Abstract
Redo surgical aortic valve replacement (SAVR) has long been the standard treatment for severe symptomatic aortic stenosis (AS) and insufficiency, but transcatheter aortic valve replacement (TAVR) has emerged as a less invasive alternative, particularly for symptomatic AS. With calcification being a primary mechanism behind structural valve degeneration, factors such as patient characteristics and the type of bioprosthetic valve play crucial roles in determining risk. Valve-in-valve TAVR (ViV TAVR) has gained prominence as a viable alternative to redo SAVR in recent years. Echocardiography plays a pivotal role in patient selection, procedural guidance, and post-procedural evaluation in ViV TAVR. From pre-procedural assessment using transthoracic and transesophageal echocardiography to intra-procedural guidance and post-procedural follow-up, echocardiography aids in ensuring procedural success and monitoring valve function. Decision-making between redo SAVR and ViV TAVR involves multidisciplinary teams considering various factors like patient risk profile, anatomical considerations, and technical feasibility. While redo SAVR remains preferred for younger patients with lower risk, ViV TAVR is increasingly considered for older patients or those with higher reoperation risks. Factors such as coronary artery obstruction risk, patient-prosthesis mismatch, and paravalvular leak guide treatment decisions. Procedural techniques in ViV TAVR have evolved to minimize complications and optimize outcomes. Factors like access route selection, valve choice, and procedural guidance techniques significantly impact procedural success. Balloon valve fracture and preventive strategies against coronary obstruction are crucial considerations during the procedure. Post-procedural evaluation involves assessing clinical and hemodynamic outcomes, with long-term studies indicating favorable results but highlighting the importance of careful evaluation of transprosthetic gradients and valve function. ViV TAVR offers promising outcomes but requires meticulous patient selection, procedural planning, and post-procedural management to ensure optimal results.
Keywords
Valve-in-valve transcatheter aortic valve replacement, redo surgical aortic valve replacement, balloon valve fracture, coronary artery obstruction, patient-prosthesis mismatchIntroduction
In recent years, redo surgical aortic valve replacement (SAVR) has remained the gold standard for treating severe symptomatic aortic stenosis (AS) and insufficiency, with bioprosthesis implantation being the preferred method in approximately 80% of cases. Despite an aging patient population and increasing overall risk profiles, the morbidity and mortality associated with isolated aortic valve replacement have decreased [1]. Transcatheter aortic valve replacement (TAVR) has emerged as an effective and less invasive alternative to SAVR, expecially for individuals with symptomatic AS [2]. However, calcification of valve leaflets is primary mechanism responsible for structural degeneration of the valve, with factors such as patient characteristics (younger age, higher body surface index), cardiovascular risk, and to the bioprosthetic valves themselves contributing to an increased risk. While surgical reintervention has been the standard treatment for bioprosthetic AS, valve-in-valve TAVR (ViV TAVR) has gained traction as a viable alternative in recent years [3]. Transthoracic echocardiography (TTE) is useful for assessing patient suitability for TAVR, while transesophageal echocardiography (TEE) can swiftly detect complications during the procedure. Post-procedurally, TTE plays a crucial role in evaluating the function of the prosthetic valve [2].
A comprehensive review of ViV TAVR should encompass pre-procedural considerations, including patient selection and assessment using TTE, intra-procedural techniques and complications detected by TEE, and post-procedural follow-up with TTE to monitor valve function. Echocardiography remains integral throughout the evaluation and management of patients with AS, spanning from pre- to post-procedure for TAVR.
ViV TAVR or redo SAVR?
Given the indispensable role of cardiac tomography (CT) and contrast angiographic examination in diagnosing prosthetic dysfunction and planning/executing the ViV TAVR procedure, the echocardiographer’s role is crucial [4, 5].
The diagnosis of dysfunction due to degeneration primarily relies on echocardiography (as shown in Figure 1), encmpassing morphological evaluation of the prosthesis, calculation of mean gradient, Doppler velocity index (DVi), prosthetic valvular area (PVA) via continuity equation (CE), and assessment of regurgitation. Additional evaluations include 3D study of prosthetic cusps morphology and movement, localization of regurgitation jet (intra-/extra-cardiac), and assessment of residual prosthetic area [6]. Performing TTE for bioprosthetic valves necessitates technical adjustments, as ultrasound velocity changes passing through prosthetic material, potentially distorting images. Adjusting ultrasounds emission gains can mitigate this effect. Reverberations from prosthetic material can obscure visualization, requiring multiple echocardiographic windows including TEE for comprehensive assessment.
