Abstract
Drawing insights from a spectrum of in vitro, in vivo experimental, and clinical studies, this review illuminates the underlying mechanism by which iodinated contrast media (ICM) exerts an indirect genotoxic effect. The mechanism involves the photoelectric effect induced by iodine molecules, thereby augmenting radiation attenuation and subsequently elevating the locally absorbed radiation dose. The ensuing generation of secondary electrons from each photoelectric absorption interaction triggers molecular reactions, culminating in discernible DNA damage, notably in the form of DNA double-strand breaks. A convergence of evidence from in vitro, experimental, and clinical investigations underscores a consistent pattern: the addition of iodine contrast linearly heightens the absorbed radiation dose and associated DNA damage. This quantification was evident through alterations in attenuation and the manifestation of double-strand breaks in circulating lymphocytes, serving as an intermediate endpoint and a potential long-term indicator of cancer. The observed surplus of DNA damage in contrast-enhanced images compared to non-contrast images ranged notably from +30% to +200%. This broad range accentuates a substantial amplification effect on radiation-induced damage, particularly noteworthy at clinically relevant iodine doses. Crucially, this effect remains unaffected by brands or manufacturers and exhibits a robust, exclusive correlation with the concentration of iodine in the bloodstream. The significant augmentation of absorbed dose and genotoxic impact of X-rays due to the use of contrast agents warrants critical attention within the medical community. This often-unacknowledged genotoxic influence may play a pivotal role in elevating cancer risks among patients undergoing radiation-based procedures, necessitating a reconsideration of risk assessment protocols and clinical practices.
Keywords
Cancer, DNA damage, iodine, radiationIntroduction
The reliance on iodinated contrast media (ICM) for accurate diagnostic imaging is a crucial aspect of contemporary cardiology practice. Based on the estimates of the National Council of Radiation Protection in the United States, in the radiologic year 2016, there were 8,100,000 interventional fluoroscopy procedures including cardiac and non-cardiac procedures, 13,027,708 computed tomography (CT) angiography (non-cardiac), 281,920 cardiac CT, and 13,250,657 chest CT scans [1].
Despite their benefits, the use of ICM carries recognized drawbacks, where side effects can manifest within moments or minutes post-injection, such as hypersensitivity reactions, or emerge within days or weeks, like contrast-induced nephropathy [2]. These complications, while well-documented and associated with high medico-legal liability, can be mitigated through preventive and therapeutic measures.
However, a third adverse consequence linked to ICM usage is less acknowledged, stemming from increased radiation absorption and amplified DNA damage, potentially leading to heightened cancer risks in the long term [3].
Central to sound radiology and cardiology practice is the practice of good radiological standards, especially given that cardiologists either prescribe or conduct 30% to 50% of all radiological examinations [4]. This review aims to present comprehensive evidence from in vitro, in vivo experimental, and clinical studies linking ICM use to heightened radiation-induced damage. Enhancing awareness regarding this correlation is crucial, forming the basis for a strategy focused on justifying and optimizing ICM use. This strategic approach aims to bolster radioprotection for patients, thereby minimizing the long-term cancer risks associated with interventional procedures and computed tomography involving contrast agents.
Physical basis: the photoelectric effect
The well-established evidence highlighting the genotoxic impact of ICM stems from the photoelectric effect on iodine molecules. ICM is specifically designed to heighten the absorption of X-ray photons. For each radiation energy delivered, the radiation dose absorbed by tissues increases linearly with the increase in tissue iodine concentration [5–7]. Each interaction via photoelectric absorption generates secondary electrons that interact with surrounding molecules, notably DNA, resulting in discernible DNA damage, specifically in the form of DNA double-strand breaks. These breaks are recognized as a risk factor contributing to subsequent cancer development. The entity of genotoxic damage is directly and tightly correlated to iodine concentration in tissues, and independent from the type of contrast, whether first-generation (ionic high-osmolar), second-generation (non-ionic low-osmolar), and third-generation (iso-osmolar). ICM can be degraded due to radiolysis at clinically relevant energies. Generated free ions rapidly associate with potassium or sodium to generate sodium or potassium iodide, which enters the cell cytoplasm and nucleus to interact with DNA, producing direct damage and mainly decreasing the activity of DNA-dependent protein kinase, with a reduction of DNA damage repair capacity [8]. Increased energy deposition is likely to occur within very close proximity to ICM molecules (within a few tens of micrometers) [5].
