Abstract
Lysosomal hydrolases were once considered effectors of the waste disposal system of the cell, the endo-lysosomal system. However, they are now recognized as highly selective enzymes, which can modulate the function of several substrates, contributing to essential homeostatic and pathological cellular processes. There are more than 50 different lysosomal hydrolases that display optimal activity in the pH present in the acidic cellular compartment but can also be found in other cellular locations. They can work alone or in cooperation with other proteases building signaling pathways or amplification cascades. In the context of liver fibrosis lysosomal hydrolases, especially cysteine cathepsins have been described to participate in several fundamental cellular events contributing to the development, progression, perpetuation, and resolution of liver fibrosis. This paper comprehensively reviews the current knowledge on the contribution of lysosomal hydrolases to liver fibrosis.
Keywords
Lysosome, protease, cathepsin, liver fibrosisIntroduction
Lysosomes are specialized membrane-enclosed cytoplasmic organelles responsible for the degradation and recycling of several macromolecules such as proteins, carbohydrates, lipids, and nucleic acids. Endocytic, autophagic, and phagocytic pathways are responsible for the transportation of the cargos that feed the lysosomes. Once in the lysosomes, more than 50 different hydrolases oversee the processing of the different macromolecules for their subsequent reutilization. Lysosomes have been classically considered the “garbage disposals” of the cell. However, this paradigm has been overturned in recent decades, and the participation of the lysosomes in several cellular processes such as cell adhesion and repair, immunity, metabolic signaling, gene regulation, and migration has been reported [1]. In addition, lysosomal hydrolases, which were considered strictly functional and restricted to the acidic lysosomal lumen, are now known to display activity in diverse cellular locations, even at neutral pH for a limited period [2], such as the cytosol [3], the pericellular surroundings (PCSs) [4] and even the nuclei [5].
Lysosomal hydrolases are proteolytic enzymes or proteases that display their greatest stability at the acidic pH present within these organelles. Previously considered random catalytic devices, proteases are currently recognized to cleave specific substrates in an efficient and highly selective manner through limited proteolysis. In addition, it is important to acknowledge that proteases do not operate alone but in regulatory circuits and signaling cascades [6]. As a result, targeted proteolytic processing of substrates finely tunes several cellular processes such as apoptosis, cell-cycle, cell migration, immune cell activation, and extracellular matrix (ECM) remodeling among others [7]. Proteolytic impairment or dysregulation is responsible for most lysosomal storage disorders (LSDs) [8] and contributes to other human pathologies including neurodegenerative disorders [9], cancer [6], arthritis [10], and liver disease [11]. Several excellent revisions have been published on the contribution of lysosomal hydrolases to the progression of several pathological conditions [12]. However, no specific revision has summarized the specific role of lysosomal hydrolases in the development, progression, and resolution of liver fibrosis, something this review aims to address.
Lysosomal hydrolases
Cathepsins are the most abundant lysosomal hydrolases present within the lysosome but other types such as naspin A and asparagine endopeptidase (AEP) can also be found. Naspin A is mainly expressed in kidney and lung and its expression has only been detected in liver during liver cancer [13]. AEP expression has been reported in liver but its role during liver disease progression has not yet been explored [14]. For this reason, this review will mainly focus on the role of cathepsins in liver fibrosis.
The name cathepsin derives from the combination of Greek verbs cata- (digest) and hepsein (boil), defining their degradative capacity. All cathepsins are synthesized as inactive precursors in the ribosome including an N-terminal signal peptide to route them to the endoplasmic reticulum (ER), where simultaneous N-linked glycosylation and cleavage of the N-terminal peptide occurs. Phosphorylation of the high-mannose residue glycans in the Golgi causes the generation of mannose-6-phosphate (M6P) residues, which are recognized by the M6P receptors present in the endo/lysosomes and directs them to these cellular compartments. Triggered by the acidic pH of the lysosomes, cathepsins undergo cleavage of the pro-region, thought to act as an auto-inhibitor. This processing results in the generation of a double or a single chain, which are both active forms of the enzyme [2]. Alternative routes to reach the lysosomes [15] and the extracellular space [16] can occur in the absence of M6P residues.
