Abstract
Vascular aging is recognized as one of the hallmarks of atherosclerosis. Currently, a growing body of evidence suggests that there exists a mutual crosstalk between telomere dysfunction and mitochondrial dysmetabolism during the process of vascular senescence. This underscores the importance of comprehensively studying the molecular mediators involved in this complex and intricate connection. In pursuit of this goal, the “VICTORIA” protocol entails a prospective single-center cohort study aimed at recruiting patients undergoing coronary angiography at Niguarda Hospital in Italy. The primary objective is to explore potential associations between peripheral markers of cell aging (telomere length and mtDNA content), dysregulation of non-coding RNA [specifically lncRNA TERRA and mitochondrial microRNA (MitomiR)], and the varied presentations of ischemic heart disease (stable angina, unstable angina, NSTEMI, and STEMI). Furthermore, we aim to investigate whether these markers correlate with vulnerable plaque characteristics, as assessed by optical coherence tomography findings. Additionally, systemic levels of pro-inflammatory biomarkers and novel indicators of senescence will be assessed. Patients will be followed up at 1 year to monitor primary outcomes including mortality, myocardial infarction, stroke, unplanned revascularization, and rehospitalization. The anticipated findings of this study hold promise for advancing our understanding of the telomere-mitochondria crosstalk, potentially paving the way for novel treatment modalities and refined risk stratification approaches for acute coronary syndrome.
Keywords
Stable angina, unstable angina, NSTEMI, STEMI, telomere length, mtDNA content, lncRNA TERRA, MitomiRIntroduction
In recent decades, DNA damage (telomeric, non-telomeric, and mitochondrial) has emerged as a crucial trigger of vascular aging and atherogenesis [1–7]. Indeed, extensive evidence revealed the presence of oxidative DNA lesions, telomere erosion, and mitochondrial DNA (mtDNA) damage in both experimental and human plaques [8–13] as well as in peripheral cells of atherosclerotic patients [14–17].
Additionally, there is a growing understanding that genomic instability can directly influence vascular cellular function by activating signaling pathways that induce a range of pathophysiological cellular and molecular alterations. These alterations facilitate inflammation, apoptosis, autophagy, and ultimately, cellular senescence, accompanied by the associated “senescence-associated secretory phenotype” (SASP) [7, 18].
Targeting DNA damage and the related molecular mechanisms of vascular aging may provide a logical rationale to developing comprehensive interventions aimed at mitigating age-related vascular dysfunction and disease [19].
Nonetheless, the precise mechanisms linking DNA damage to vascular and cellular aging, as well as to the pathogenesis of atherosclerosis and vulnerable atheroma, are still awaiting elucidation.
Currently, a growing body of evidence suggests that there exists a mutual crosstalk between telomere dysfunction and mitochondrial dysmetabolism during the process of vascular senescence. This underscores the importance of comprehensively studying the molecular mediators involved in this complex and intricate connection [20–22].
Changes in cellular aging, as measured by leukocyte telomere length (LTL) and mtDNA copy number (mtDNAcn), have recently gained recognition as independent biomarkers for predicting risk and prognosis in patients with coronary artery disease [23]. However, their potential as markers for plaque rupture and thrombosis leading to acute myocardial infarction has not yet been fully elucidated in detail. Moreover, recent findings suggest that a subclass of long noncoding RNAs (lncRNA) transcribed at telomeres, referred to as Telomeric Repeat-containing RNA (TERRA), plays an active role in the mechanisms governing telomere maintenance and chromosome end protection [24, 25]. However, the role and the expression levels of lncRNA TERRA in patients with cardiovascular disease have yet to be fully elucidated [26]. Furthermore, current research has revealed that microRNAs (miRNAs) within mitochondria, referred to as MitomiRs, may serve as significant mediators in the intricate crosstalk between the nucleus and mitochondria [27].
