Abstract
Adventitial crosslinking is a method in current investigational stage for preventing the rupture of aortic aneurysms. It is based on the photochemical crosslinking of adventitial collagen by exposure to ultraviolet A radiation. Essentially, an adventitial top layer is generated that displays enhanced mechanical properties and imparts additional strength and stiffness to the aneurysmal wall. Looking back upon the history of aortic surgery during 1940s, the aortic film wrapping, then dubbed “cellophane wrapping”, also was a procedure employed for delaying the aneurysmal rupture. In principle, the two procedures are similar in that both result in laminar composites, although the top layers differ fundamentally from each other. This review discussed in some detail the use and clinical outcomes of the aortic wrapping with artificial films, also mentioning the contemporary procedures still grouped under this umbrella term. The focus of the review was a comparative view on two procedures, the aortic film wrapping and adventitial crosslinking. It was concluded that the methods are different in many aspects, including the mechanisms of action. In fact, the promoters of adventitial crosslinking were not aware of the prior existence of aortic film wrapping. However, the achievements of the classical wrapping, by now regarded as merely historical episodes, did not discard prior knowledge, but repurposed it in a process that led to innovative strategies.
Keywords
Aortic aneurysms, wrapping procedures, film, cellophane, polyethylene, external reinforcing, adventitial crosslinkingIntroduction
In its extensive multitude of forms, arterial disease is a major cause of death in the world. It includes the aortic disease, which can materialize itself into a large variety of pathologies, from those with slow progression to those causing sudden vascular rupture [1]. If not treated effectually, such conditions can lead to high levels of morbidity and mortality in human population. Since their identification in antiquity, when also christened with names originating in the ancient Greek language [2], the terms “artery”, “aorta”, and “aneurysm” have been associated with countless attempts to find treatments for more or less recognizable vascular pathological conditions. Nevertheless, historic unfolding shows that it was more progress achieved in the course of the 20th century alone than in the preceding two millennia [3–11]. The present level of advancement of vascular surgery in general, and aortic surgery in particular, is the result of the efforts and dedication of brilliant minds and ingenuity, such as Halsted, Carrel, Jeger, Matas, Leriche, De Bakey, Blalok, Cooley, Diethrich, Dubost, Blakemore, and other equally prominent surgeons. Closer to our times, their work has been continued and expanded successfully by a plethora of remarkable surgeons and physician-scientists whose contribution is reflected, for instance, in the currently decreasing prevalence and mortality of abdominal aortic aneurysm (AAA), thanks to refined surgical strategies, rapid diagnosis, greater insight into the pathophysiology of disease, and effective medication.
The subject of this paper relates to a procedure that was developed in the 1940s and applied in order to avoid the rupture of aneurysms—the wrapping of aneurysmal arteries with a thin plastic sheet (film). Although apparently simple at the first glance, the mechanism of this technique is associated with certain aspects that this study will attempt to clarify. For instance, was the mechanical reinforcement of the dilated aortic region, ascribable to a tight wrapping, the main factor for a successfully delayed rupture? This issue has become recently of some import, as a procedure for delaying indefinitely the rupture has been proposed based on the mechanical strengthening of the aneurysmal abdominal aorta through the photocrosslinking of adventitial collagen induced by exposure to the region A of ultraviolet (UV) radiation at a wavelength of 365 nm [12–15]. The same technique proved effective on veins too, and therefore suggested also to be used for alleviating the venous compliance mismatch in arteriovenous grafts [16, 17]. Before evaluation in clinical trials for becoming a legitimate vascular surgical procedure, the method—that was named “adventitial crosslinking” [17]—is still the subject of ongoing laboratory investigations.
At this point, a question can arise as to whether the aortic wrapping may be used as a supporting argument for the validity of adventitial crosslinking (ACL). In principle, both procedures involved the additional insertion of a peripheral layer, either outside or inside the adventitia. Although the proponents of ACL were not aware of the “historical” aortic wrapping at the time of their proposal, it is now an opportunity to acknowledge that the idea to delay the aneurysm rupture by perivascular reinforcing or restraining, originated about 80 years ago. While the crosslinking therapy relies entirely on the enhancement of mechanical properties of an irradiated superficial layer of the adventitia, the functionality of aortic wrapping is rather tangled and worthy of learned debate.
