Abstract
The liver, characterized by a unique metabolic and immunosuppressive environment, is also the organ to which invasive malignant cells of many different cancer types most frequently metastasize. The reasons for this organ-specific metastatic process have been investigated for decades. This review first provides an overview of recent breakthroughs in this field, introducing intercellular communication between circulating tumor cells and the heterogeneous cell populations of the liver, and modifications to the extracellular matrix (ECM). Subsequently, to improve the understanding of the molecular mechanisms involved in the metastasis of colorectal cancer to the liver, the second leading cause of cancer-related mortality, the recent literature on this question was analyzed. Among the various parameters involved, the mechanisms behind the activation of hepatic stellate cells, proteins inducing ECM remodeling, specific genomic features of liver metastases, metabolic rewiring, and characteristics of stromal-enriched microenvironments were discussed. To provide more insights into the molecular determinants of liver metastatic colonization, important findings reported on a set of mitochondrial proteins were addressed, the relative abundance of which changed in the liver during the progression stage of an aggressive experimental model of peritoneal malignant mesothelioma in immunocompetent rats. Based on previous studies cross-comparing the liver proteomes from curcumin-treated tumor-bearing rats/untreated tumor-bearing rats/normal rats, data from the literature were reviewed for 25 mitochondrial proteins of interest. Their role in lipid metabolism, heme biosynthesis, the electron transport chain, small molecule transport, mitochondrial dynamics, the tricarboxylic acid cycle, and protection against oxidative stress were analyzed in the context of both cancer and non-malignant liver diseases.
Keywords
Cancer, liver metastasis, mitochondrial biomarkers, metabolic reprogramming, liver metabolism, lipid metabolism, proteomics, malignant peritoneal mesotheliomaIntroduction
The liver is the organ to which invasive malignant cells most frequently metastasize for most cancer types, and the mechanisms orchestrating the formation of the hepatic metastatic niche are a permanent field of investigation [1]. About thirty years ago, pioneering studies used different murine models to elucidate the reasons for this organ-specific metastatic process [2]. More recently, new strategies with simple biomaterial- or microfluidics-based co-cultures have tried to summarize this complex microenvironment to better understand the interactions between cancer cells and the stroma at the metastatic site [3].
Circulating tumor cells (CTCs) play a crucial role in this process, and considerable improvements have been made in identifying their molecular features and decreasing their detection levels in peripheral blood for diagnostic purposes [4]. However, this process is also related to the specificities of the liver vasculature system, this organ receives blood from two sources, the hepatic artery and the portal vein, with important immunological consequences [5]. As the main contributor is the portal vein [6], the hepatic sinusoids are directly exposed to CTCs emerging from primary malignant tumors developing in the gastrointestinal tract. The fenestrated vasculature that characterizes the liver [5] also explains why CTCs easily exit the vessels and preferentially colonize this organ [3]. The different steps of this colonization have been remarkably deciphered and illustrated, emphasizing the role of complex interactions between tumor cells and a great diversity of highly specialized resident cells, among which hepatocytes play an important role [6, 7]. However, once CTCs exit the circulation at their metastatic site, they also need to alter their metabolism to adapt to the new environment in the host organ [8], and only a small percentage of them can colonize and form metastatic lesions [9]. In this process, morphological and molecular adaptations play determinant roles, through changes in the mechanical properties of selected CTCs, an increased rat sarcoma virus/mitogen-activated protein kinase/extracellular-signal-regulated kinase (Ras/MAPK/ERK) signal output having been observed to be associated with this event [10].
In addition to its anatomical location and organization, the liver presents a unique metabolic and immunosuppressive environment [5] that, when affected, can promote the development of a premetastatic niche through intercellular communication between primary sites of malignant transformation and the heterogeneous cell populations present in the liver environment [6]. In this field, important breakthroughs identified 1) tumor necrosis factor-alpha (TNF-α) and transforming growth factor-beta (TGF-β) released by activated Kupffer cells (KCs), 2) trans-differentiation of hepatic stellate cells (HSCs) into myofibroblasts producing collagens and matrix metalloproteinases (MMPs), and 3) surface receptors expressed by liver sinusoidal endothelial cells (LSECs), such as intercellular adhesion molecule 1 (ICAM-1), C-type lectin domain family 4 member M (CLEC4M), and vascular cell adhesion molecule 1 (VCAM1), used by CTCs to adhere to the inner vessel layer, enabling tumor cell extravasation [11]. Extracellular vesicles (EVs) are also critical mediators of these complex bi-directional tumor-host cell interactions which are now being deciphered to understand this metastatic organotropism [12].