Some mature miRNAs are nuclear-encoded and are translocated into the mitochondrion, where they likely target nuclear-encoded mRNAs localized on the mitochondrial surface [28]. Others, known as MitomiRs, may originate from mRNA molecules derived from the mitochondrial genome, exerting post-transcriptional modifications within the mitochondria [28]. Dysregulation of MitomiRs may disrupt mitochondrial homeostasis and function during cellular senescence [29]. Therefore, MitomiRs could serve as crucial mediators in the regulation of mtDNAcn and may represent suitable candidates for therapeutic intervention. However, there are currently no studies that have investigated the role of MitomiRs in regulating mtDNAcn and, consequently, mitochondrial function in patient populations. Additional studies are necessary to elucidate the underlying mechanisms through which mitochondrial dysfunction influences telomere length (TL) and vice versa, as well as how their interaction contributes to the vascular aging process and clinical outcomes [23].
The “VICTORIA” protocol aims to explore potential associations between peripheral markers of cellular aging (TL and mtDNA content), dysregulation of non-coding RNAs (lncRNA TERRA, MitomiR), and the various presentations of ischemic heart disease [stable angina, unstable angina (UA), myocardial infarction without ST-segment elevation on ECG (NSTEMI), and myocardial infarction with ST-segment elevation on ECG (STEMI)], and to determine if they correlate with vulnerable plaque characteristics.
Materials
Study design and population
The VICTORIA study (ClinicalTrials.gov, Identifier: NCT06313645) is a prospective single-center cohort study involving patients suffering from chest pain undergoing coronary angiography and revascularization procedures at the Cardiology Department of Niguarda Hospital, Italy. The study adheres to the general principles outlined in the Declaration of Helsinki [30] and the rules of good clinical practice (GCP).
A total of 300 consecutive patients with acute coronary syndrome (ACS) will be enrolled, including those presenting with myocardial ischaemia at rest or on minimal exertion in the absence of acute cardiomyocyte injury/necrosis (UA), NSTEMI, and patients with acute STEMI, as defined by the European Society of Cardiology [31].
The study will also recruit patients with documented chronic coronary artery disease admitted for coronary angiography or elective revascularization (stable angina) and patients with positive stress test but with non-angiographically significant coronary artery disease. Exclusion criteria will include patients in cardiogenic shock, with congestive heart failure, valve disease, acute renal failure, undergoing coronary artery bypass grafting, or with active cancer.
Clinical characteristics and signs for ACS diagnosis are described in Table 1.
Clinical symptoms and vital signs for the diagnosis of patients with acute coronary syndrome (ACS)
Resting chest pain | ECG | Troponin level at 0 h | Troponin change within 1–3 h | Diagnosis |
---|---|---|---|---|
+ | Normal | –/+ | –/+ | UA |
++ | No ST-elevation | + | ++ | NSTEMI |
+++ | ST-elevation | ++ | +++ | STEMI |
UA: unstable angina; NSTEMI: myocardial infarction without ST-segment elevation; STEMI acute myocardial infarction with ST-segment elevation on ECG
Patients will undergo follow-up within 12 months, either through hospital records or via in-person or telephone interviews, to evaluate occurrences of all-cause mortality and major adverse cardiovascular events (MACE).
Procedure
Data collection and biobanking
Prior to enrollment, all eligible participants will need to provide informed written consent. The study will document all clinical characteristics for each patient, comprising demographics, complete medical history, medication details, and previous diagnostic and therapeutic interventions. Upon admission, patient laboratory data, encompassing total blood count and standard biochemistry parameters, will be collected. Experienced interventional cardiologists will gather angiographic parameters, including lesion characteristics and coronary dominance.
Additionally, specific details concerning plaque characteristics such as thickness, type of fibrotic cap, presence of intraplaque hemorrhage, and inflammation, will be obtained from patients undergoing evaluation of plaque morphology and composition using intravascular ultrasound (IVUS) or optical coherence tomography (OCT).
Within 24 h of admission, an echocardiographic assessment will be performed. This assessment will involve quantification of cardiac chamber sizing, measurements of the left ventricle (LV), and evaluation of conventional LV diastolic parameters using Doppler measurements, all in accordance with current published criteria [32, 33].
