Thus, NPs (owing to their small size and adjustable surface properties) can cross the BBB through passive or receptor-mediated processes, making them ideal candidates for delivering targeted sedatives that reach neurons and other brain cells. Their nanoscale nature allows them to be integrated into pharmaceuticals, improving bioavailability, minimizing systemic side effects, and reducing necessary dosages.
Nanoparticles for drug delivery in IDDs
Even though the potential of NPs has long been underestimated, it is now widely recognized that they represent one of the most effective methods for delivering therapeutic medications directly to brain tissue. This efficacy is largely due to their unique ability to cross the BBB, a major obstacle in neuropharmacology. Among the various types of NPs, liposomes are especially prominent in applications requiring targeted delivery to the brain. This approach leverages nanomaterials for enhanced drug delivery systems [24]. In 2023, it is highlighted the potential of liposomal NPs to provide neuroprotective interventions for AD patients. These treatments show promise not only for improving cognitive function but also for reducing neurodegeneration, offering a dual benefit in Alzheimer’s care [8].
In Figure 2, nanomaterials usage for neurological disorders has focused on spinal cord injury, Huntington’s disease, AD, Parkinson’s disease, amyotrophic lateral sclerosis (ALS), and conditions such as stroke and tumors in the brain. These nanomaterials are indispensable in augmenting methods of treatment and delivering them as well as increasing efficiency in the management of complicated neurological disorders. These features provide considerable possibilities for future progress in the neurosciences and neuro therapeutics.
In tolerable daily intake (TDI) therapies, research has demonstrated that NPs are versatile enough to act as carriers for a variety of therapeutic treatments. This versatility is one reason why companies in this field are successful, as they are capable of synthesizing NPs that deliver both hydrophilic and hydrophobic drugs effectively. One notable achievement is the use of polymeric NPs, which have proven to be a reliable method for delivering targeted therapies for genetic defects, such as those associated with birth conditions like fragile X syndrome. These NPs have been beneficial in a wide range of applications, reflecting the extensive research and development invested in their advancement [29].
Polymeric NPs have evolved significantly since their initial creation as delivery systems, and recent innovations continue to expand their potential. For instance, a study by Zhou et al. [30] utilized CRISPR/Cas9-loaded polymer NPs in a Delicate X mouse model, successfully correcting genetic mutations. This breakthrough led to further positive effects, including improved synaptic function and enhanced learning and memory capabilities, highlighting the potential of NPs in addressing complex neurological disorders [11].
Nanomaterials for neuro-regeneration
The synthesis of nanofibers and other nanomaterials has been aimed at facilitating nerve regeneration in patients with degenerative inflammatory bowel disease (IBD). In 2023, the same author and his team further demonstrated that graphene oxide nanofibers could stimulate neuron regrowth in a Rett disease model. In their study, these nanofibers acted as a scaffold, enabling neural stem cells to multiply and thrive, thereby enhancing the organism’s motor and cognitive abilities [23]. With advancements in understanding the cellular and molecular dynamics of the nervous system, researchers have identified new pathways and tools involved in neurodegeneration. However, significant challenges remain in managing neuro-degenerative disorders, particularly due to the ontopathogenic complexities of the nervous system. Addressing neuroinfectious disorders, such as brain tumors and neurodegenerative diseases, is challenging, as the body’s defense mechanisms—including the blood-cerebrospinal fluid barrier and the BBB serve as formidable obstacles against foreign agents that could harm the neurological system [31]. These barriers represent critical points of defense that complicate treatment delivery. Furthermore, the appropriate application of nanomaterials and regeneration techniques is essential, as highlighted by the insights gained from both preclinical and clinical research [32].
Figure 3 shows that the technology of nano therapy has multiple uses in medicine, particularly in delivering drugs, protecting neurons, and in other therapeutic developments. The emerging areas include nano-carriers, such as ‘Nanomicelles’ for specific drug delivery; another field noticed is ‘Personalized Medicine’ for treatment with specific precision; and lastly, solutions for perinatal and neurological disorders. For instance, Nano Perfection and Beib obstacle are displayed as products that eliminate naturally set biological obstacles for domestically located microbes, indicating the application of nanotechnology in biomedical engineering with tremendous possibilities besides amplifying the bringing into effect of remedial solutions.