In vitro studies in cells
DNA damage resulting from ionizing radiation encompasses various forms, including base and sugar damage, single-strand breaks, and double-strand breaks. Among these, double-strand breaks are particularly challenging to repair and hold a significant risk for genomic instability and chromosome aberrations, which can be passed on to subsequent cellular generations. Hence, they serve as a robust biomarker of DNA damage and a long-term indicator of cancer risk, given their potential to produce oncogenic rearrangements during faulty repair processes.
To evaluate DNA damage induced by radiation, the most sensitive method for the quantification of radiation-induced DNA double-strand breaks in blood lymphocytes is phosphorylated histone H2AX (γ-H2AX) immunofluorescence microscopy. It measures the formation of γ-H2AX foci, which serve as markers for double-strand breaks. While the contrast medium itself did not exhibit significant effects in generating chromosomal aberrations, when combined with X-rays, it increased the yield of radiation-induced chromosomal aberrations, an effect dependent on the iodine concentration, as shown by several in vitro studies summarized in Table 1 [9–21]. With ICM, X-rays are damaging to cell DNA, but gamma rays are not [13]. The damage is highest immediately after exposure, but decreases progressively in the following 24 h due to the DNA repair mechanism, with a return to baseline levels after 24 h [17]. The entity of damage is proportional to iodine concentration and independent of the type of ICM [19]. ICM has some possible genotoxic effects, amplified (doubled) by radiation exposure. In some studies, there was a direct genotoxic effect of ICM, attributed to iomeprol radiolysis that inhibits double-strand repair rate by decreasing DNA-PK kinase activity [14]. Studies were mostly focused on lymphocytes, but similar effects were found with other cells such as fibroblasts [16]. A summary of the primary conclusions derived from 25 years of in vitro experiments and relevant to the practice of the cardiologist is presented in Table 2.
The in vitro studies
Author, year | Cell type | X-rays dose (mGy) | Equipment | ICM type | Iodine dose (mg I/mL) | Results | Reference |
---|---|---|---|---|---|---|---|
Schmid and Bauchinger, 1976 | Chromosome dicentrics in Chinese hamster cells | NA | NA | Joduron | NA | Increased chromosomal damage | [9] |
Norman et al., 1978 | Lymphocyte micronuclei and chromosome aberrations | NA | NA | Diatrizoate, triiodobenzoic acid | - | Increased chromosomal damage | [10] |
Callisen et al., 1979 | Lymphocytesurvival | 30–120 | Faxitron | NA | 3.7–18.5 | +270% | [11] |
Hadnagy et al., 1982 | Lymphocyte dicentrics | 500–1,880 | NA | Sodium-meglumin-joxaglat | 5.6–19.2 | +160% | [12] |
Matsubara et al.,1997 | Lymphocyte micronuclei and chromosome aberrations | 500–4,000 | 137Cs gamma rays | Unspecified | - | No effect in gamma-irradiated cells | [13] |
Joubert et al., 2005 | Endothelial cells | - | CT scanner | Iomeprol | - | - | [14] |
Jost et al., 2009 | Lymphocyteγ-H2AX foci | 0–1,000 | Siemens Sensation 64 (CT) | Iotrolan | 5–50 | +149% | [15] |
Grudzenski et al., 2009 | Lymphocyteγ-H2AX foci | 10–500 | Philips PW2148 (radiography tube) | Iopromide | 33 | +60–250% | [16] |
Fibroblast γ-H2AX | 1,500 | Philips PW2148 (radiography tube) | Iomeprol | 33 | +50% (31–75) | [16] | |
Pathe et al., 2011 | Lymphocyteγ-H2AX foci | 0–1,000 | Siemens MultixM (radiography) | Iopromide | 37 | +58% | [17] |