An overview of wrapping techniques in vascular surgery
The cellophane was the first material of choice as a plastic wrap in surgery. This material is made from regenerated cellulose and became available in the 1910s as films of a variety of thickness and resistance to water vapor (moisture). It is biodegradable and non-toxic, and displays low permeability to liquid water, air, oils, and bacterial contamination. As the industrial production of plastic wraps made of synthetic polymers, such as poly(vinyl chloride), polyethylene, polypropylene, or poly(vinylidene chloride), took off only a few decades later, the term “cellophane” came to be used informally for many of such products that chemically had nothing to do with cellulose. This certainly makes difficult to identify what material was actually the “cellophane” used by some surgeons at that time.
An earlier surgical use of cellophane was in orthopedic surgery, as a permanent tendon sheath in arthroplasties [18], or as interposition membranes fitting the suprapatellar pouch in cases of synovectomy [19]. Commercially available films of cellophane “No. 300”, with a thickness of 22.3 μm, were used in both applications with a successful outcome. No foreign-body reaction was noted.
The potential of cellophane wrap to constrict blood vessels in order to generate experimental arterial hypertension was discovered by Page [20, 21] in animal models where he wrapped the kidneys with cellophane; after a few weeks, a fibrocollagenous “hull” formed that constricted the renal vasculature. The implanted cellophane material degraded and gradually disappeared, but the shell remained and thickened, likely due to persistent fibrotic reaction. Pearse [22] was the first to extend the method to the canine aorta and carotid, showing that by wrapping the vessels with cellophane “300 P.T.” (uncoated, therefore not moisture-proof), progressive occlusion of the lumen occurred, which was the target of the experiments as a potential anti-aneurysmatic strategy. The formation of an occluding mass was explained by a strong foreign-body reaction and fibrosis induced by the cellophane.
Harrison and Chandy [23] reported the first use in human patients of the cellophane wrapping technique. They treated two patients in Bahrein, who presented with aneurysms of the subclavian arteries, by wrapping the vessels with a few successive layers of cellophane both proximally and distally to the bulge, and securing with sutures. A successful outcome was recorded in one patient who was followed up to 20 months after surgery, when the aneurysmal bulge became fully obliterated. Unfortunately, the progress of the second patient had worsened due to a postoperative infection, and the cellophane wrapping had to be replaced by ligation. Clinical success with cellophane wrapping was also reported later [24] in a series of aortic aneurysms.
Poppe [25–28] undertook the most comprehensive experimental investigation of the perivascular wrapping for treating aortic aneurysms. First, noticing that the cellophane was previously described either as a completely inert, or as a reactive material causing fibrosis, he concluded that the chemical composition must be the determinant factor. Then, in an animal model (dog), the physiologic reactions elicited by five different plastic films available on the market at that time, including cellophanes grades P.T. and P.U.T. (both uncoated, but of different mechanical strength), cellulose acetate, poly(vinyl alcohol), and polyethylene, have been evaluated [25]. The films were wrapped around the abdominal aorta, and the fibrotic reaction was assessed postoperatively. A polyethylene film (38-μm thick) was the only material causing massive fibrosis, leading after 10 weeks to a six-fold increase of the wall thickness and a reduction of the luminal diameter by 70%. Poppe [25, 26] has subsequently applied polyethylene wrapping to treat ascending or descending aortic aneurysms in nine patients. Five patients survived for one to three years, and the remaining were still alive at the time of reporting. The success of a certain type of polyethylene to induce fibrosis was attributed to contamination with dicetyl phosphate [28, 29], a chemical additive used in production of the Du Pont’s product “Polythene NV-7-14” film. Poppe used the chemically meaningless misnomer “polythene cellophane” for this material, which unfortunately was adopted by other surgeons too. The application of this particular wrapping film has been reported with variable success in treating a range of arterial aneurysms [30–33]. Wrapping cloths such as Nylon, Dacron, and Marlex polyolefins have been also reported as being successful in reducing the bulge size [34, 35].