An example of such combinational techniques, driven by necessity, is the application of kinetically favorable methods alongside other approaches such as photothermal, photodynamic, and chemoprevention techniques. This combinatorial approach has proven to be notably effective, offering enhanced outcomes compared to standalone interventions. Such an integrated framework leverages the advantages of multiple methods, providing a more comprehensive therapeutic solution. However, treatments like benzodiazepines have shown limited effectiveness for minor conditions such as enuresis, lock-in syndrome, and certain neuromuscular injuries, where sedatives are generally ineffective [33].
One particularly intriguing approach is the brain-computer interface (BCI), which enables clinicians to access and interpret physiological and cognitive processes in real-time. Interpersonal Communication Boards (ICBs) within BCIs allow for a more nuanced understanding of patient brain activity, facilitating insights into mental and physiological states. These ICBs use varied techniques for detecting, assessing, and managing brain impulses, with specific gadgets designed to serve targeted functions. Nevertheless, the field of ICB technology faces several barriers, including policy regulations, sensor limitations, user accessibility, legal and ethical concerns, as well as challenges related to security and privacy protections [14].
Figure 4 shows a more organized plan for developing nano-enabled therapies by beginning with collaborations to increase research and trials. It advances through ethical system studies with mice, employs the use of AI to enhance therapeutic use, and it undertakes rigorous risk analyses. Lastly, it aspires to create novel nanostructures for therapeutics that are also multipurpose and responsive to stimuli.
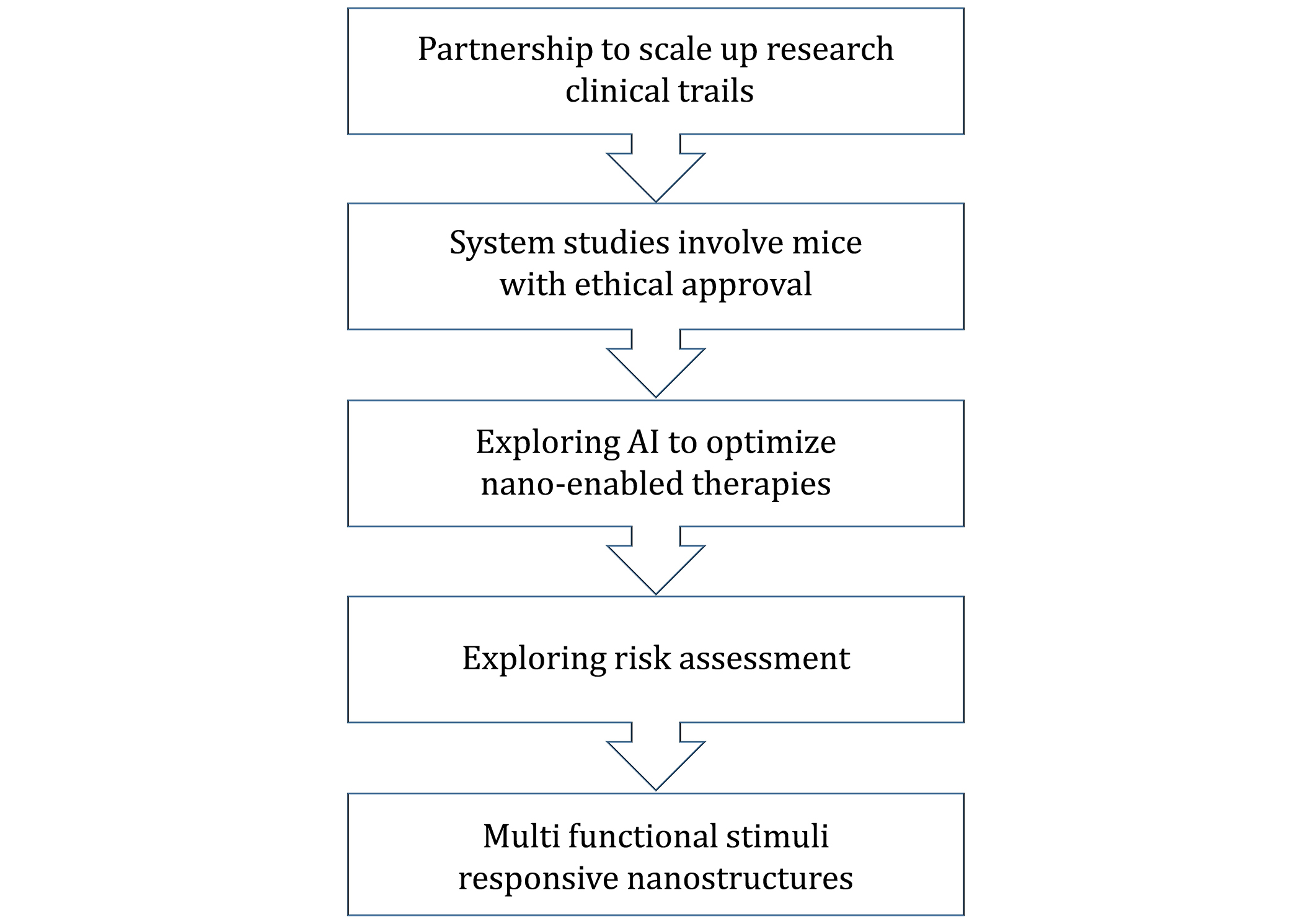
Detailed introduction of the scaling up of research from the nano level as well as ethical studies, AI optimization, risk assessment, and optimization of multifunctional nanostructures