There are three main classification systems for proteases currently in use, which group them according to either the amino acid present in their catalytic site (metallo, serine, cysteine, aspartic, threonine, glutamic, and asparagine) [17]; their catalytic reaction (endopeptidases, omega-peptidases, exopeptidases, aminopeptidases, carboxypeptidases, dipeptidyl-peptidases, tripeptidyl-peptidases, peptidyl-dipeptidases, and dipeptidases; https://www.qmul.ac.uk/sbcs/iubmb/) or their sequence homology and molecular structure (http://merops.sanger.ac.uk) [18]. The most commonly used classification for cathepsins is based on their catalytic site: aspartate (cathepsin D and E), cysteine [cathepsin B, C/dipeptidyl peptidase 1 (DPP1), F, H, K, L, O, S, V, W, and Z/X] and serine (cathepsin A and G). Cathepsins exhibit endo and exopeptidase activity and are ubiquitously distributed across tissues, except for cathepsin K (mainly expressed in osteoclasts and epithelial cells) and cathepsin S, E, and W (immune cells). The proteolytic activity of cathepsins is subjected to several regulatory steps at transcriptional [19], epigenetic [20], translational [21], and post-translational [22] levels. In addition, besides the pH, lysosomal hydrolases are also regulated by endogenous inhibitors such as cystatins and stefins [23]. This is essential as cathepsins contribute to several homeostatic cellular events, such as apoptosis, autophagy, chemotaxis, and antigen presentation. Hence, dysregulation of cathepsin activity is linked to the development and progression of several human diseases, ranging from liver disease to cancer. The following sections will discuss the main contributions of cathepsins to the different cellular events responsible for the development, progression, and resolution of liver fibrosis.
Role of cysteine hydrolases in liver fibrosis
Cysteine cathepsins are the largest cathepsin family, it encompasses 11 members (B, C, F, H, K, L, O, S, V, W, and Z/X) and shares the characteristic of presenting a cysteine in their catalytic site. Most cysteine cathepsins are ubiquitously expressed in tissues (B, C, F, H, L, O, V, and X). Conversely, cathepsin K, W, and S have a more specific tissue distribution. While cathepsin K is highly expressed in osteoclasts, cathepsin W [CD8+ lymphocytes and natural killer (NK)] and S [B-cells, dendritic cells (DCs), antigen presenting cells (APCs)] are expressed in immune cells.
Among the lysosomal hydrolases, cysteine cathepsins are the most extensively studied family of proteases in liver fibrosis and have been described to contribute to several important biological processes fundamental for its development and progression, which will be described in more details in the following subsections.
Cysteine hydrolases as effectors of apoptotic cell death during liver fibrosis
The role of cysteine cathepsins, specially cathepsin B, in the canonical apoptotic pathway upstream the mitochondria, is one of the most extensively reported. During apoptosis, the permeabilization of the lysosomal membrane enables the translocation of cathepsin B into the cytosol, where it can proteolytically process members of the B-cell lymphoma protein-2 (Bcl-2) family influencing their activity. The exact mechanism triggering lysosomal membrane permeabilization (LMP) is not fully understood, but two mechanisms have been proposed for the release of cathepsin B from the lysosome into the cytosol. The first one involves the activation of the c-Jun N-terminal kinase (JNK) signaling pathway by tumor necrosis factor-related apoptosis-inducing ligand (TRAIL), which triggers the activation of Bim to induce Bax-mediated lysosomal permeabilization [24]. The second one proposes a signaling cascade in which the factor associated with neutral sphingomyelinase activation (FAN) is responsible for the release of cathepsin B by LMP upstream of caspase-8/Bid [25]. In the context of fibrosis induced by bile duct ligation, genetic (cathepsin B knock-out) and pharmacological (R-3032) inhibition of cathepsin B resulted in a reduction in liver injury, inflammation, and fibrosis caused by a decrease in the number of apoptotic hepatocytes [26]. In agreement with these results, cathepsin B knock-out mice displayed higher resistance to hepatocyte apoptosis and increased survival, when subjected to a model of tumor necrosis factor-α (TNF-α)-induced acute liver damage, driven by decreased activation of the canonical apoptotic pathway [26–28]. More recent evidence attributed the cytoprotective effect of albumin, against TNF-α-induced liver inflammatory injury, to its effect preventing LMP and cathepsin B leakage from the lysosome, thereby reducing apoptosis triggered by the release of cytochrome c from the mitochondria [29].