“Cellophane wrapping” for AAA will be indissolubly associated with a famous patient, Albert Einstein, an episode that was given much publicity both in medical literature and in tabloids. Perhaps the most influential scientist ever, and a friendly person with a great sense of humor, Einstein was unfortunate to suffer for half of his life from multiple ailments [36]. In December 1948, while living in the USA, he was suspected of an intestinal cyst and admitted to Brooklyn Jewish Hospital for surgical removal. Upon examination by the attending surgeon, Rudolph Nissen, a large AAA was detected. Nissen, himself a prominent surgeon known for pioneering achievements in gastroesophageal surgery [37, 38], decided to apply “cellophane” wrapping [36, 39, 40]. The anterior two-thirds of the aneurysm were wrapped with a film that very likely was polyethylene, not true cellophane. Einstein’s aneurysm ruptured on 13 April 1955 and he died five days later at the age of 76, in Princeton Hospital [36, 39–41], after declining resection surgery. His survival period of six years and four months following an aneurysmal rupture is probably the longest ever reported to occur after applying aortic wrapping. We might speculate that if the wrapping would have contained the posterior aorta too, this period would have been even longer. As the original autopsy report went missing, the only postmortem information was gathered almost six decades later via an interview with the same pathologist who conducted the autopsy [40]. The main findings included massive hemorrhage in the abdominal cavity, a lumen “riddled with plaques”, and no evidence of any residual wrapping material.
Although admitting that the wrapping technique led to symptomatic improvement, De Bakey and colleagues [42] suggested that the procedure does not have an “actual curative value”, and illustrated it by their detailed analysis of a series of eight cases of AAA treated by polyethylene film wrapping. In all cases, the patients had to be readmitted after periods ranging from seven to 41 months to have the aneurysms excised and replaced with homografts. However, we may argue that to define a “cured” aneurysm is not a straightforward point at issue. Also, the patients may pass away from unrelated causes after a period of time in which the potential rupture of their aneurysm was kept in check by the successful application of a certain procedure, whether the aortic wrapping or another technique. Therefore, we might rather agree that as long as the patients did not expire because a ruptured aneurysm, all procedures for delaying the rupture display formally the same curative value.
By the 1950s, the status and limitations of arterial aneurysm treatments became distinct. Three major procedural categories were identified [42, 43]: (a) fibrosis-induced occlusion of the vessel (“cellophane” wrapping belonging here); (b) opening of aneurysmal sac followed by its surgical remodeling in order to achieve a normal lumen size (endoaneurysmorrhaphy); and (c) extirpation of aneurysm and restoration of normal blood flow, with or without removal of the involved vessels.
The next stage was a transition to the modern era of arterial surgery, what we can legitimately label as its golden era. Perivascular wrapping with thin plastic sheets/films did not last to reach to and be part of this era, as its application dwindled, if not ceased altogether. However, the term “aortic wrapping” survived as an occasional name for the external grafting without the resection of aorta, which was developed to treat small or medium-sized fusiform aneurysms of the ascending, thoracic, or abdominal aortas, or of the aortic arch [44–49]. The technique usually involved Dacron or Teflon off-the-shelf vascular prostheses, commonly corrugated, that were cut open longitudinally, placed around the vessel, trimmed to the necessary size and shape, and sutured tight around the aneurysm. A warning [46] to avoid confusion with what used to be known as “cellophane wrapping” was apparently ignored; others described [47] the external grafting as a reintroduction of the former, and currently the terms are used imprecisely. When dealing with a dilated ascending aorta, the procedure is frequently termed “wrapping” [50–52], therefore, the confusion on nomenclature persists [53]. More recently, the external grafting/wrapping with Teflon was extended to the treatment of aortic dissection [54–56] and of intramural hematoma [57].