In recent years, gold NPs (AuNPs) have garnered significant attention across various fields, largely due to their antibacterial properties and potential applications in nerve regeneration. These NPs are promising for the treatment of several nervous system disorders and injuries, thanks to their anti-inflammatory and antioxidant effects [5]. In an experiment demonstrating that AuNPs functionalized with neurotrophic factors (AuNPs-NTFs) could support neuronal survival in mice with traumatic brain injury (TBI). This study highlighted the capacity of these functionalized NPs to reduce oxidative stress, a factor closely linked to cell migration and recovery following neurological trauma or infection [5].
The processes involved in TBI such as injury exacerbation, oxidative stress, and cell death mirror mechanisms seen in degenerative disc disease (IDD), suggesting that similar interventions could apply. The findings revealed that neurotropic AuNPs serve as both anti-inflammatory agents and stimulators of nervous tissue regeneration, enhancing cellular signaling pathways essential for survival and tissue remodeling. These NPs provide cellular protection against oxidation, a key factor in slowing dementia progression, as evidenced by decreased ROS levels in injured tissue [4].
Beyond AuNPs-based medication synthesis, this research underscores the potential for developing treatments for neurodegenerative diseases like Parkinson’s, Alzheimer’s, and other IDDs. Due to their modifiable surface properties, AuNPs can be adapted for specific therapeutic functions, making them versatile tools in rehabilitation medicine with applications in treating various degenerative diseases [23].
Challenges and future directions
While NPs show significant potential in the therapeutic management of IDDs, their precise role and mechanisms within these applications remain to be fully understood. Moreover, there are numerous challenges and critical factors that require careful consideration. Due to their nanoscale size and unique properties, NPs interact with biological systems in distinct ways, which can introduce risks not seen with other therapeutic approaches. The potential for bioaccumulation in vital organs, such as the kidneys, liver, and lungs, raises concerns about long-term safety and necessitates further exploration [9].