Before the angiography procedure, roughly 10 mL of peripheral blood will be drawn from each consenting participant in the catheterization laboratory for the collection, preparation, and storage of different biosamples. Aliquots of samples will be promptly frozen following standardized procedures. The study design is illustrated in Figure 1.
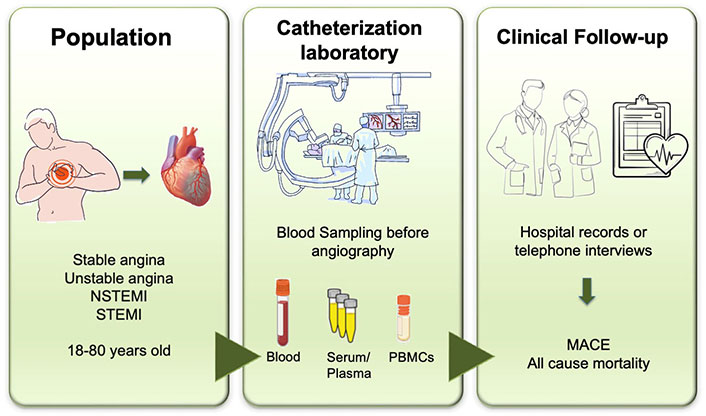
Flowchart of patient enrollment and procedures in the VICTORIA Study. MACE: major adverse cardiovascular events; PBMCs: peripheral blood mononuclear cells. Created in BioRender.com
Experimental workflow and biological measurements
The biomaterials utilized for molecular analysis will comprise serum, plasma (EDTA, citrate), genomic DNA (gDNA), RNA from whole blood, and peripheral blood mononuclear cells (PBMCs). Specifically, we will assess biomarkers of cellular aging (TL and mtDNA content) and the dysregulation of specific non-coding RNAs (lncRNA TERRA, MitomiR). Moreover, the project will investigate the potential role of circulating extracellular vesicles (EVs) in modulating the telomere-mitochondria link through miRNA-sensitive mechanisms. To gain insight into cellular effects and communication, we will also evaluate levels of secreted pro-inflammatory cytokines and EV cargo in the supernatant of the whole blood culture. Figure 2 provides an overview of the experimental workflow and planned measurements. After the collection process, the biological samples will undergo analysis using state-of-the-art methods to determine the levels of selected biomarkers.
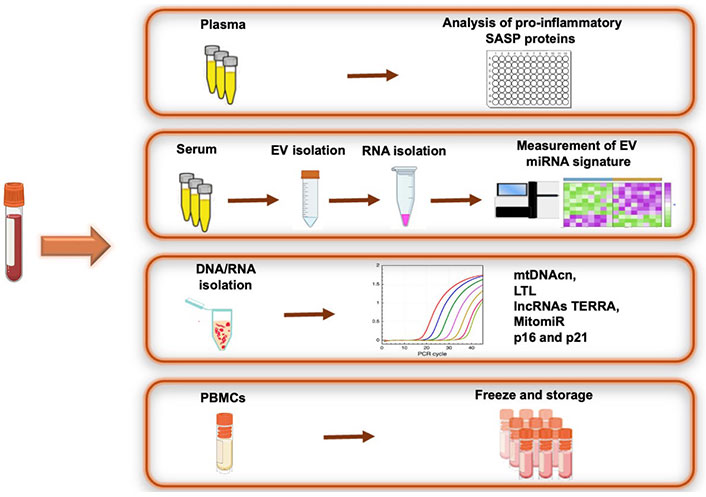
Overview of biomaterial processing and planned measurement of biomarkers. PBMCs: peripheral blood mononuclear cells; SASP: senescence-associated secretory phenotype; mtDNAcn: mitochondrial DNA copy number; LTL: leukocyte telomere length. Created in BioRender.com
Telomere length (TL) and mtDNA copy number (mtDNAcn)
TL and mtDNAcn will be assessed using DNA extracted from 200 µL of blood samples via quantitative real-time PCR, following standardized protocols. Briefly, TL will be determined by measuring the ratio of telomere repeat copy number (T) to a single-copy gene (S) and calculating the T/S ratio. The relative TL will be calculated using the formula “T/S ratio = 2^(–ΔΔCt)”, where ΔCt = Ct telomere – Ct single-copy gene. The T/S ratio provides an average TL across all leukocytes. For the quantification of mtDNAcn, the NDI1 gene in the undeleted region of the mtDNA reference sequence (mtNDI1) will serve as an internal control, while the human ß-globin gene from gDNA will be amplified by PCR in both gDNA and mtDNA. mtDNA-CN will be determined using the (2^ΔCt) method, where ΔCt = Ct mtNDI1 – Ct gDNA.