Among other factors produced by the primary tumor that precondition the liver microenvironment to form niches of activated resident cells promoting tumor expansion, bone marrow-derived immune cells recruited to the liver, including neutrophils, fibrocytes, and myeloid-derived suppressor cells (MDSCs) also appear to play an important role [13]. Studying the diverse signaling mechanisms involved in this “pre-metastatic niche”, released by the primary tumor to the liver, identified TGF-β, interleukin 6 (IL-6), integrin beta-like 1 (ITGBL1), glucose-regulated protein 78 (GRP78), the tissue inhibitor of metalloproteinases (TIMP-1), and a significant list of miRNA signatures transferred via exosomes [14]. TGF-β appears to play a central role in the formation of this pre-metastatic niche, inducing migration of tumor cells toward the liver, eliciting fibrosis, and stimulating epithelial-to-mesenchymal transition (EMT) in hepatic and stellate cells, while dampening immune responses via repression of CD8+ T cells and promoting regulatory T cells (Tregs) and macrophages [6]. The creation of this fibrotic and immune suppressive environment is favored by the transition from a quiescent to an active state in HSCs in the space of Disse, where there is an interplay between T cells and MDSCs [15]. Pro-inflammatory signaling can also contribute to a pro-metastatic environment via secretion of serum amyloid A proteins (SAA) by hepatocytes [16], supporting metastatic seeding and colonization, both at the microvascular and extracellular phases of liver metastasis [17]. SAA appeared to represent a downstream mediator of IL-6 signaling that drove myeloid cell accumulation and fibrosis in the liver [18]. Surgical resection of the primary tumor also frequently results in ischemic injury to hepatocytes, the associated hyperactivation of inflammatory pathways promoting the recurrence of liver metastasis [19]. Finally, hepatic progenitor cells (HPCs) were found to be activated at the periphery and in the interior of liver metastases of colorectal carcinoma, in the form of immature ductular structures, whose role remains largely unknown [20].
Besides cell changes, modifications in the composition of the liver extracellular matrix (ECM) promote cancer cell adhesion and growth. To test whether liver ECM could determine colon CTCs propensity to metastasize to this organ, pioneering studies by Zvibel et al. [21] revealed a specific stimulatory effect of hepatocyte-derived ECM on colon cancer cells, followed by an induction of erb-b2 receptor tyrosine kinase 2 (ERBB2) expression. Fifteen years later, a transcriptional profiling of genes altered by this hepatocyte-derived ECM showed it increased the expression of heparin binding EGF like growth factor (HBEGF) and the colon stem cell marker leucine rich repeat containing G protein-coupled receptor 5 (LGR5 gene) [22]. Moreover, the early phase metastasis of human colon cancer cells in the liver involved another kind of interaction, through desmosomal junctions with hepatocytes [23]. Additional interactions of interest with hepatocytes included integrins, osteopontin, and claudins [7]. Another component of ECM remodeling is represented by lysyl oxidase (LOX), which mediates the cross-linking of collagen and elastin. LOX was found to be involved in the generation of a fibrotic microenvironment supporting metastatic growth [24], and its increased expression was correlated with hepatic metastasis [25]. Increased production of fibronectin, some collagen isoforms, and cell-adhesion molecules also contribute to pre-metastatic niche formation [26]. Adhesion of cancer cells is also promoted by binding α2β1 integrin to type IV collagen and some cadherins containing arginyl-glycyl-aspartic acid (RGD) motifs. This appeared to be associated with aggressive forms of cancer [27]. Finally, in the last decade, much insight has been gained regarding the liver metastatic process. However, important questions remain, such as the parameters governing the dormancy and activation of quiescent disseminated tumor cells [28]. Moreover, there are additional questions regarding the signals that activate them to enable the growth of macro-metastases through the various patterns of the macro-metastasis/organ parenchyma interface (MMPI) [29].