Additionally, certain nanomaterials can trigger immunological responses. While these responses may sometimes provide protective effects, they can also cause irritation in individuals involved in the development and application of these materials, potentially worsening conditions rather than alleviating them. This calls for more research into the impact of nanomaterials on the immune system prior to their widespread application in IDDs [3]. Regarding the long-term stability of nanomaterials, safety remains a crucial question, as the potential short-term advantages may be outweighed by unknown long-term effects. It is essential to assess and understand the implications of delayed or prolonged exposure to nanomaterials [4].
To better understand the body’s adaptation to nanomaterials, further toxicological and pharmacological studies are necessary, especially those focusing on bioaccumulation and secondary effects. Given that IDDs are complex and heterogeneous disorders, a single nanomaterial approach may not suffice for optimal treatment. Instead, combining nanomaterials with other therapeutic methods (such as pharmacological, behavioral, physical, and occupational therapies) could offer a more comprehensive strategy for managing diverse symptoms and challenges associated with IDDs. This combined approach may yield benefits in areas like pain relief, functional restoration, psychological support, and inflammation prevention [34]. Given the complexity and multidisciplinary needs of IDDs, relying solely on nanomaterials may be insufficient for achieving the best rehabilitation outcomes. IDDs often involve mental and social challenges alongside physiological issues, and a multi-faceted approach is therefore necessary to address the full spectrum of symptoms effectively [35].
Figure 5 shows a contrast between the control oral insulin delivery method and the third-party chitosan insulin NPs designed to improve insulin uptake and control glucose levels. During conventional delivery, insulin comes across the highly acidic area of the stomach (pH 1.2) and gets attacked by enzymes, which results in less absorption of insulin in the intestines (pH 7.4) and hence the slight decrease in blood glucose level. On the other hand, chitosan insulin NPs offer a shield against enzymatic degradation in the stomach, and the insulin reaching the intestine is actually intact. This leads to enhanced insulin uptake and blood glucose reduction where the therapeutic effectiveness of chitosan formulations for delivery systems is apparent.