In this paper, the term “aortic film wrapping” (henceforth, AFW) will be used for the classical (“cellophane”) wrapping, and “aortic external reinforcing” (henceforth, AER) for the current procedures including external grafting or girdling/banding with prosthetic materials in the form of sheets, plates, or mesh. Beyond the terminology debate, it is important to know whether mechanical reinforcement is responsible for the performance of these two procedures. Theoretical approaches have been used to assess the distribution of wall stress when the ascending aorta is externally reinforced [58]: computational stress analysis by the finite elements methodology showed that stress was only slightly higher in the reinforced vessel when compared with that in the non-dilated aorta model, but lower than in a moderately-dilated model. As a collateral research interest, the effect of AER on improving the aortic compliance in order to avoid reduction of vascular distensibility and age-related stiffening, has been investigated on a model of the aged human aorta using non-linear transient numerical analysis [59]. The results suggested that the elastic reinforcing reduced stiffening, but displayed a potential risk of over-compression leading to internal folding of the wall. This confirmed in part the results of a previous study [60] where AER using an elastic silicone sheet decreased stiffness of aged ascending aorta in vitro, and of dilated vessels in vivo in a sheep model. Actually, such an outcome may not be meaningful for preventing aneurysmal rupture where the aim is the enhancement of mechanical properties.
If we add now the ACL (as detailed in the Introduction, and henceforth ACL) to AFW and AER, we end up with three procedures aiming at reinforcing a dilated aorta, presumably based on the concept that by adding external layers on the top of adventitia, the tensile properties (stiffness and strength) of the whole wall shall be enhanced. However, attractive by its simplicity may be, this assumption is untenable without theoretical support. So far, modeling approaches could not predict the failure of external layers or provide information on the strength of mechanical reinforcement. In fact, the concept of strengthening and stiffening an aneurysmatic artery may not be a method as efficacious as expected for preventing its rupture. Enlargement of an aneurysm and its final rupture take place in the same hemodynamic environment and are the result of an interplay between material factors (e.g., regional decline of mechanical properties, structural fatigue, microstructural characteristics of the tissue) and the pathophysiological growth and repair processes. Obviously, the idea that a rupture occurs when stress exceeds the wall strength and/or rigidity should be regarded rather as an oversimplification. Therefore, reinforcing of an aneurysm by adding peripheral layers has its limitations due to the complexity of the rupture process as such.
Structural comparison of the reinforcing procedures
There are essential differences between AFW, AER and ACL reinforcing methods. By covering the aorta with an external layer, or by modifying the adventitial top layer, a toroidal sandwich is generated (Figure 1A). In AER (Figure 1B), the covering layer is significantly thicker and less elastic than those in AFW and ACL, and is often secured by external means (mainly sutures). This layer also must be able to recapitulate the properties of the target native blood vessel, a condition not necessary for AFW but self-evident in ACL. AER can generate free pockets between the external layer and native adventitia, and induce extensive wall degeneration and atrophy [61]. In AFW and ACL, the thickness of covering layers is in the micron range, and they are more intimately bonded to the adventitia, in AFW due to water-assisted interfacial adhesion, capillarity-induced cohesion, and microstructural effects [62–66], and in ACL by in-situ photochemical modification of the adventitia [12, 15]. The adhesive strength between the artificial film and outer adventitia in AFW is determined by the cohesive energy density and interfacial adhesion energy, and may require molecular modelling and dynamic simulations for evaluation.
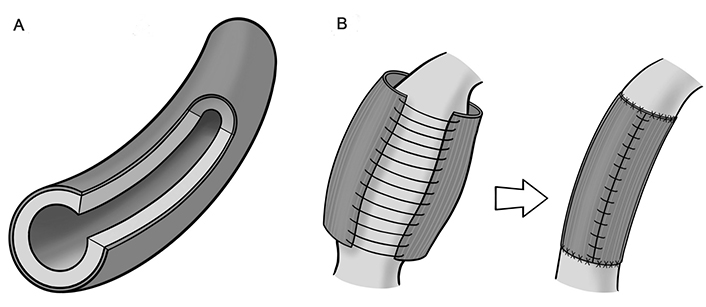
Concepts of the procedures. A. Peripheral additional layering; B. aortic external reinforcement
A common denominator for the three procedures, potentially offering a supporting theoretical treatment, would be to consider each of the resulting structures as a laminate consisting of two layers (laminae). However, the classical theory of lamination cannot be applied for modeling these particular bilayer laminates because they do not fulfil most of the fundamental assumptions of the theory [67], which include: a perfect bonding between laminae (with no slippage), which must not be deformable by shear stress; the laminate shall act as a single lamina; each lamina must be a homogeneous material layer; and the laminate must deform according to Kirchoff’s hypotheses regarding the normals (i.e., the lines intersecting the surface at right angle) to the midplane. A theoretical approach becomes even less achievable if considering the stresses and strains arising along three axes during pressurization (driving, transmural, and hydrostatic pressures) and filling (pulsatile deformation) of the vessel.