Colorectal liver metastasis
Among the various initial cancer localizations, colorectal cancer initially attracted particular scientific interest [30], as it is the 3rd most common cancer in developed countries and the 2nd leading cause of cancer-related mortality. It is currently estimated that 50–60% of patients diagnosed with this cancer develop liver metastases during their disease course [31]. A decade ago, the need to improve the understanding of the molecular mechanisms involved in the metastasis of colorectal cancer to the liver was emphasized, identifying insulin-like growth factor 1 (IGF-1), hypoxia-inducible factor 1-alpha (HIF1α), vascular endothelial growth factor (VEGF), epidermal growth factor receptor (EGFR), and integrin subunit beta 1 (ITGB1) as interesting potential biomarkers in this process for improving diagnosis for patients [32]. Meanwhile, both cellular (bone marrow-derived cells, HSCs, KCs) and molecular events (ECM remodeling) were investigated [33]. Among the first biomarkers identified, the upregulation of VEGF appeared to promote liver metastasis through a positive feedback loop with H2S anabolism and cystathionine-β-synthase [34]. HSC activation was found to involve 1) EVs secreted from highly metastatic colorectal cancer cells enriched in miR-181a-5p, 2) liver ECM remodeling [increased expression of alpha-smooth muscle actin (α-SMA) and fibronectin, decreased expression of vitronectin and tenascin C], and 3) secretion of C-C motif chemokine ligand 20 (CCL20), CCL24, and C-X-C motif chemokine ligand 10 (CXCL10) chemokines by activated HSCs [35]. In exosomes derived from the primary colorectal tumor tissue, the expression of angiopoietin-like protein 1 (ANGPTL1), a protein that regulates KC secretion patterns and especially decreases MMP-9 production, was also found to be downregulated [36]. Within proteins involved in ECM remodeling (see also the previous section for a general view of the liver metastatic process), the high level of TIMP-1 at the tumor front was found to be mediated by the release of EVs enriched in this protein by cancer cells, with a cytokine-like function in recipient fibroblasts, inducing ECM remodeling [37]. Besides collagens, which are the most abundant ECM components, the colorectal liver metastatic process involves changes in discoidin domain receptors (DDRs), which are part of the receptor tyrosine kinase family (RTK), cross-talking with several transmembrane receptors and thus playing the role of ECM sensors [38].
Given the very high incidence of colorectal cancer, and the fact that liver metastasis is the primary contributor to the death of patients with this cancer, therapeutic strategies have been directed towards therapeutic targeting of the tumor microenvironment through classification into four main molecular subtypes [39]. A recent review of the genomic profiling of liver metastases versus the primary colorectal tumors pointed to their considerable genetic heterogeneity with the co-existence of different intra-tumor sub-clones. The chromosome regions found to be preferentially altered in the liver metastases encoded genes encoding proteins degrading ECM (MMP-2), involved in cell adhesion [carcinoembryonic antigen-related cell adhesion molecule 7 (CEACAM7)], angiogenesis [angiopoietin 2 (ANGPT2)], cell growth (ERBB2) or cell dissemination [inhibitor of DNA binding 1 (ID1) and BCL2 like 1 (BCL2L1)], while an upregulation of genes from the TGF-β signaling pathway was also observed [40]. Another important discovery was the loss of the colon-specific gene transcription program found in liver metastatic colorectal cancer cells, substituted by a liver-specific one, driven by a reshaped epigenetic landscape where the transcription factors forkhead box A2 (FOXA2) and HNF1 homeobox A (HNF1A) appeared to play an important role [41]. Using a liver-specific transgenic model, Zeng et al. [42] also demonstrated the role of the overexpression of hepatocyte-intrinsic cell cycle-related kinase (CCRK) in liver immune microenvironment reprogramming to promote liver metastasis.