Beels et al., 2012 | Lymphocyteγ-H2AX foci | 5–50 | Philips MG420 generator | NA | 5–20 | No net effect | [18] |
Deinzer et al., 2014 | Lymphocyteγ-H2AX foci | 20–500 | Siemens Axiom Multix MT | Iopromid, iodixanol, iomeprol, iopamidol | 7.5–15 | +40% | [19] |
Gould et al., 2016 | Lymphocyteγ-H2AX foci | 70–450 | Carestream DRX-Evolution (radiograph) | Ioexol | 15–52.5 | +11–111% | [20] |
Wang et al., 2017 | Lymphocyteγ-H2AX foci | 33 | Siemens Somatom definition flash, CT dual source | Iopromide | NA | +83% of ICM alone; +350% with CT scan | [21] |
NA: not available; -: blank cell
Main results of in vitro studies linking ICM with X-rays to DNA damage
Experimental model | Effect |
---|---|
Cell types (lymphocytes) | DNA damage increased from 30% to 200% |
ICM without X-rays | No effect |
ICM added after X-rays | No effect |
ICM pre-irradiated before mixing with blood | No effect |
ICM irradiated with gamma rays | No effect |
Increasing concentrations of iodine | Linear dose-dependent effect |
Different ICM brands or manufacturers | No difference (only iodine concentration counts) |
Studies in the experimental animal
In a laboratory animal investigation, minipigs underwent exposure to a cardiac CT scan protocol, with and without the administration of iodixanol (Visipaque), ranging from 0 mg I/mL to 50 mg I/mL concentrations [22]. The extent of DNA damage was evaluated using the standard γ-H2AX immunofluorescent staining of blood lymphocytes. A noticeable increase in DNA damage was observed in the exposed group compared to the unexposed group. The magnitude of damage at the same radiation dose exhibited a direct correlation with the blood iodine dose. Specifically, there was a 56.1% increase for the reduced iodine dose (160 mg I/mL) and a 141.1% increase for the standard iodine dose (320 mg I/mL) protocols.
These in vivo findings were substantiated by a dosimetry simulation model, revealing 78.8% and 133.7% increases in locally absorbed blood dose in the left ventricle for the reduced and standard iodine dose protocols, respectively. These results align with in vitro investigations, with almost all studies (excluding one reference) demonstrating the amplifying effects of ICM on DNA damage following exposure to X-ray radiation.
The significance of these data is underscored by the use of a cardiac scanner (Revolution CT, GE Healthcare, 256 slice) and the administration of ICM at clinically relevant doses. The experimental design meticulously controlled for variables that could act as confounders in clinical studies, including the patient’s medical history, irradiated blood volume, iodine concentration in the exposed blood volume, patient physiology, and inter-individual differences in DNA repair capabilities.
Clinical studies
Early studies started in the seventies based on chromosome aberrations during angiocardiography and urography, showed increased DNA damage detected as chromosome aberrations and micronuclei in circulating lymphocytes at a much greater rate than expected based on radiation exposure alone [23–27]. These clinical studies have demonstrated that the use of ICM leads to an escalation in the absorbed radiation dose and correlates with an elevated level of DNA damage, as evidenced by an increase in γ-H2AX foci count in patients undergoing ICM-enhanced chest or coronary or abdominal computed tomography scans [16, 17, 20, 27–29]. The main study findings and methodology are summarized in Table 3.