ViV TAVR follows standard techniques, preferably via transfemoral vascular access. Choice of TAVR system (balloon-expandable or self-expandable), considers aortic root dimensions (from multislice CT, angiography, and echocardiography) and existing bioprosthesis dimensions and implant technique. Fluoroscopy root angiography with the pigtail catheter in the right coronary sinus determine perpendicular annular plane and cusp alignment [7]. Rapid ventricular pacing (≈ 180 bpm) during angiography reduces cardiac output for device alignment during deployment, typically under general anesthesia.
Resurgical intervention via median sternotomy involved cannulation of ascending aorta, aortic arch or femoral artery, and right atrium or femoral vein for cardiopulmonary bypass, often with systemic normothermia or mild hypothermia (32°C). Intermittent antegrade hematic cardioplegia ensures myocardial protection, with concurrent procedures as needed [8].
The ViV TAVR technique enhances procedural understanding, emphasizing factors like valve sizes/types, degeneration type, valve position, delivery system, and implant height, all influencing procedural outcomes.
Preprocedural aspect
The decision on whether a patient is suitable for ViV TAVR or redo SAVR involves a multidisciplinary team comprising cardiologists, cardiothoracic surgeons, echocardiographers and diagnostic cardiologists [9].
While redo SAVR remains the preferred treatment for younger patients with low to intermediate surgical risk and severe structural prosthetic valve deterioration (SVD), ViV TAVR may be considered for older patients or those with comorbidities at higher reoperation risk [10]. ViV TAVR is typically indicated for patients at high or extreme surgical risk, while those at intermediate or low risk are usually referred for redo SAVR unless anatomical conditions are unfavorable. However, long-term durability data for ViV TAVR in younger patients are limited.
For patients at high risk of coronary artery obstruction with ViV TAVR, referral for redo SAVR is recommended. However, in cases where Bioprosthetic or Native Aortic Scallop Intentional Laceration to prevent Iatrogenic Coronary Artery obstruction (BASILICA) technique is not feasible or when commissural alignment of the valve is necessary to prevent obstruction by one of its commissural posts, redo SAVR may be the preferred option. Additionally, for patients with nonstructural valve dysfunction such as severe patient-prosthesis mismatch (PPM) or paravalvular leak (PVL), repeat SAVR should be considered if balloon valve fracture (BVF) cannot be performed in the SAVR with stent, or when percutaneous treatment of PVL is not feasible [6].
These recommendations highlight the importance of considering various factors, including anatomical considerations, technical feasibility, and the patient’s overall condition, when determining the appropriate treatment approach for patients with aortic valve dysfunction.
Echocardiographic and anatomical-structural parameters play a crucial role in deciding between redo SAVR and ViV TAVR, analyzed in detail in Table 1.
Evaluation of parameters when choosing between redo surgical aortic valve replacement (SAVR) and valve-in-valve transcatheter aortic valve replacement (ViV TAVR)
Parameters/Features | ViV TAVR | Redo SAVR |
---|---|---|
High surgical risk | √ | |
Age > 80 years | √ | |
Concurrent surgical interventions | √ | |
PVL | √ | |
Small ID and PPM | √ | |
Feasibility of BVF | √ | |
Other risk of coronary obstruction, valve malposition and aortic root injury | √ | |
Calcific aortic root or hostile chest | √ | |
Favorable coronary anatomy | √ |
BVF: balloon valve fracture; ID: internal diameter; PPM: patient-prosthesis mismatch; PVL: paravalvular leak
During TAVR, coronary artery ostium obstruction can lead to myocardial ischemia, posing a significant risk. The risk of coronary obstruction is higher in ViV TAVR, expecially in patients with stenosis and a distance ≤ 4 mm between the valve and the coronary ostia, and a cut off ≤ 3 mm is considered high risk [6, 10, 11]. Redo SAVR is preferred to minimize the risk, although the BASILICA technique may be used as an alternative [6]. Other causes of coronary artery obstruction can include a narrow sinotubular junction/sinuses of Valsalva, previous root repair, and supraannular position of the surgical aortic bioprosthesis [11].
Rahimtoola [12] was the first to introduced the concept of PPM, suggesting that it should be considered when the effective area of the bioprosthesis is smaller than that of a normal human valve typically defined as less than 0.65 cm2/m2. When the effective orifice area (EOA) is smaller than expected, it leads to higher transvalvular gradients. The transvalvular pressure gradient (TPG) can be calculated using the equation:
Where:
Q represents transvalvular flow, which depends on cardiac output, itself influenced by body surface area (BSA).
k is a constant.