Reprogramming also concerns metabolic aspects as metastatic colon cancer cells are influenced by the specificities of the milieu they colonize. For example, the upregulation of aldolase B, involved in fructose metabolism, does not only represent a carbon source for stimulating tumor cell proliferation; but also a way to activate other pro-growth metabolic and signaling pathways [43]. As the liver is an organ rich in citrate, it is a perfect niche for attracting metastasizing cancer cells, helping them to survive [44]. Another driver of metastatic liver colonization associated with metabolic reprogramming is the increased expression of the red blood cell pyruvate kinase (PKLR), which negatively regulates the glycolytic activity of the major pyruvate kinase isoenzyme PKM2, increasing cellular glutathione levels [45]. Interestingly, expression of mitochondrial pyruvate carrier 1 (MPC1), the protein that transports pyruvate into the mitochondria and links glycolysis and the tricarboxylic (TCA) cycle, is also gradually decreased in liver metastasis relative to the primary tumor and normal tissue [46]. Additionally, several pathways of lipid metabolism are also concerned, such as fatty acids, the downregulation of one member of the protein tyrosine phosphatase family (PTPs), the receptor type O (PTPRO), promoting lipid accumulation and liver metastasis while decreasing overall survival [47]. The mevalonate pathway is also involved, its upregulation being documented in invasive cancers [48]. Finally, lipid metabolism reprogramming was also reported in cancer-associated fibroblasts, promoting liver metastasis through the secretion of CXCL5, TGF-β, MMP-2, and serpin family E member 1 (SERPINE1) [49].
All the factors described above contribute to producing a stromal-enriched microenvironment, initially characterized by leucocyte recruitment where L-selectins play an important role in association with the P-selectins present in platelets and endothelial cells, through the mediation of colorectal cancer cell extravasation [50]. A single-cell analysis of the spatiotemporal immune landscape of 97 samples of colorectal liver metastases revealed that among immunosuppressive immune cells, a population of highly metabolic M2 macrophages was present, characterized by their high expression of mannose receptor C-type 1 (MRC1) and CCL18, sharing the KC signature, suggesting their potential origin [51]. Besides this enrichment in immune suppressive cells, evidence of stem cell characteristics was reported one decade ago in 80% of clinical liver metastases, such as increased expression of the stem cell transcription factor gene Nanog homeobox (NANOG) [52]. Moreover, key processes in cancer stem cell metabolism during liver metastasis were recently reviewed, emphasizing the role played by the Warburg effect/glycolysis and fatty acid oxidation in increasing the production of ATP for energy production [53].
Other metastatic tumors
Many different types of cancer show a remarkable preference for the liver for establishing secondary tumors, including pancreatic cancer, which is characterized by a specific chemokine-chemokine receptor pattern where the early expression of C-X3-C motif chemokine receptor 1 (CX3CR1) appears to play a significant role [54]. Moreover, proteomic studies revealed the influence of exosomes released by pancreatic ductal adenocarcinoma cells taken by KCs, then reacting by increased TGF-β signaling, and releasing macrophage migratory inhibitory factor initiating the liver fibrosis pathway [55]. In ovarian cancer, the associated inflammation and subsequent destruction of the protective hyaluronan coat of the peritoneal mesothelium that follows co-optation of epithelial ovarian carcinoma cells with mesothelial cells, was found to involve the internalization of exosomes enriched in CD44, resulting in MMP-9 secretion, ultimately helping cancer cell invasion [56]. Neuroendocrine tumors [57] and prostate cancer, when found in the liver compared to other metastatic sites, present the worst prognosis, a specificity apparently related to phenotypic plasticity. E-cadherin downregulation (EMT) in prostate cancer cells allowed them to escape from the primary tumor mass, while hepatocytes were found to educate tumor cells to re-express E-cadherin to help tumor cells seed in and colonize the liver [58]. The same process, EMT, was mentioned in liver colonization by acute myeloid leukemia cells, a rarer event [59].
Insights from studies on peritoneal malignant mesothelioma
Investigations conducted on experimental malignant peritoneal mesothelioma (MPM) have provided insight into the molecular determinants of liver metastatic colonization. Proteomic analyses of the liver in an aggressive model of sarcomatoid MPM (M5-T1), established in the immunocompetent rat strain F344, resulted in identifying a set of proteins showing significant changes between liver tissues from three groups of rats. Cross-comparison of the proteomes from 1-normal rats (G1), 2-adjacent non-tumorous liver from untreated tumor-bearing rats (G2), and 3-curcumin-treated rats without hepatic metastases (G3), led to a list of 179 candidate biomarkers [60], of which 22 corresponded to proteins exclusively located in mitochondria, as shown in Table 1 below. This listing also includes 3 more proteins of interest, additionally located in the endoplasmic reticulum (encoded by Mgst1), cytosol (encoded by Decr1), and vesicles (encoded by Prdx3) (Table 1). For discussion on the 154 other non-mitochondrial proteins exhibiting changes in link with invasiveness, the reader is referred to previous work [60].