Cysteine hydrolases as modulators of the phenotypical transdifferentiation of hepatic stellate cell
Cysteine cathepsins also contribute to fibrosis development by modulating the phenotypic transformation of quiescent hepatic stellate cell (HSC) into myofibroblasts-like cells. Evidence in the literature suggests an opposite role for cathepsin B depending on its cellular location during the activation of HSC. On the one hand, intracellular inhibition of cathepsin B in activated HSC, using the cell-permeable inhibitor (Ca074-Me), caused defective phosphorylation of Akt after platelet-derived growth factor (PDGF) stimulation, resulting in a reduction of their proliferative and migratory capacity. In agreement, treatment with Ca074-Me in a model of intraperitoneal carbon tetrachloride administration led to a decrease in liver inflammation and fibrosis [30]. On the other hand, extracellular cathepsin B has been reported to be responsible for the proteolysis of PDGF receptor-β during N-acetyl-L-cysteine (NAC) desensitization of HSC towards PDGF-BB, ultimately resulting in a reduction of liver fibrosis in a rat model of thioacetamide [31].
Several candidates have been proposed to activate cathepsin B in activated HSC, including cathepsin D [30] and acidic sphingomyelinase (ASMase) [32]. While paracrine processing between different cathepsins is an often-described mechanism of activation, the direct mechanism of cathepsin B activation by ASMase has not been defined yet. Despite the axis ASMase/cathepsin B not being fully understood, several reports have demonstrated that it is important for the phenotypical transformation of HSC. Hierarchy between ASMase and cathepsin B was established by reports showing that while ASMase inhibition had an effect over cathepsin B, cathepsin B inhibition did not have an effect over ASMase, suggesting that ASMase is upstream cathepsin B. To that respect, pharmacological inhibition (imipramine or amitriptyline) of ASMase decreased cathepsin B levels and reduced HSC proliferation, resembling the effect of Ca074-Me [32]. Interestingly, whereas partial ablation of ASMase, displayed by ASMase heterozygous mice, resulted in decreased liver fibrosis after both carbon tetrachloride administration and bile duct ligation, total genetic ablation exhibited in ASMase knock-out mice led to increased liver fibrosis and increased levels of cathepsin B, due to compensatory mechanisms [33]. These reports demonstrated an important and dependent relationship between ASMase and cathepsin B in activated HSC.
In addition to cathepsin B, other cysteine cathepsins have also been proposed to modulate the activation of HSC within the nuclei. Nuclear cathepsin H has been linked to the degradation and stabilization of class IIa histone deacetylases, thus its absence results in the modulation of metalloproteinases gene expression and the activation of HSC [34]. Furthermore, nuclear cathepsin F has been reported to transcriptionally regulate the activation of HSC markers in rat by an unknown mechanism [35].