Analysis of MitomiRs and TERRA lncRNAs
A panel of MitomiRs known to be linked to senescence (miR-21-5p, miR-146a-5p, miR-181a-5p, miR-210, and miR-34a) and the expression levels of TERRA lncRNAs will be measured following RNA extraction from blood. Initially, total RNA (1 µg) will be reverse transcribed into cDNA using the TaqMan miRNA Reverse Transcription Kit (Thermo Fisher Scientific, USA). Subsequently, RT-qPCR will be performed utilizing sequence-specific TaqMan MicroRNA Assays (Thermo Fisher Scientific, USA) and TaqMan Universal PCR Master Mix (without AmpErase® UNG). Specific oligonucleotides will be used to amplify TERRA transcripts from different chromosome ends. Relative transcript levels will be calculated using the ΔΔCT method and normalized to the reference genes.
Exosome isolation and profiling of exosome-derived miRNA
Exosomes will be isolated from serum using an Exosome Isolation Kit (Invitrogen, Life Technologies) according to the manufacturer’s instructions. The quality, concentration, and size of exosomes will be assessed by nanoparticle tracking analysis (NTA). RNA extraction from exosomes will be performed using a specific miRNA Kit (Qiagen, Hilden, Germany). Library preparation, quality control, and next-generation sequencing procedures will be outsourced to a specialized company. Finally, the expression of the selected dysregulated miRNAs will be confirmed by qRT-PCR analysis.
SASP mediators
Pro-inflammatory cytokines and chemokines [interleukins (IL)-2, IL-6, IL-8, IL-18, tumor necrosis factor α (TNFα), monocyte chemoattractant protein-1 (MCP-1)] will be measured in patient plasma or serum using commercially available Human ELISA Kits (FineTest, Wuhan, Hubei, China). Briefly, the microplates will be coated with monoclonal antibodies specific to cytokines. Standards and supernatants will be added to the wells of the microplate and run in duplicate. Cytokine concentrations (pg/mL) will be determined against a standard concentration curve. Additionally, the expression levels of cell cycle inhibitor expression (p16 and p21) will be quantified by RT-qPCR analysis in isolated PBMCs (p16 and p21) will be also performed by RT-qPCR analysis in isolated PBMCs.
Sample size and statistical analysis
To detect a medium effect size (f = 0.25) in the difference of the mean LTL value between the groups, we estimate that a sample size of at least 232 total patients is required, with an alpha level of 0.05 and a power of 90% or higher. Accounting for a at least 10% dropout rate, the total number of patients to be enrolled should be approximately 300.
In terms of statistical tests, normal distribution of data will be assessed using the Kolmogorov-Smirnov test. Continuous variables will be presented as mean, standard deviation, median, first, and third quartiles. Categorical variables will be expressed as numbers and percentages.
Comparisons between two groups will be conducted using Student’s t-test for independent samples for continuous variables, and the chi-square test or Fisher’s exact test for categorical variables. Comparisons between more than two groups will be tested with one-way analysis of variance (ANOVA) followed by Bonferroni post-hoc tests for pairwise comparisons. If the assumption of normal data distribution is not met, equivalent non-parametric tests will be employed.
Pearson’s or Spearman’s coefficient will be calculated to investigate univariate correlations between variables. Event-free survival curves will be constructed using the Kaplan-Meier model and tested with the log-rank test between different groups. The Cox proportional hazards model, both univariate and multivariate, will be used to identify the predictive value of each variable vs. MACE events; data will be expressed with hazard ratios and their 95% confidence intervals.