List of potential mitochondrial biomarkers involved in liver metastases by MPM invasive cells
Gene | Full name | Change # |
---|---|---|
Slc25a5 | ADP/ATP translocase 2 | ↓ |
Prdx5 | Peroxiredoxin-5, mitochondrial | ↓ |
Acadm | Medium-chain specific acyl-CoA dehydrogenase, mitochondrial | ↓ |
Acads | Short-chain specific acyl-CoA dehydrogenase, mitochondrial | ↓ |
Hmgcs2 | Hydroxymethylglutaryl-CoA synthase, mitochondrial | ↓ |
Idh3a | Isocitrate dehydrogenase [NAD] subunit alpha, mitochondrial | ↓ |
Dlat | Dihydrolipoyllysine-residue acetyltransferase component of pyruvate dehydrogenase complex, mitochondrial | ↓ |
Cpox | Oxygen-dependent coproporphyrinogen-III oxidase, mitochondrial | ↓ |
Ndufa10 | NADH dehydrogenase [ubiquinone] 1 alpha subcomplex subunit 10, mitochondrial | ↓ |
Aldh5a1 | Succinate-semialdehyde dehydrogenase, mitochondrial | ↓ |
Fis1 | Mitochondrial fission 1 protein | ↓ |
Sardh | Sarcosine dehydrogenase, mitochondrial | ↓ |
Nln | Neurolysin, mitochondrial | ↓ |
Acadl | Long-chain specific acyl-CoA dehydrogenase, mitochondrial | ↑ |
Aco2 | Aconitate hydratase, mitochondrial | ↑ |
Hadha | Trifunctional enzyme subunit alpha, mitochondrial | ↑ |
Grpel1 | GrpE protein homolog 1, mitochondrial | ↑ |
Micu1 | Calcium uptake protein 1, mitochondrial | ↑ |
Uqcrq | Cytochrome b-c1 complex subunit 8 | ↑ |
Vdac2 | Voltage-dependent anion-selective channel protein 2 | ↑ |
Ogdh | 2-oxoglutarate dehydrogenase, mitochondrial | ↑ |
Phb | Prohibitin | ↑ |
Mgst1* | Microsomal glutathione S-transferase 1 | ↑ |
Decr1* | 2,4-dienoyl-CoA reductase 1 | ↓ |
Prdx3* | Thioredoxin-dependent peroxide reductase, mitochondrial | ↓ |
* Proteins not strictly located in the mitochondria (according to https://www.proteinatlas.org/). Gene names are given for Rattus norvegicus (according to https://www.uniprot.org/). #: significant changes (P < 0.05) in G2 vs. G1, and G3 vs. G2. ↑: increase; ↓: decrease
Note. Adapted from “Curcumin treatment identifies therapeutic targets within biomarkers of liver colonization by highly invasive mesothelioma cells—potential links with sarcomas,” by Pouliquen DL, Boissard A, Henry C, Blandin S, Richomme P, Coqueret O, et al. Cancers (Basel). 2020;12:3384 (https://www.mdpi.com/2072-6694/12/11/3384). CC BY.
The 25 mitochondrial proteins listed above are involved in 10 main biological processes, the most important being lipid metabolism, heme biosynthesis, ion transport, the respiratory chain, protection against oxidative stress, mitochondrial dynamics, and hydrolytic processing of bioactive peptides in the extracellular environment (Figure 1). Five of these proteins were additionally reported as presenting some immune cell specificity for plasmacytoid dendritic cells (IDH3A), T cells (SARDH), and monocytes (NLN, PRDX3, MGST1). Interestingly, five proteins were also potential biomarkers in liver cancer, with their quantitative changes observed in [60] and Table 1 consistent with a favorable prognostic value established for three of them (encoded by Acads, Hmgcs2, and Aldh5a1), and a negative prognostic value was reported for the other two (encoded by Vdac2 and Phb).