EOA is the effective orifice area of the valve.
To maintain a low gradient, the EOA must be proportionate to the flow requirement [13]. During redo SAVR the expected EOA, obtained from the values provided by the manufacturers of the bioprostheses and indexed based on the BSA, is typically used. In patients undergoing ViV TAVR, the EOA is measured by the CE using TTE. However, the evaluation of the PPM is not straightforward because the measured EOA seems to overestimate it, seems more accurate and suggests it is a rarer event. The diagnosis of PPM is primarily an echocardiographic one, made using TTE, although TEE may be required to evaluate bioprosthesis morphology and calculate the DVi, particularly when is within the range of 0.25 to 0.40 cm2/m2 [14].
PVL is a condition where there’s a gap between a prosthetic valve and the native annular tissue, allowing blood to leak from one heart chamber to another. The causes of PVL include the native friability of the annulus tissue, calcification of the annulus and endocarditis. PVL occurs more frequently in TAVR than in SAVR [15]. Significant PVL is a contraindication to ViV TAVR [16, 17].
Some authors [18] believe that in degenerated aortic bioprostheses, the echocardiographic evaluation of the mean gradient (which is based on the Bernoulli equation) overestimates the mean gradient compared to the invasive evaluation. This discrepancy is particularly significant in cases of degeneration due to stenosis or in mixed forms, expecially with lower degrees of stenosis. The discrepancy aries because echocardiographic evaluation does not account for pressure recovery and omits non-convective forces and viscosity from gradient calculation for simplification. The pressure gradients across an orifice can be calculated used the simplified Bernoulli equation:
Where:
P1 = inlet pressure.
P2 = Outlet pressure.
ρ = mass density of the blood 1.06 × 103 kg/m3.
V1 = inlet velocity.
V2 = outlet velocity.
In most clinical situations, flow acceleration (
Since both velocity terms are squared and V2 is significantly greater than V1, we can further simplify:
Where V is the maximum velocity of the stenotic jet [19].
Balloon valve fracture
Introducing the concept of BVF involves understanding the internal diameter (ID) of the dysfunctional bioprosthesis. Each prosthetic valve comes in various sizes, determined by the cardiac surgeon during direct inspection. BVF entails the fracture of the stent of the dysfunctional bioprosthesis using a non-compliant balloon inflated to high pressure inside the bioprosthesis until the stent fractures [6]. The advantages of BVF include improved outcomes due to the ability to insert a larger bioprosthesis or achieve more complete deployment of the existing bioprosthesis during ViV TAVR, ultimately enhancing post-procedural hemodynamics and patient outcomes.
Procedural aspects
The approach to ViV TAVR procedures has evolved over the years, with a notable decrease in vascular and access site complications due to increased operator experience and improved complication prediction. Transfemoral access has become more prevalent due to advancements in delivery system technology, leading to areduction in the use of the transapical access. Transapical access carries the disadvantages of requiring a minithoracotomy and ventriculotomy. Percutaneous access through the femoral artery is the most commonly used approach, although other accesses routes such asdirect aortic, subclavian or carotid may also be utilized [10]. During the procedure, guidance techniques vary depending on the chosen approach. Transfemoral procedures typically rely on TTE guidance, while transapical approaches often usefluoroscopic guidance. TEE is utilized to guide the transit of the guide and catheter through the left ventricle (LV), helping to preventing dislocation of the anterior mitral leaflet and subsequent severe insufficiency of the mitral valve. Echocardiographic guidance is crucial throughout the procedure, facilitating the insertion of the TAVR into the dysfunctional bioprosthesis and allowing for real-time evaluation of deployment and final result. Additionally, echocardiography aids in the identification and assessment of any complications that may arise during the procedure.
In relation to interventional procedures and, consequently, to ViV TAVR, it is appropriate to make some considerations regarding radiation exposure and the associated risk. A general consideration concerns radiation exposure in the general population, including those not undergoing medical procedures. The consensus on the optimal use of radiation highlights how, in the USA, average exposure increased from 0.6 mSv in 1987 to 3.2 mSv per person/year in 2006, which is an alarming trend seemingly on the rise. To delve more specifically into a TAVR implantation procedure, the radiation dose in fluoroscopy varies from 12–33 mSv for the transapical procedure to 33–100 mSv for the transfemoral one.