Multi-layered constructs in biomedicine are rather scarcely available for a comparison with the vascular wall/external sheet bilayer resulting from the above reinforcing procedures. Constructs have been developed from synthetic polymers for applications in tissue engineering and regenerative medicine, aiming at duplicating the structure and mechanical properties of tissue to be replaced or augmented, and at functioning as substrates for growing cells, or as matrix for drug release. Techniques such as layer-by-layer self-assembly, sequential chemical processes, electrospinning, and 3-D printing have been employed [68] to obtain a variety of biomaterial templates, but none intended as a perivascular coat. Multi-layered engineered blood vessels and grafts are routinely produced from biomaterial scaffolds seeded with vascular cells, where the cell sheet stacking is a preferred fabrication technique [69, 70]. However, the resulting products were developed as wholly implantable components for the surgical repair of diseased vessels, and does not include materials for reinforcing dilated aortas. In another marginally related example, mechanical strengthening of bioprosthetic heart valves based on decellularized bovine pericardium was reported by coating with synthetic polymers [71, 72].
There may be only one reported polymer substrate/coating layer composite that has been evaluated to confirm experimentally that the mechanical properties were enhanced globally by adding a layer [73]. A slab of elastic silicone polycarbonate-polyurethane copolymer was dip-coated with chitosan, resulting in a laminate where the upper lamina had a thickness around 10 μm. The uniaxial tensile testing indicated an increase in the stiffness of laminated composite after coating (Young’s modulus 5.8 ± 1.0 MPa compared with 2.9 ± 1.3 MPa for the uncoated substrate), while the tensile strength and elongation at break remained unchanged. This finding casts back to an earlier statistical treatment of strength in laminated composites [74], where was suggested that when n sheets are attached together to form a laminate, the load-carrying capacity per sheet is much greater than that of an individual sheet, which was regarded as a result of interlaminar adhesion. However, this conclusion may not be valid for a value as low as n = 2.
An example of biological reinforcement, which may serve as a model for ACL, consists of the skin/subcutaneous white adipose tissue (sWAT) composite [75, 76], where the top skin layer (dermis) is thinner and stiffer than the adipose layer. The unavoidable age-induced decline in our skin’s mechanical properties led to procedures aimed at reinforcing each of the two layers. These procedures are based on the structural and quantitative enhancement of collagen network in both the dermis and the sWAT [76], which can be achieved through (a) reorientation of collagen fibers under compressive loading; (b) creation of new physical linkages within the fibrous system; and (c) additional crosslinking of collagen macromolecules leading to increased collagen density. The procedures do not result in the formation of additional layers to the skin/sWAT laminate, but may lead to slightly increased thickness. By allowing a number of simplifying assumptions regarding the properties of the two heterogeneous layers, a theoretical analysis has shown [76] that the efficacy of reinforcement is dependent on the applied loading, and the laminate is stiffer under transverse loading than under longitudinal loading.
Correlative analysis of AFW and ACL
Although based on wrapping a laminar material around the aorta, AER is compositionally and practically different from the other two procedures, AFW and ACL, which will be further analyzed. Their structural principles are depicted schematically in Figure 2A (AFW) and Figure 2B (ACL). An issue for our comparison would be the thickness of perivascular layers specific to each method. In AFW, that layer is a separate artificial film, while in ACL is an undivided layer of the adventitial tissue. Perhaps the latter should not be called “perivascular”, as it belongs inherently to adventitia.