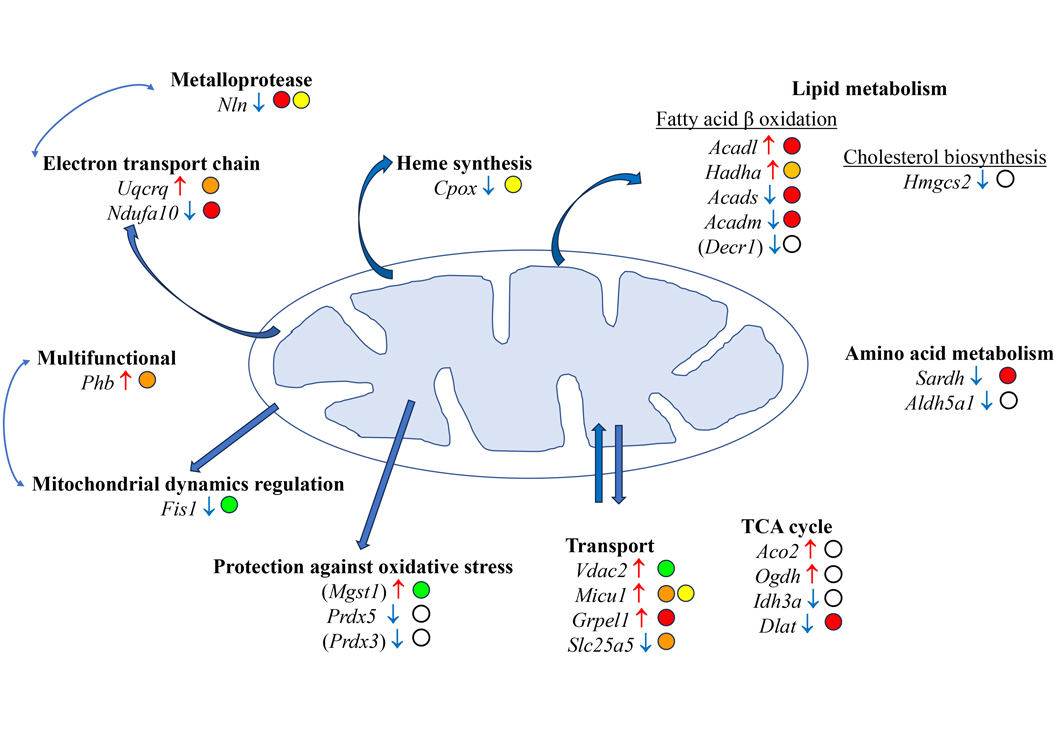
Main mitochondrial proteins potentially involved in the molecular determinants of liver metastatic colonization by aggressive MPM cells. Gene names are given for Rattus norvegicus (according to https://www.uniprot.org/). Gene names in brackets correspond to proteins not strictly located in the mitochondria (according to https://www.proteinatlas.org/). Open circles indicate submitochondrial locations. White circle: mitochondrion; green circle: outer membrane; yellow circle: inter membrane space; orange circle: inner membrane; red circle: matrix; ↑: increase; ↓: decrease
Mitochondrial biomarkers
Lipid metabolism
Metabolic reprogramming, including altered lipid metabolism, has been extensively documented in malignant tumors, and an update was recently provided by Raggi et al. [61] in the case of cholangiocarcinoma. In contrast, the literature has been scarce regarding the impact on liver lipid metabolism of malignant tumor progression which does not primarily develop in this organ. Linked to previous findings on MPM [60] and Table 1 above, an interesting observation came from the study by Ma et al. [62] who highlighted for the first time the role of ACADM in hepatocellular carcinoma (HCC). Regarding its hypothesized tumor suppressor role and downregulation in HCC, they showed that caveolin-1 enhanced the nuclear accumulation of the sterol regulatory element-binding protein-1 that was a direct upstream regulator of ACADM. Finally, they revealed the crucial role played by ACADM suppression in HCC aggressiveness [62]. Besides cancer, the expression of ACADM, which is increased in nonalcoholic fatty liver disease (NFLD), decreases with the stage and grade of the disease [63]. Conversely, the upregulation of this enzyme, produced by the consumption of three berry-based beverages for 18 weeks in obese rats, prevented hepatic steatosis [64]. Concerning the short-chain acyl-CoA dehydrogenase, ACADS, Chen et al. [65] reported that its downregulation is also related to hepatocarcinogenesis, thus attesting to its role as a biomarker for good liver function. In parallel, the increase previously observed in the long-chain acyl-CoA dehydrogenase, ACADL [60], a characteristic which was shared by both rat and human invasive mesothelioma cell lines [66], found an interesting echo in the work of Cai et al. [67] who recently reported its mechanoresponsive character in response to ECM stiffness in the context of lipid metabolic reprogramming in HCC. In the work of Di Carlo et al. [68], a lipidomic investigation showed that upregulation of another enzyme of fatty acid metabolism, encoded by the Hadha gene, was also concerned, linked to the study of pancreatic cancer stem cells. Conversely, the downregulation of HMGCS2 in this study [60] is consistent with previous observations made on this enzyme showing that decreased expression of the Hmgcs2 gene promoted NFLD [69], worsening hepatic lipid accumulation and liver injury [70]. Finally, DECR is an auxiliary fatty acid oxidation enzyme required for the full degradation of polyunsaturated fatty acyl-CoAs, deficiency of which in mice was shown to lead to hypoglycemia and cold intolerance [71]. DECR controls the balance between saturated and unsaturated phospholipids, and its deletion in mice was shown to impair lipid metabolism, leading to accumulation of unsaturated fatty acids in the form of triacylglycerols in the liver [72].