In vivo human studies using lymphocyte γ-H2AX foci as the assay type
Author, year | N (with and without ICM) | Equipment | Dose | ICM type | Iodine dose administered (g) | Results | Reference |
---|---|---|---|---|---|---|---|
Grudzenski et al., 2009 | 19/18 | Philips MX 8000 CT | 287/480 mGy*cm2 | Iopromide | 34.9 | +30% | [16] |
Pathe et al., 2011 | 15/15 | Siemens Somaton 10 or 64 | 336–392 mGy*cm2 | Iopromide | 37–44.4 | +58% | [17] |
Gould et al., 2016 | 57/12 | Philips Allura Xper (fluoroscopy) | 116 mGy*cm2 | Iomeprol | 19 | - | [20] |
Piechowiak et al., 2015 | 179/66 | Siemens Sensation 64 (CT) | 301–342 mGy*cm2 | Iopromide | 18.6 | +267% | [27] |
Wang et al., 2017 | 48/22 | Siemens Sensation 64 (CT) | 294 mGy*cm2 | Iopromide | 33 | +38% | [28] |
Van Cauteren et al., 2021 | 45/5 | Coronary CT in humans | 11.3 mGy ± 5.3 mGy | Iopromide, iobitridol, iomeprol | 16.5–34.0 | - | [29] |
-: blank cell
This heightened DNA damage was consistently observed across various types of commercial scanners, encompassing studies involving both adults and children and notably, in scenarios involving clinically relevant iodine concentrations (with an average iodine administration of around 35 g). Importantly, this effect was found to exhibit a highly significant positive linear correlation between the administered blood iodine dose and the radiation-induced DNA damage. The excess amount of DNA damage observed in contrast-enhanced images compared to non-contrast images ranged notably from +30% to +200%. This substantial range underscores a pertinent amplification effect on radiation-induced damage, particularly noteworthy at clinically relevant iodine doses [27–29] (Figure 1).
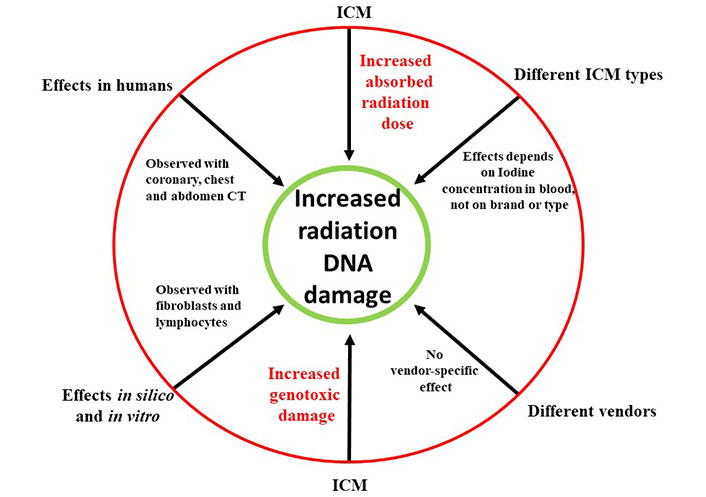
The increased radiation absorption and increased genotoxic damage to DNA mediated by ICM administration. The effect is independent of ICM brand and radiologic equipment, and observed in silico, in vitro, in experimental animals and in humans
Implications for clinical practice
The consideration of ICM as a factor increasing the radiation absorbed dose and associated DNA damage is especially important since the cumulative dose of a patient now averages 2.3 milliSievert (mSv) per capita in the United States, and the cumulative dose per cardiological patient can be more than 100 mSv per single hospital admission and even for a single procedure, such as dilation of chronic coronary occlusion or endovascular treatment of an abdominal aortic aneurysm [30]. This is especially worrying in cardiac patients since the ICM dose required is often > 50 g. A prudent physician should take into account the cumulative radiation exposure stemming from both prior and ongoing examinations to mitigate excessive cumulative cancer risks, especially in children, adolescents, and young adults [31]. It is imperative to uphold the principles of optimization and justification, ensuring not only the appropriateness of the examination but also executing it with the lowest feasible radiation dose, adhering to the As Low As Reasonably Achievable (ALARA) principle. Additionally, this careful approach should extend to the administration of ICM to mitigate not only acute and subacute but also long-term side effects, e.g., radiation-induced cancer, manifesting years or decades later.