An interventional cardiologist (performing an average of 500 procedures/year) absorbs 10 mSv/year and therefore potentially 300 mSv over the course of their career. However, the interventional cardiologist is not the only exposed medical professional. The echocardiography cardiologist who performs transesophageal examinations during procedures (such as left atrial appendage closure, percutaneous mitral repair, and even ViV TAVR performed transapically) is also highly exposed, being close to the radiation source. McNamara et al. [20] demonstrate that during percutaneous closure of the left atrial appendage, the echocardiographer’s exposure is 10.5 µSv compared to 0.9 µSv for the interventional cardiologist, and during mitral valve repair, it is 1.6 µSv compared to 2.1 µSv.
Radiation damage can be tissue reaction (e.g., cutaneous) and occurs above a threshold at which the lesion occurs (typically within 4–8 weeks) but can also be probabilistic. This DNA damage from radiation exposure, with potential neoplastic complications, may not manifest in the irradiated individual but can be transmitted to descendants. A final consideration concerns gender and age. Women are at higher risk (likely due to the greater vulnerability of breast tissue), as are young individuals, due to their expected longer lifespan and thus the wider period in which the lesion may manifest. Additionally, children with congenital heart diseases are at higher risk, being more exposed to radiation for diagnostic reasons or corrective procedures [20, 21].
The size of the valve used is determined based on measurements obtained from the CT scans and direct assessment. The choice between self-expandable or balloon-expandable valves is typically based on the preferences and experience of the operator [9]. These advancements in approach selection and guidance techniques have contributed to improved outcomes and reduced complications in ViV TAVR procedures.
When ViV TAVR becomes necessary and the ID is very small, the PPM can pose a significant complication that needs to be minimized. Self-expanding bioprostheses with a supra-annular design are considered a viable alternative. In such cases and have shown to provide hemodynamic improvements [22]. Additionally, achieving a higher valve deployment within specific ranges can offer benefits. For example, for CoreValve system (CoreValve Revalving System, Medtronic, Minneapolis, MN, USA) an implantation depth between 0 mm and 5 mm is recommended, while for Sapien system (Edwards Lifesciences, Irvine, California, USA), it ranges from 0 mm to 2 mm to 3.5 mm (0–10% device frame). However, it’s crucial to note that this approach may carry an increased risk of coronary obstruction and valve embolization [23, 24]. The deployment of the valve during ViV TAVR should be carefully managed, as both excessively high or low deployments can significantly impact procedural outcomes, even in the absence of PPM-related issues [19], as shown in Table 2. Balancing these considerations is essential for optimizing the success of the procedure and minimizing complications.
Consequences of a higher or lower valve deployment
Higher bioprosthesis deployment | Lower bioprosthesis deployment |
---|---|
Perivalvular regurgitation | Coronary ostial obstruction |
TAVR embolization | Interference with the anterior mitral leaflet |
Damage to the conduction tissue |
BVF is another method used to minimize PPM during ViV TAVR procedures. This technique involves fracturing or lengthening the valve ring, which improves the seal of the valve, reduces the possibility of leaks, and decreases the transprosthetic gradient. BVF is performed using a non-compliant balloon such as True Dilatation or Atlas Gold (Bard, Murry Hill, NJ, USA); along with other equipment including a tap and high-pressure hose; a deflator; a 60 mL syringe with a diluted contrast medium. During BVF, rapid ventricular stimulation is initiated, and the non-compliant balloon is inflated with 60 mL of contrast medium until the fracture occurs. Confirmation that BVF is observed through angiographic changes in the waistline of the balloon and the geometry of the valve, along with a dop deflator pressure drop in deflator pressure and an audible click indicating fracture. Determining the size of the balloon for BVF during ViV TAVR procedures involves consideration of several factors: valve type, ID, desired post-procedure diameter, anatomy of the aortic root, height of the coronary arteries [25]. Timing of BVF is crucial. Performing BVF before ViV TAVR procedure may result in better closure of the valve flaps and hemodynamic instability. On other hand, performing BVF after the procedure may yield better outcomes, although there is a risk of damaging the bioprosthesis [26, 27].
Coronary obstruction is another risk during ViV TAVR procedures, particularly when the bioprosthesisflaps moves towards the sinus of Valsalva, impeding diastolic perfusion of the coronary ostium. Stented bioprostheses like Mitroflow (Sorin Group USA, Inc., Arvada, CO) and Triflecta (St. Jude Medical, St. Paul, MN) may further increase this risk. In such cases, using a self-expanding lower-profile balloon valve with a smaller diameter can reduce the risk, but post procedural gradients must be carefully considered [10, 11]. Preventive strategies outlined in registries like the VIVID registry [28] can also help mitigate the risk of coronary obstruction. These strategies (indicated in Table 3) consider factors such as bioprosthesis type, the coronary height, sinus of Valsalva dimensions, and the distance between the fully expanded valve ring and the coronary ostium [29]. By carefully considering these factors and employing appropriate preventive measures, the risk of complications during ViV TAVR procedures can be minimized.