Heme biosynthesis and electron transport chain
Among the 25 mitochondrial proteins listed above, HEM6 (encoded by the Cpox gene), plays an important role not only in heme synthesis and erythropoiesis, but also in the production of mitochondrial electron transport chain [73]. In our previous study ([60] and Table 1), the downregulation of this enzyme suggests the liver’s mitochondrial energetics is affected during the early stages of liver metastatic colonization by invasive cancer cells. NDUAA (encoded by the Ndufa10 gene) is a subunit of the complex I of the mitochondrial respiratory chain. Its downregulation leads to abnormal mitochondrial ATP synthesis and reduced organ energy supply [74]. Interestingly, a study of the interactions between the mitochondrial import inner membrane translocase subunit (TIM22) and NDUFA10, conducted in liver tissues from patients with non-alcoholic steatohepatitis, revealed the involvement of this protein in mitochondrial fatty acid metabolism [75]. QCR8 (encoded by Uqcrq) is a small core-associated protein and a subunit of ubiquinol-cytochrome c reductase complex III associated with the oxidative phosphorylation pathway. Intriguingly, Zeng et al. [76] found a potential relationship with the pathogenesis of non-alcoholic steatohepatitis, although the mechanistic process remains obscure. Tian et al. [77] nevertheless revealed this protein is strongly associated with hypoxia stress resistance, a point of great interest in the context of the liver metabolic changes associated with metastatic liver colonization.
A peptidase, NLN, which is abundant in the liver [78], exhibited a decrease in the context of liver metastatic colonization by MPM cells. Although its mitochondrial function remains unknown, Mirali et al. [79] reported that NLN is required for the formation of respiratory quaternary superstructures involving complexes I, III, and IV. Their findings that NLN knockout mice demonstrated liver gluconeogenesis are also intriguing regarding the catabolism of amino acids and the link with the TCA cycle [80].
Transport
Within this category, one main protein for which a very significant decrease in its abundance was observed was the mitochondrial ADP/ATP carrier. This protein, involved in importing ADP into the mitochondrial matrix for ATP synthesis and the subsequent export of ATP, is a master regulator of mitochondrial energy. It also plays a crucial role in maintaining the mitochondrial membrane potential, ∆Ψm, and thus a decrease in its abundance means apoptosis is no longer prevented [81]. The expression of programmed cell death-related biomarkers, after SLC25A5 upregulation, was also documented [82]. In the context of the lipid metabolism alterations described above, their relationships with the voltage-dependent anion channel proteins (VDACs) have also been discussed, as these are the main cholesterol-binding proteins from the intermembrane contact sites of liver mitochondria [83]. The more specific role played by VDAC2 in the mitochondrial outer membrane, linked to its major differences in primary protein structures compared with VDAC1, has been emphasized, pointing to its indispensable role in the regulation of cholesterol transport [84]. An intriguing feature was also the parallel increase observed in VDAC2 and MICU1 [60], the latter being a regulator of mitochondrial Ca2+ uniporter, while the former was reported as mediating sarcoplasmic reticulum to mitochondria Ca2+ local transport [85]. Finally, although the interaction of VDAC2 with Bak has been well documented, its apparently pro-apoptotic function appears much more complex than expected [85, 86], with VDAC2 necessary for efficient Bax-mediated apoptosis, while conversely it inhibits Bak-mediated apoptosis [87].