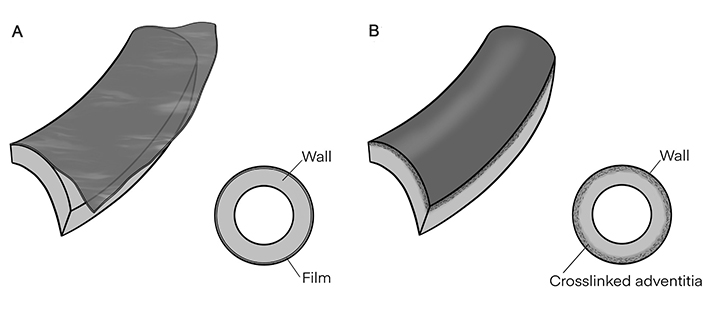
Structural principles. A. Aortic film wrapping (AFW); B. adventitial crosslinking (ACL). The layers’ thicknesses are not at real scale
Thickness of adventitia and whole wall in normal and aneurysmal aorta
To describe the composite structure of the crosslinked aorta in order to compare it with the aortic wrapping bilayer, it would be helpful to know the thickness of the aortic wall and of the adventitia. This is a notoriously difficult task, and we have to accept that there are reasons that “make estimates of wall thickness from ordinary histologic sections almost useless” [77], such as the imprecision in defining the limits of the adventitia related to perivascular tissue, and histological processing artifacts. However, based on a large number of published histologic micrographs of the sectioned aortic wall, it was possible to estimate the contribution of each layer by measuring it directly on the microphotographs, showing that adventitia accounts for 15–25%, media for 70–80%, and intima for 2–10% of the total wall thickness. With these data, if we consider a probable average value of 1.5 mm for the wall thickness in the large normal elastic arteries, we reach to the following values for the thickness of each layer: adventitia 225–375 μm, media 1,050–1,200 μm, and intima 30–150 μm. These values, as well as the value of 2.1–2.6 mm for the thickness of aneurysmal aortic wall, are adequately supported by a selection of published data [78–87].
Thickness of the film in AFW
There are hardly any information on the thickness of the cellophane or polyethylene films employed for aortic wrapping in the early days of the procedure. In two studies [19, 25] values less than 50 μm have been mentioned for the films, and we shall consider it as a reasonable value. Information on film thickness is also absent in later publications. Currently, polyethylene films alone are available on the market within a very large range of thickness (10 μm to 1 mm).
Thickness of the crosslinked adventitial layer in ACL
This thickness depends on the penetration distance of UV-A radiation into the tissue. The extent of crosslinking process depends on the advance of the diffusing front of the photosensitizer solution. Here, we shall elaborate on existing similar situations involving the penetration of electromagnetic radiation into tissues. By far and as expected, the skin has been most studied when compared to other tissues, while also has provided the largest scatter of the reported data, likely caused by a multitude of interfering factors such as skin type, variable regional skin structure and thickness, light sources, radiation wavelength, and irradiation conditions. Regarding the UV-A radiation only, it is agreed that it penetrates up to the dermo-epidermal junction, at a depth of ~100 μm, when ~50% of the incident fluence (i.e., energy density of radiation) is still preserved [88–90]. The residual fluence of UV-A radiation can be as high as 90% at low penetration depths between 10 μm and 20 μm, but drops to 10% at 100–400 μm and, respectively, to 1% at 700–1,000 μm depths [90].
Another issue of interest in comparing composites with a crosslinked upper lamina comes from the field of an established therapy for progressive keratoconus, an eye pathology that leads to severe visual disability. The method is based on the photochemical crosslinking of the corneal collagen using UV-A radiation and a photosensitizer (riboflavin) [91–94]. As a result of irradiating the anterior region of the cornea, the progress of keratoconus is arrested due to stiffening and strengthening of the collagen network. The development of adventitial photocrosslinking [12] has been actually inspired by the corneal photocrosslinking. UV-A radiation can penetrate through the entire cornea of the eye and beyond [95], however, the crosslinking is induced and limited only within the diffusion path of the photosensitizer solution, which, according to current protocols, is ~300 μm when the epithelium is removed, or ~100 μm for transepithelial irradiation [96, 97].