Data management and statistical analyses will be conducted using SPSS software, version 26 (IBM SPSS Statistics) and StatView statistical package, version 5.0.1 (Abacus Concepts, Berkeley, CA, USA).
Expected results
Vascular aging is recognized as one of the hallmarks of the atherosclerotic disease and its acute thrombotic events. While chronological aging is an inevitable risk factor for atherosclerotic vascular disease, age-related vascular damage can also be influenced by lifestyle, environment, and extrinsic stimuli, leading to a gradual decline in structure and function within the vascular system. The identification of novel risk markers holds promise for enhancing risk stratification and facilitating the development of innovative treatment strategies.
Telomere and mitochondrial dysfunctions are recognized as crucial triggers in the pathogenesis of vascular aging and atherosclerosis, making them potential targets for the treatment of age-related vascular diseases.
In this context, the prospective, non-interventional cohort study involving patients hospitalized with ACS has the potential to significantly advance our understanding of how telomere function and mitochondrial metabolism influence the clinical presentation of ischemic heart disease (including stable angina, UA, NSTEMI, and STEMI) and plaque phenotypes. Progress in this field has the capacity to uncover therapeutic targets and define novel, more accurate diagnostic, and prognostic indicators.
Abbreviations
ACS: | acute coronary syndrome |
ANOVA: | one-way analysis of variance |
EVs: | extracellular vesicles |
GCP: | good clinical practice |
gDNA: | genomic DNA |
IL: | interleukins |
IVUS: | intravascular ultrasound |
lncRNA: | long noncoding RNAs |
LTL: | leukocyte telomere length |
LV: | left ventricle |
MACE: | major adverse cardiovascular events |
MCP-1: | monocyte chemoattractant protein-1 |
miRNA: | microRNA |
MitomiR: | mitochondrial microRNA |
mtDNA: | mitochondrial DNA |
mtDNAcn: | mitochondrial DNA copy number |
NSTEMI: | myocardial infarction without ST-segment elevation on ECG |
OCT: | optical coherence tomography |
PBMCs: | peripheral blood mononuclear cells |
SASP: | senescence-associated secretory phenotype |
STEMI: | myocardial infarction with ST-segment elevation on ECG |
TERRA: | Telomeric Repeat-containing RNA |
TL: | telomere length |
TNFα: | tumor necrosis factor α |
UA: | unstable angina |
Declarations
Acknowledgments
We are very grateful to the nursing staff of Hemodynamic Laboratory, including Giusi Longo, Anna Iacomella, Simone Manca, Orazio Leonardi. Figures 1 and 2 were created with BioRender.com.
Author contributions
JC and PC: Conceptualization, Investigation, Writing—original draft. MGA: Conceptualization, Investigation, Writing—original draft, Funding acquisition. MP, CD, GG, RN, and AB: Investigation, Writing—review & editing. EP, IB, GE, and JO: Writing—review & editing, Supervision. All authors read and approved the submitted version.
Conflicts of interest
Andrea Borghini who is the guest editor of the special issue “Molecular Mechanisms of Cardiovascular Aging” of Exploration of Cardiology had no involvement in the decision-making or the review process of this manuscript. The other authors declare that they have no conflicts of interest.
Ethical approval
The VICTORIA study was approved by Ethics Committee of Niguarda Hospital (reference number: 211-18042023/18 April 2023). All methods are performed in accordance with the ethical standards as laid down Declaration of Helsinki and its later amendments.
Consent to participate
The eligible participants provide informed written consent.
Consent to publication
Not applicable.
Availability of data and materials
The data generated during this study will be not publicly available because restricted by the Local Ethics Committee.
Funding
We acknowledge co-funding from Next Generation EU, in the context of the National Recovery and Resilience Plan, Investment PE8—Project Age-It: “Ageing Well in an Ageing Society” [(Partenariato Esteso - PE0000015) - CUP B83C22004880006]. The views and opinions expressed are only those of the authors and do not necessarily reflect those of the European Union or the European Commission. The funders had no role in study design, data collection and analysis, decision to publish, or preparation of the manuscript.
Copyright
© The Author(s) 2024.