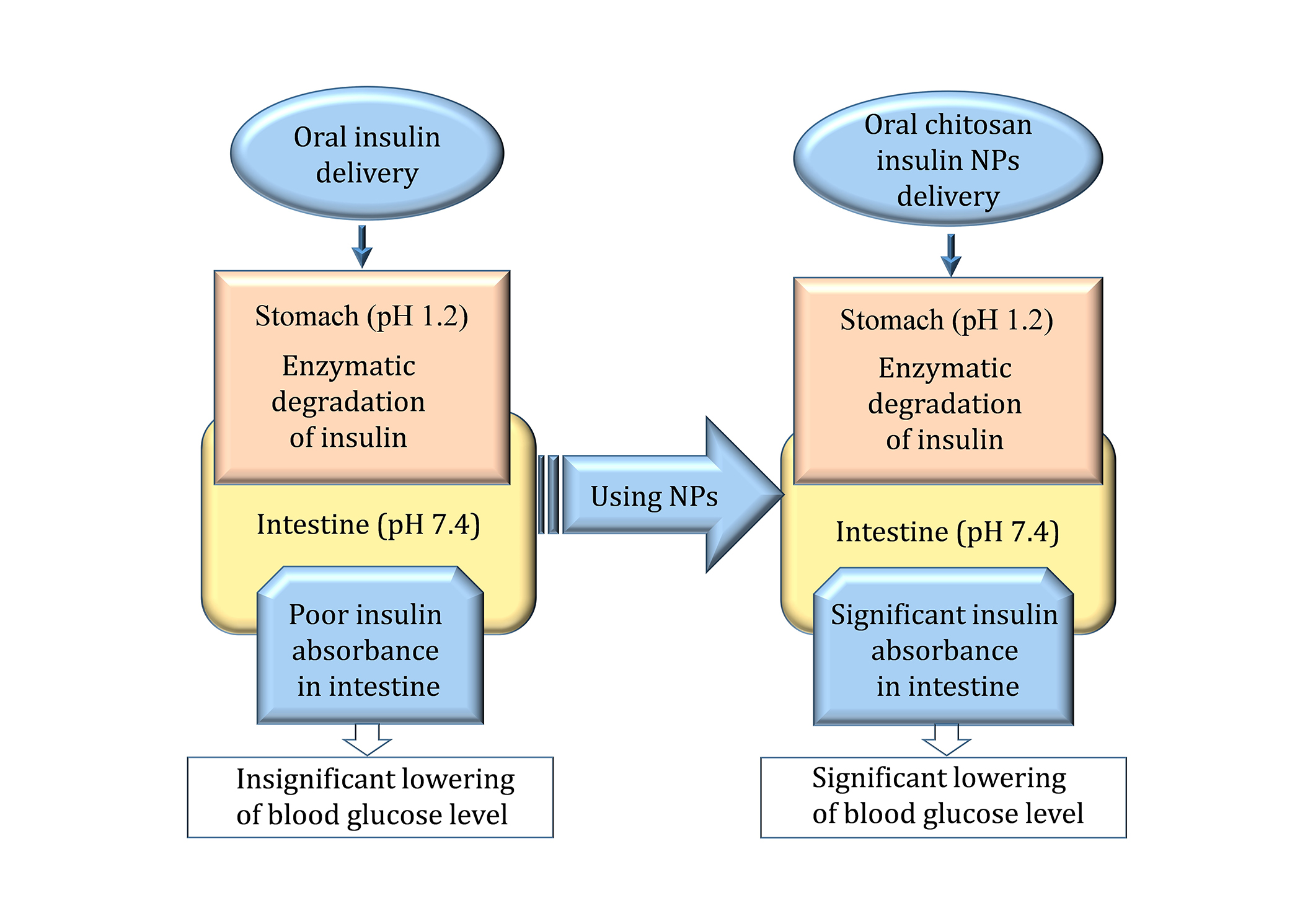
Nanoparticles increase the loading efficiency, protectiveness, and biological availability of insulin in treatment procedures. NPs: nanoparticles
The supplementary use of nanomaterial-based therapies alongside conventional treatments, such as behavioral therapy, physical therapy, and medications, offers promising benefits for patients with IDDs. A synergistic combination of these rehabilitation approaches may assist in managing aspects of IDD, including anxiety, acute pain, physical limitations, and psychological well-being [36].
Future research should focus on enhancing nanomaterials to improve their regenerative properties and biocompatibility. This may involve modifying the surface characteristics of NPs to ensure better compatibility with native tissues, minimizing any potential adverse effects. Additionally, the standardization of methodologies is necessary to ensure that NPs integrated into existing therapeutic approaches are optimized for safety and efficacy, complementing rather than disrupting established treatment processes [32].
Innovations in targeted nanomedicine could potentially address conditions like intervertebral disc degeneration, a type of IDD. Advances in personalized medicine may lead to the development of nanomaterials tailored to an individual’s medical history, genetic profile, and treatment needs. The use of nanotherapeutics represents a significant breakthrough in the management of complex conditions like IDD, as these therapies can be customized for each patient. With this approach, nanomaterials hold the potential to become precise and adaptable tools, offering targeted solutions for complex disorders rather than relying on generalized treatments [7, 37].
In one below Figure 6, nanomedicine has attendant issues such as low therapeutic response proportionally to the BBB, which poses a challenge for the drug delivery system; nanotoxicity by aggregation of NPs in the brain and the body; a relative issue in scaling up of nanomedicine production due to the high costs involved and the present lengthy approval procedures and lack of sufficient safety data for use in the long term. Regarding the aforementioned ones, the future directions encompass the development of nanomedicine that is specifically designed according to individual patient profiles, improved drug delivery systems to cross the BBB with better accuracy, safer biodegradable NPs to replace current toxic ones, and integration of AI and machine learning to utilize NP design to their maximum effect and safety.