Strategy to prevent coronary artery obstruction
Strategy | Advantages |
---|---|
Lower implantation of the bioprosthesis | Preferring self-expandable valves for the possibility of re-capturing |
Chimney snorkel stenting technique | Wiring the coronary artery and putting a stent on standby |
Orthotopic snorkel stenting technique | Re-cannulation and wiring after THV release |
BASILICA | Laceration of surgical leaflets with an electrified guidewire to create a communication between the sinus and neo-sinus |
BASILICA: Bioprosthetic or Native Aortic Scallop Intentional Laceration to prevent Iatrogenic Coronary Artery obstruction; THV: transcatheter heart valves
Commissural alignment plays a crucial role in TAVR procedures as shown in Figures 2 and 3. It refers to the correct positioning of the valve prosthesis so that its commissures align with those of the native valve. This alignment is crucial because it facilitates future access to the coronary arteries and can also influence other aspects such as valve durability, risk of valve thrombosis, structural valve deterioration, and the possibility of future TAVR procedures. Commissural alignment can facilitate coronary access after TAVR by reducing the risk of overlap between the prosthesis commissures and coronary ostia, which could complicate the placement of catheters during subsequent coronary procedures. However, commissural alignment alone may not always guarantee optimal positioning of the prosthesis for coronary access, as other factors such as asymmetry of the native valve cusps and eccentricity of coronary ostia may influence such access. Improper alignment could increase the risk of complications such as aortic regurgitation, valve thrombosis, and structural valve deterioration. However, evidence regarding the association between commissural alignment and these complications is still under research and not entirely clear. Commissural alignment may be important in ViV TAVR procedures as proper commissural alignment can reduce the risk of obstruction of the coronary arteries caused by the leaflets of the previous valve, thereby improving the safety and effectiveness of the procedure [30].
Postprocedural aspects
There were low levels of mortality, acceptable complication rates, and clear improvement in valvular hemodynamics with a low risk of moderate to severe aortic regurgitation. These results indicate that clinical outcomes in real-world settings fall acceptable limits, justifying this treatment as the preferred approach for these patients [31]. The PARTNER 2 studies [32–34] established the groundwork and regulations for subsequent studies that further evaluated and demonstrated the long-term efficacy of the ViV TAVR procedure. Post ViV TAVR mortality rates are reported as 1.3% at 30 days, 31.2% at 2 years and 42.9% at 36 months based on the type of valve used [35] or 50.6% at 5 years for all causes [32].
Regarding the duration of clinical and hemodynamic benefits [such as improvement in New York Heart Association (NYHA) class, increase in EOA, reduction in the mean gradient and increase in DVi], transprosthetic mean gradient after ViV TAVR varies between 12 mmHg and 26 mmHg [35]. A post-procedural transprosthetic mean gradient > 20 mmHg is considered a failure [36] as shown in Figure 4.