Despite these apparent considerations, real-world clinical practice often presents challenges. Studies indicate that approximately 50% of examinations involving intravascular administration of ICM, such as computed tomography and invasive fluoroscopy in cardiology, are either wholly or partially inappropriate [32]. Moreover, there is a prevalent trend of inappropriate overutilization of ICM [30]. Balancing the need for accurate diagnoses and patient safety against the risks associated with radiation exposure and ICM administration poses a significant challenge in clinical practice. Informatic clinical decision support systems have been developed to limit the overuse of ICM to limit acute kidney disease and might be usefully applied in general to limit the adverse side effects of ICM in invasive cardiology and cardiac radiology [33].
Implications for research studies
In recent times, there has been a redefinition and elevation of the radiation risk profile. This shift is attributed to the maturation of epidemiological data derived from extensive exposure to professional, environmental, and medical radiation among millions of subjects. Notably, this estimated significant impact of radiation on cancer disregarded the load of ICM. The obligatory administration of ICM during invasive fluoroscopy studies can be an important co-determinant of the unexpected increase in cancer risk observed in observational studies in radiosensitive organs receiving the highest organ dose, for instance with an increase in abdominal cancer in patients undergoing endovascular abdominal aortic aneurysm repair [34]. Considering this, future trials should include the variable of ICM load within the dataset of prospective randomized studies comparing medical or surgical treatments to interventions reliant on fluoroscopy, known for their higher radiation exposure levels. Incorporating the assessment of ICM load alongside radiation exposure in these trials would enable a more comprehensive understanding of the associated risks, thereby facilitating more informed and balanced treatment decisions in medical practice.
Conclusions
The genotoxic impact of ICM remains largely overlooked by physicians, yet it constitutes a substantial contributor to the heightened cancer risk observed in patients subjected to medical radiation. The fundamental principles of justification and optimization typically applied to radiological procedures and further emphasized in Europe by the 2013/59 Euratom Directive [35], ought to extend to the utilization of ICM. Recognizing the significance of iodine in this context is crucial, as it amplifies and exacerbates the oncogenic effects resulting from radiation exposure. Therefore, it becomes imperative to integrate considerations regarding ICM usage into the overarching framework of radiation safety protocols, aligning with the established principles of justification and optimization to ensure enhanced patient care and minimize long-term health risks. The importance of ICM as an amplifier of radiation damage was first recognized by the early pioneering observations [23] of a pediatric cardiologist, Adams and Rigler [36], who already in 1964 wrote a visionary editorial with Riglerin Circulation on “Reduction of Radiation to Children”. Dr. Adams stated that “Properly controlled radiation can provide benefits that greatly outweigh the potential hazards if there are adequate indications for its use and the instrumentation and technics are optimal. (…) Ideally, radiation exposure records should be maintained for each patient, particularly those requiring long-term follow-up” [36]. Half a century later, cardiologists may repeat Adams’s words, adding that both radiation exposure and ICM records should be maintained for each patient.
Abbreviations
CT: | computed tomography |
ICM: | iodinated contrast media |
γ-H2AX: | phosphorylated histone H2AX |
Declarations
Author contributions
CI: Conceptualization, Writing—original draft. MX, VP, and EC: Writing—review & editing.
Conflicts of interest
The authors declare that there are no conflicts of interest.
Ethical approval
Not applicable.
Consent to participate
Not applicable.
Consent to publication
Not applicable.
Availability of data and materials
Not applicable.
Funding
Not applicable.
Copyright
© The Author(s) 2024.