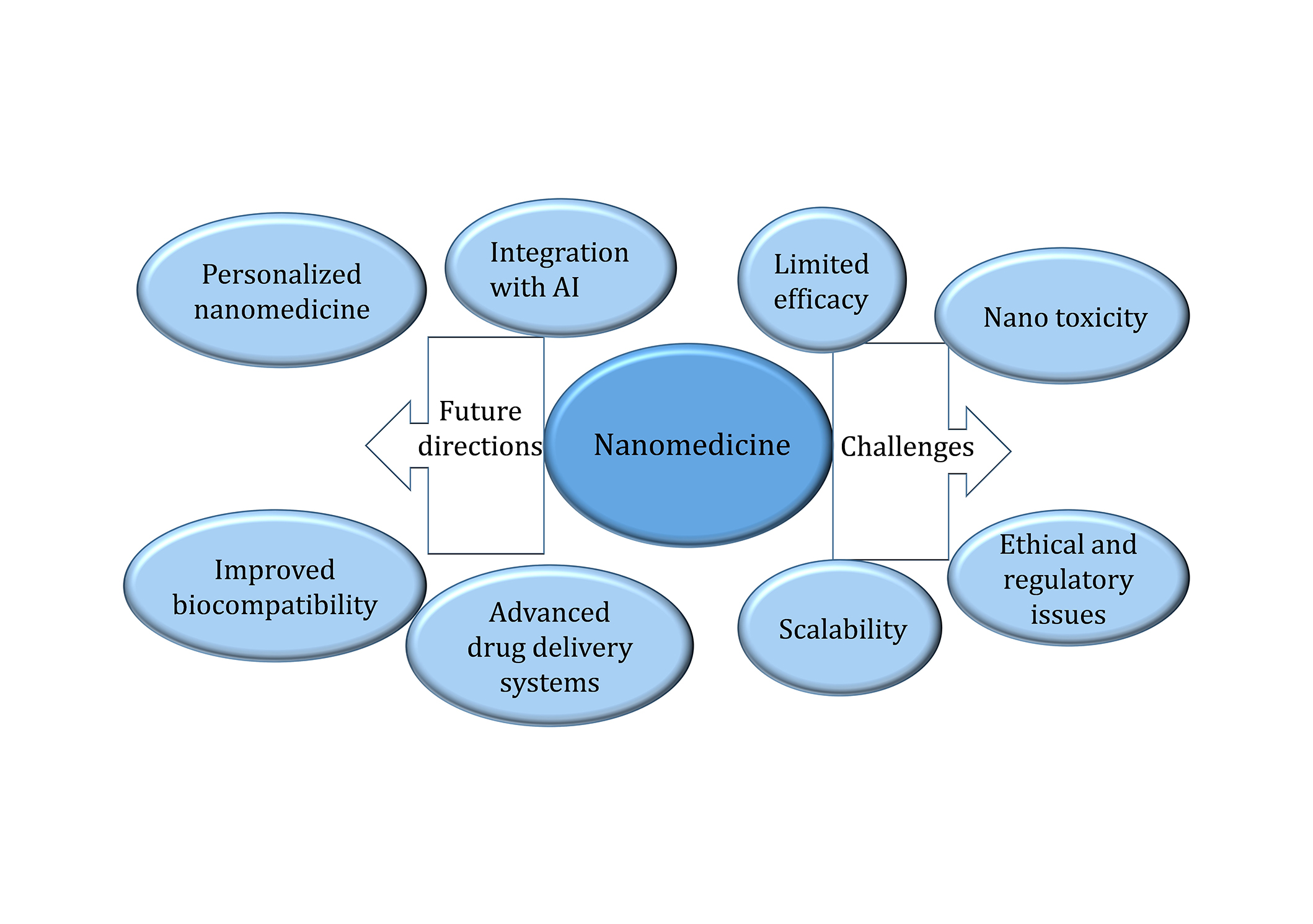
Nanomedicine: present state and development trend with future perspectives and problems
Table 1 displays data regarding various dosage levels as well as the routes of direction and time of application of different nanomaterials. X-ray was performed simultaneously with intravaginal of cobalt NPs (CoNPs) or silver NPs (AgNPs) at 0.1 to 100 mg/kg. Such can further be said for exposure durations, with some studies lasting even several weeks. For instance, polymeric NPs like PLGA and CeO2, with antioxidant-loaded were used for several weeks to months, while T-LP and AuNPs were expected to last shorter durations. As for most of the experiments, single or multiple injections of CNTs have been done over several weeks, while within days and weeks, bioactive 2D nanomaterials such as graphene oxides were used. This diverse range speaks to the multitude of experimental protocols designed to evaluate the toxicity, biological activity, and therapeutic efficiency of the nanomaterials.