The penetration of UV-A radiation into arterial wall has been barely investigated, and the reported values have resulted by computations based on the attenuation coefficient α, which was estimated (in mm–1 or cm–1) from the transmission, absorption, reflection and scattering experimental data employing the Kubelka-Munk model. In in vitro experiments with the radiation source located inside the lumen of the aorta, a penetration depth of 200 μm was estimated for the UV radiation with 365-nm wavelength, and between 100 μm and 250 μm globally for the UV-A region [98]. Neither the presence of atherosclerotic plaques, nor the removal of adventitia has affected the results, the latter suggesting that the radiation did not reach the adventitia. A penetration of 182 μm of the 355-nm UV radiation into the wall of human artery in vitro was also reported [99], again based on the coefficient α, with no experimental details given. Accordingly, we may safely suppose that UV-A radiation can penetrate into the aortic wall up to ~150 μm, which thus can be taken as the thickness of the top lamina in the ACL system, assuming that the photosensitizer solution penetrated to the same depth.
Action mechanism of AFW
Poppe [25, 28] was the first user of AFW to discuss occlusion as its mechanism of action. He identified three different methods in use at that time to generate occlusion for treating large arterial aneurysms: (a) insertion of foreign materials into the lumen to induce thrombosis; (b) external compression (clamps, spring devices, and rubber bands); and (c) injury to the wall to induce fibrosis and reduction of lumen size, where AFW was included. The first two procedures have been generally ineffectual, and the third worked only when the films were contaminated with irritating substances. Poppe [28] concluded that neither the tensile strength of the wrapping film nor its tightness at enclosing have any effect on the level of aneurysm’s reinforcement: the film represents “merely a vehicle … for an irritating chemical which produces the fibrosis”. Strictly speaking, a mechanical action occurs eventually due to the fibrotic mass pushing and occupying part of the luminal space. To speculate whether the wrapping film contributed mechanically to occlusion through own strength and tightness is rather futile, as the film was rapidly degraded and disseminated after implantation, and any contribution during the initial postoperative stage would have been of little relevance.
Action mechanism of ACL
As mentioned in the Introduction, a new procedure is being developed for prevention of aneurysmal rupture, based on the crosslinking of adventitial collagen that leads to mechanical augmentation of the wall [12–17]. Crosslinking of collagen is a well-known reliable method. Its chemistry is mostly elucidated [100–104], and the exogenous crosslinking is extensively employed to generate substrates for regenerative medicine applications [105–107]. The function of the ACL is based on the enhancement of mechanical properties (tensile strength, stiffness) enabling the aneurysmal wall to resist and potentially delay indefinitely sudden rupture.
Analogy between AFW and ACL
There are fundamental differences between the two techniques. The wrapping films are external artificial sheets that adhere temporarily to the wall and break down relatively soon after implantation. The reinforcing layer in ACL is an intrinsic part of the adventitia, can last indefinitely, and cannot be removed from the system. While the action of AFW is based on fibrosis-induced luminal occlusion, with no mechanical contribution from the wrapping film, the ACL is based entirely on mechanical strengthening of the crosslinked adventitial top layer. Although based on insufficient literature data, the wrapping films were about 2–3 times thinner than the adventitial reinforcing layer resulted from irradiation.
Conclusions
Even if based on bilayer structures, and aiming at the same clinical outcome, the AFW and the ACL are fundamentally different in their nature, composition, and mechanisms of action. It is apparent that the classical aortic wrapping method could not have served as an inspiration for the ACL. Both procedures are associated with limitations arising from the mechanobiological complexity of an aneurysmal rupture event.
Abbreviations
AAA: | abdominal aortic aneurysm |
ACL: | adventitial crosslinking |
AER: | aortic external reinforcing |
AFW: | aortic film wrapping |
sWAT: | subcutaneous white adipose tissue |
UV: | ultraviolet |
Declarations
Acknowledgments
The author thanks to his institution for allowing him to carry out literature search and to write parts of the manuscript during working hours. He is also grateful to Mr. Ralee Winks and Dr. Shuko Suzuki for assistance with the graphic material.
Author contributions
TVC: Conceptualization, Investigation, Writing—original draft, Writing—review & editing.
Conflicts of interest
The author declares that there is no conflicts of interest.
Ethical approval
Not applicable.
Consent to participate
Not applicable.
Consent to publication
Not applicable.
Availability of data and materials
Not applicable.
Funding
Not applicable.
Copyright
© The Author(s) 2024.