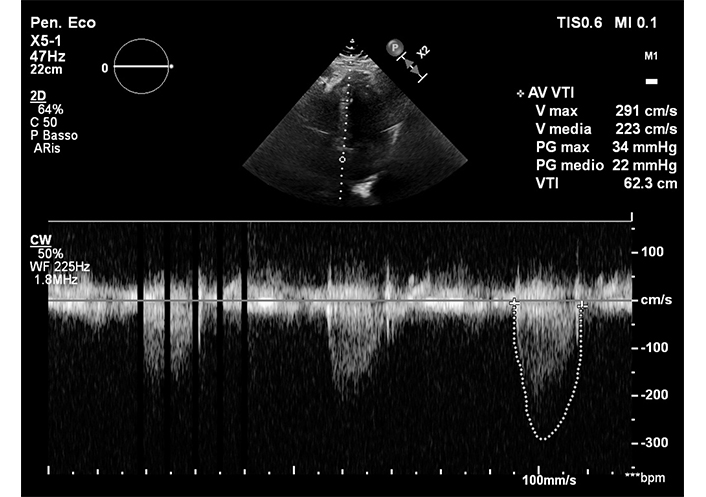
Post-procedure transthoracic echocardiographic check of ViV TAVR. ViV TAVR: valve-in-valve transcatheter aortic valve replacement
Debate persists regarding post-procedural mean transprosthetic gradients, as those post ViV TAVR tend to be higher than those detectable after TAVR on a native aortic valve, and on the duration of improvement in mean gradient and valve area. Some studies indicate persistent persistent positive result at 2 months, 1 year [9] and 5 years [32], while others show a tendency for the transprosthetic mean gradient to rise above 20 mmHg at 3 years [35], sometimes exceeding 30% [31]. Outcome variability depends on factor such as the type of valve used for ViV TAVR. Evaluating the ID is crucial, as it may differ from the size declared by the manufacturer and significantly impacts long-term outcomes [37]. It has been demonstrated that for an ID < 19 mm, the result is unfavorable, leading to a high transprosthetic mean gradient and related complications [9]. Additionally, patients undergoing TAVR for valve stenosis had a less favorable long-term outcome than those with initial regurgitation [10]. However, no significant differences were found between ViV TAVR performed with auto or balloon expandable valves [35]. It is important to consider the discrepancy between the evaluation of the post-gradient between angiography and echocardiography. Careful evaluation of the role of “pressure recovery” is necessary keeping in mind that hemodynamic and invasive evaluations are more precise [18]. Compared to native valve TAVR, a clinically relevant feature of ViV TAVR is the lower incidence of leaks, annulus, or mitroaortic continuity lesions, and conduction defects, as the dysfunctional bioprosthesis frame protects the underlying structures during the procedure [6]. The size provided by the manufacturer is important, but there is also an ID smaller than the size of the bioprosthesis that can be measured with CT. This determines the good long-term outcome of the ViV TAVR procedure. It has been demonstrated [32] that for an ID less than 19 mm, the result will be unfavorable with the restoration of a high transprosthetic mean gradient and the related complications.
Conclusions
In summary, the landscape of treating severe symptomatic AS and insufficiency has evolved significantly, with redo SAVR remaining the standard for younger patients with lower surgical risk, while TAVR offers a less invasive option, particularly for older or higher-risk patients. The choice between redo SAVR and ViV TAVR involves careful consideration of patient factors and anatomical considerations.
ViV TAVR has emerged as a viable alternative to redo SAVR, especially for high or extreme surgical risk patients. Echocardiography plays a crucial role throughout the patient’s journey, from pre-procedure assessment to post-procedure follow-up. Procedural considerations include access site selection, valve choice, and deployment techniques, with careful management of complications like coronary artery obstruction and PPM.
Post-procedural follow-up is essential to monitor valve function and detect complications early. ViV TAVR offers improved outcomes and reduced morbidity compared to redo SAVR but requires further research to optimize long-term durability and procedural techniques for optimal patient outcomes.
Abbreviations
AS: | aortic stenosis |
BASILICA: | Bioprosthetic or Native Aortic Scallop Intentional Laceration to prevent Iatrogenic Coronary Artery obstruction |
BSA: | body surface area |
BVF: | balloon valve fracture |
CE: | continuity equation |
CT: | cardiac tomography |
DVi: | Doppler velocity index |
EOA: | effective orifice area |
ID: | internal diameter |
LV: | left ventricle |
NYHA: | New York Heart Association |
PPM: | patient-prosthesis mismatch |
PVA: | prosthetic valvular area |
PVL: | paravalvular leak |
SAVR: | surgical aortic valve replacement |
SVD: | structural prosthetic valve deterioration |
TAVR: | transcatheter aortic valve replacement |
TEE: | transesophageal echocardiography |
TPG: | transvalvular pressure gradient |
TTE: | transthoracic echocardiography |
ViV TAVR: | valve-in-valve transcatheter aortic valve replacement |
Declarations
Author contributions
MFC: Conceptualization, Writing—original draft, Validation, Supervision. GD: Formal analysis, Investigation. FC: Formal analysis, Investigation. LS: Formal analysis, Investigation, Methodology, Visualization, Writing—review & editing. All authors read and approved the submitted version.
Conflicts of interest
The authors declare that they have no conflicts of interest.
Ethical approval
The images are part of the SE 2030 study approved by the institutional ethics committees (291/294/295-Comitato Etico Lazio-1, 8 March 2021).
Consent to participate
Informed consent from the patients were obtained.
Consent to publication
Not applicable.
Availability of data and materials
Relevant data can be provided on reasonable request.
Funding
Not applicable.
Copyright
© The Author(s) 2024.