Cysteine hydrolases as mediators of autophagy in liver fibrosis
Lysosomal hydrolases have been reported to participate in autophagy in several diseases and liver fibrosis is no exception. Recent studies have proposed an autophagic-cathepsin B-Nod-like receptors containing pyrin domain 3 (NLRP3) signaling pathway in HSC as a contributor to liver fibrosis development. Briefly, autophagic flux promotes the release of cathepsin B into the cytosol, which activates NLRP3, alone [36] or in conjunction with reactive oxygen species (ROS) [37], triggering HSC activation and liver fibrosis induced by long-term exposure to arsenic (NaAsO2) [36] or upon lipopolysaccharide (LPS)/ATP stimulation [37]. Besides, lysosomotropic compound GNS561 causes defective autophagic processing in HSC, due to impaired cathepsin activity, resulting in deficient transforming growth factor-β1 (TGF-β1) maturation, increased HSC apoptosis and attenuation of liver fibrosis in a rat model of diethylnitrosamine [38].
Cysteine hydrolases as contributors to the inflammatory response associated with liver fibrosis
Cathepsins have also been implicated in the inflammatory response contributing to the development of liver fibrosis. Cytosolic cathepsin B participates in the cytotoxic response mediated by TRAIL in NK/natural killer T (NKT) cells during liver fibrosis after bile duct ligation [39]. However, the exact function of cathepsin B in NK/NKT cells has not been described yet. In addition, cathepsin B and S have been proposed to regulate sirtuin-1, an NAD+-dependent protein deacetylase that modulates nuclear factor-κB (NF-κB) transcription factor. In this study, inhibition of cathepsin B and S decreased NF-κB dependent inflammation and fibrosis with an increase in sirtuin-1 [40]. Finally, evidence suggests that interferon-γ (IFN-γ) upregulates cathepsin S expression in HSC, which processes CD74 contributing to HSC antigen presentation [41].
Cysteine hydrolases as remodelers of the ECM during liver fibrosis
The most evident but probably least accurately explored function of lysosomal hydrolases in liver fibrosis is their role remodeling the ECM [42]. The collagenolytic activity of cathepsins in patients with cirrhosis and chronic hepatitis was described as early as the 80s [43]. Since then, correlations between the level of cathepsin L [44, 45] in plasma with the development of liver fibrosis and cirrhosis have been made, suggesting that the collagenolytic activity of cathepsins contributes to disease progression.
Role of aspartyl and serine hydrolases in liver fibrosis
Aspartic and serine cathepsins are smaller families than cysteine cathepsins and display an aspartate or serine amino acid in their catalytic site, respectively. Both families have two members, cathepsin D and E are aspartyl hydrolases and cathepsin A and G are serine hydrolases. As cathepsin E and G are mainly expressed in immune cells, for that reason this section will focus on the role of cathepsin D and A in liver fibrosis.
Both cathepsin D and A have been far less studied than other cathepsins, such as cathepsin B, and their participation in the different cellular processes that contribute to liver fibrosis development is unclear. Similarly to cathepsin B, cathepsin D has been reported to participate in the canonical apoptotic pathway, upstream mitochondria, after TNF-α stimulation. Evidence suggests that cathepsin D proteolytically cleaves Bid into truncated Bid (t-Bid), resulting in the release of cytochrome c from mitochondria and the activation of the effector caspases, culminating in apoptosis [46]. Cathepsin D has also been shown to activate cathepsin B in HSC in a paracrine way, contributing to HSC activation [30]. Furthermore, a positive correlation between cathepsin D and autophagy with the ductular reaction (DR) has been found in human cirrhotic patients, suggesting cathepsin D could play a role in autophagy in the DR associated with cirrhosis [47]. Finally, cathepsin A has also been related to autophagy, as in vitro evidence in isolated lysosomes proposes that cathepsin A degrades lysosome associated membrane protein 2a (LAMP2a), restricting the rate of chaperone-mediated autophagy [48].