Summary of doses, routes of exposure, and duration for nanomaterials
Nanomaterial | Dose | Route of exposure | Duration | References |
---|---|---|---|---|
Cobalt nanoparticles (CoNPs) | 1–100 mg/kg | Intravenous (IV) | Days to several weeks | [22] |
Silver nanoparticles (AgNPs) | 0.1–10 mg/kg | IV or intranasal | Days to weeks | [18, 38] |
Polymeric nanoparticles (e.g., PLGA) | 10–50 mg/kg | IV or intranasal | Weeks to months | [14, 26] |
Gold nanoparticles (AuNPs) | 0.5–5 mg/kg | IV or oral | Days to several weeks | [4, 5] |
Antioxidant-loaded nanoparticles (e.g., cerium oxide NPs) | 1–10 mg/kg | IV or intranasal | Several weeks | [3, 39] |
Phyto-nanoparticles (plant-derived) | 5–20 mg/kg | Oral or IV | Days to weeks | [6] |
Carbon nanotubes (CNTs) | < 1 mg/kg | Intranasal or IV | Single dose to multiple doses over weeks | [35, 40] |
Bioactive 2D nanomaterials (e.g., graphene oxide) | 1–5 mg/kg | Intranasal or IV | Several days to months | [7, 32] |
PLGA: poly lactic-co-glycolic acid
Conclusions
Nanomaterials bring refreshing approaches to addressing some issues related to IDD, such as low therapeutic bioavailability and the inability to cross the BBB in treating developmental and degenerative psychiatric disorders. By increasing medication delivery, decreasing the time it takes for drugs to clear the body, and increasing early imaging options through improved neuroimaging techniques, what they offer is a lever to effectively navigate the intricate landscape of IDD. However, despite such advancements, great challenges are still evident that hinder the possibility of using nanotechnology in therapeutic applications. For more about the chronic effects, side effects, and non-happily endings of nanomaterials, further studies and clinical trials were called for. Furthermore, the application of nanotechnology in delivering transdermal medication calls for heightened biocompatibility as well as tailored screening techniques for every patient in the population. In conclusion, it can be concluded that despite the possibilities of turning around the IDD therapy fully, nanomaterials’ examination and utilization must be further pursued in-depth, useful, safe, and standardized in health facilities.
Abbreviations
AD: | Alzheimer’s disease |
AgNPs: | silver nanoparticles |
AuNPs: | gold nanoparticles |
Aβ: | amyloid-beta |
BBB: | blood-brain barrier |
BCI: | brain-computer interface |
CeO2: | cerium dioxide |
CNS: | central nervous system |
ICBs: | Interpersonal Communication Boards |
IDDs: | intellectual and developmental disorders |
ROS: | reactive oxygen species |
TBI: | traumatic brain injury |
Declarations
Author contributions
HA: Investigation, Writing—original draft. A Umar: Conceptualization, Supervision, Writing—review & editing. NN: Data curation, Methodology. MM: Investigation. A Ullah: Formal analysis, Resources. SH: Investigation, Data curation. MJS: Software, Visualization. MA and MWA: Writing—review & editing. MUK: Conceptualization, Supervision.
Conflicts of interest
The authors declare that they have no conflicts of interest.
Ethical approval
Not applicable.
Consent to participate
Not applicable.
Consent to publication
Not applicable.
Availability of data and materials
Not applicable.
Funding
Not applicable.
Copyright
© The Author(s) 2024.