Potential therapeutic interventions based on lysosomal hydrolases
Experimental therapeutic approaches to slow-down liver fibrosis progression using cathepsin inhibitors have been reported in the literature but none of them have moved forward into clinical trials yet. The deeper understanding of the biological roles that cysteine cathepsins play during liver fibrosis, in comparison with aspartyl or serine cathepsins, has translated into more effective experimental approaches to reduce fibrosis using pharmacological inhibitors in vivo. To that respect, the use of cathepsin B inhibitors has been particularly successful, resulting in a decrease of experimental liver fibrosis from different aetiologies: CCl4 chronic administration [30, 33, 40], bile duct ligation [26, 33], and high-fat choline-deficient (HFCD) feeding [40] in mouse. In addition, cathepsin B inhibitor has also been reported to decrease TNF-α-induced liver damage, reducing hepatocyte apoptotic injury [28]. A reduction in hepatocyte apoptosis can potentially also have a positive effect during fibrosis by reducing liver damage and the inflammatory response that feeds into the activation of HSC. For all of these reasons, cathepsin B seems to be the best candidate within the lysosomal hydrolases for the development of cathepsin-based drugs for the treatment of liver fibrosis. However, as cathepsin B plays a multifaceted role in the development of liver fibrosis contributing to different biological processes in different cell types (Table 1 and Figure 1), therapeutic interventions need to be carefully considered to avoid undesirable side effects.
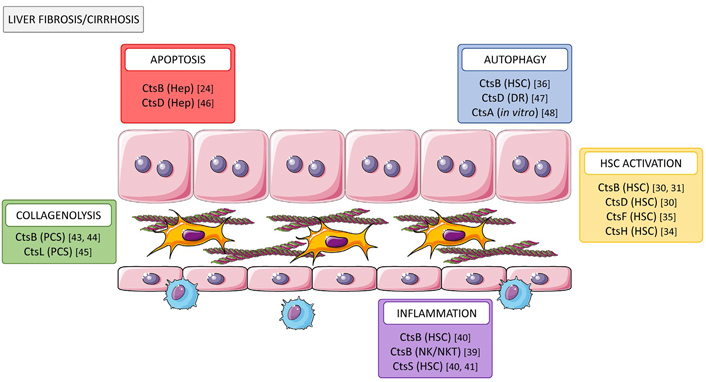
Role of cathepsins in liver fibrosis/cirrhosis. Cathepsins participate in multiple cellular processes contributing to fibrosis progression through increasing hepatocyte cell death (CtsB and D), tissue collagenolytic activity (CtsB and L), autophagy (CtsB and D), and activation (CtsB, D, F, and H) of HSC and promoting inflammation (CtsB and S). Cts: cathepsin; Hep: hepatocyte
Note. This figure contains (modified) images from Servier Medical Art by Servier (https://smart.servier.com). CC BY 3.0.
Biological functions of lysosomal hydrolases during liver fibrosis
Name(Family, MEROPS ID) | Biological process modulated during liver fibrosis | ||||
---|---|---|---|---|---|
Apoptosis | HSC activation | Autophagy | Inflammation | ECM remodeling | |
Cathepsin A(Ser, S10.002) | Degradation of LAMP2a restricting chaperone-mediated autophagy in vitro [48] | ||||
Cathepsin B(Cys, C01.060) | Proteolytic processing of members of the Bcl-2 family in hepatocytes [24] | Modulation of pAkt in HSC after PDGF stimulation contributing to their proliferative and migratory capacity [30] | Cytosolic cathepsin B, released by autophagic flux, triggers activation of NLRP3 in HSC contributing its activation [36] | Participation in the cytotoxic response mediated by TRAIL in NK/NKT cells by an unknown mechanism [39] | Collagenolytic activity by an unknown mechanism [43, 44] |
Proteolysis of PDGF receptor-β contributing to PDGF-BB desensitization in HSC [31] | Modulation NF-κB dependent inflammation in HSC via sirtuin-1 regulation [40] | ||||
Cathepsin D(Asp, A01.009) | Proteolytic cleavage of Bid into t-Bid, upstream mitochondria, triggered by TNF-α in hepatocytes [46] | Paracrine activation of cathepsin B in HSC contributing its activation [30] | Correlation of cathepsin D and autophagy during DR in cirrhosis by an unknown mechanism [47] | ||
Cathepsin F(Cys, C01.018) | Transcriptional regulation of markers of HSC activation by an unknown mechanism [35] | ||||
Cathepsin H(Cys, C01.040) | Modulation of metalloproteinases gene expression in HSC via stabilization of class Iia histone deacetylases [34] | ||||
Cathepsin L(Cys, C01.032) | Collagenolytic activity by an unknown mechanism [44] | ||||
Cathepsin S(Cys, C01.034) | Modulation of NF-κB dependent inflammation in HSC via sirtuin-1 regulation [40] | ||||
Processing of CD74 contributing to antigen presentation in HSC [41] |
pAkt: phosphorylated Akt; -: not applicable
Despite cathepsin B apparent suitability for drug development for liver fibrosis, growing knowledge and understanding of the participation of other cathepsins and their inhibition in other liver diseases [11] might provide new insights into their potential use as therapeutics for liver fibrosis. Finally, as cathepsins also play important roles in other diseases such as severe acute respiratory syndrome coronavirus 2 (SARS-CoV-2) infection and cancer, research done in these disciplines may render novel cathepsin inhibitors [49, 50], which could potentially be repurposed for their use as liver fibrosis treatments.
Conclusions
Our understanding of lysosomal hydrolases has shifted over the last decades from waste-bag effectors to multipurpose enzymes that play important roles in several cellular homeostatic and pathological processes. In the context of liver disease, the contribution of lysosomal hydrolases to the development, progression, and resolution of liver fibrosis is no longer contested. Lysosomal hydrolases are expressed in both liver resident cells and infiltrating cells, and participate in apoptosis, activation of HSC, inflammation, autophagy, and ECM remodeling. Considering the variety of cellular processes that lysosomal hydrolases influence, their expression in several cell types and their various cellular locations, potential therapies need to be carefully designed to ensure maximal effectiveness with minimal side effects. Despite our increased knowledge on the basic functions of lysosomal hydrolases in liver fibrosis, their specific substrates and proteolytic networks are still unknown. Thus, it is essential to unravel the proteolytic signature of liver fibrosis and its contribution to disease progression in order to design novel and targeted therapies based on lysosomal hydrolases for the treatment of liver fibrosis.
Abbreviations
ASMase: | acidic sphingomyelinase |
DR: | ductular reaction |
ECM: | extracellular matrix |
HSC: | hepatic stellate cell |
LMP: | lysosomal membrane permeabilization |
M6P: | mannose-6-phosphate |
NF-κB: | nuclear factor-κB |
NK: | natural killer |
NKT: | natural killer T |
NLRP3: | Nod-like receptors containing pyrin domain 3 |
PCSs: | pericellular surroundings |
PDGF: | platelet-derived growth factor |
TNF-α: | tumor necrosis factor-α |
TRAIL: | tumor necrosis factor-α-related apoptosis-inducing ligand |
Declarations
Acknowledgments
We would like to sincerely acknowledge all the authors, cited or not, whose work has contributed to the advancement of the research field.
Author contributions
MFF: Writing—original draft. PRB: Writing—original draft. JCP: Writing—original draft. AM: Writing—original draft, Conceptualization, Visualization, Supervision, Funding acquisition, Resources, Project administration, Writing—review & editing.
Conflicts of interest
The authors declare that they have no conflicts of interest.
Ethical approval
Not applicable.
Consent to participate
Not applicable.
Consent to publication
Not applicable.
Availability of data and materials
Not applicable.
Funding
AM’s research is supported by grants [RTI2018-097475-A-100] and [PID2021-123652OB-I00] funded by MCIN/AEI/10.13039/501100011033 and by “ERDF A way of making Europe”, by the “European Union”. Both MFF and PRB salaries were supported by FPU fellowships ([FPU20/01367] and [FPU19/05357], respectively) awarded by the Ministry of Universities, Spain. The funders had no role in study design, data collection and analysis, decision to publish, or preparation of the manuscript.
Copyright
© The Author(s) 2023.