The chaperone encoded by the Grpel1 gene is a protein which may belong to this category, as it is an essential component of the PAM complex, involved in the translocation of peptide-containing proteins that transit from the inner membrane into the matrix. Interestingly, Neupane et al. [88] found that deleting this protein was accompanied by a shut-down of oxidative phosphorylation and mitochondrial fatty acid oxidation. Thus, its increase observed in the context of liver colonization by MPM invasive cells [60] is consistent with the parallel upregulation of several enzymes in fatty acid beta oxidation.
Integrated stress response and mitochondrial dynamics
Another interesting observation concerns FIS1, a protein involved in the fragmentation of the mitochondrial network and its perinuclear clustering. The complex role of FIS1 in additional functions, including mitophagy, apoptosis, and mitochondria-associated membrane events, was also reviewed [89]. In studies on MPM [60], the decreased abundance of this protein is consistent with previously reported cell senescence, accumulation of damaged mitochondria, and higher reactive oxygen species (ROS) production [90]. As emphasized by Liou et al. [91], in link with mitophagy, FIS1 is also involved in the integrated stress response, both representing processes preserving mitochondrial functions against oxidative stress. Vidyadharan et al. [92] also reported that the hepatic mitochondrial dysfunction induced by a low protein diet in rats is also associated with the downregulation of Fis1. Interestingly, the balance between mitochondrial fusion and fission also involves PHB [92], which, conversely to FIS1, was found elevated in the context of liver metastatic colonization by MPM cells [60]. Yanran et al. [93] observed that PHB (PHB1) deficiency led to altered lipid metabolism, resulting in an increase in intracellular lipid accumulation.
Liver metabolism, energy production, and TCA cycle
Changes occurring in the metabolism and/or plasticity of the mitochondria of a tumor-influenced liver have previously been documented for aggressive tumors, pointing to the shift in liver metabolism in the host toward energy utilization rather than energy storage [94]. Both abnormal mitochondrial biogenesis and dynamic parameters of mitochondrial water phases appeared to accompany this event [95], for example, the presence of an invasive malignant brain tumor in rats induced a loss of water structural order following the changes observed in the physical properties of the hydration shells of liver mitochondria macromolecules [96]. In this field, the impact of the growth of experimental tumors is characterized by alterations in host carbohydrate metabolism, especially a decrease in glucose oxidation by hepatocytes [94]. The observed downregulation of glycolytic enzymes in the liver of MPM-bearing rats, such as triosephosphate isomerase (TPIS) and glucose-6-phosphate isomerase (G6PI), was consistent with a parallel decrease in IDH3A [60].
An intriguing feature is represented by the elevation in 2-oxoglutarate dehydrogenase (ODO1, encoded by Ogdh), an enzyme not strictly located in the mitochondria and that was reported as serving as a redox sensor in addition to its role in the production of mitochondrial NADH [97]. Given that a fraction of this enzyme, which localizes in the nucleus, is required for lysine succinylation of histones, its upregulation in MPM-bearing rats may be related to its role in this post-translational process recognized as promoting tumor growth [98]. However, the main role of this enzyme is in the TCA cycle, at the crossroads of various metabolic pathways for the oxidation of fuel molecules, including carbohydrates, fatty acids, and amino acids. The parallel decrease in ODP2 (encoded by Dlat), which links the glycolytic pathway to the TCA cycle is also consistent with the decrease in fatty acid synthesis and increase in ODO1. Finally, as the oxidation of acetyl-CoA to CO2 by the TCA cycle is the central process in energy metabolism, anaplerosis may also play a crucial role in the context of a tumor-influenced liver [99, 100]. The increase in aconitate hydratase (ACON, encoded by Aco2) is in line with the upregulation of this enzyme [60] and the role of citrate in the promotion of tumor growth, as emphasized by Desideri et al. [101]. You et al. [102] have emphasized its role as a bridge between the TCA cycle and lipid metabolism, with its downregulation, leading to enhanced fatty acid synthesis, while an opposite situation was found in previous study [60]. The same decrease in Aco2 expression, leading to fatty acids biosynthesis and lipid accumulation, was also observed in the context of mitochondrial stress in Caenorhabditis elegans [103].
An additional feature concerns SSDH (encoded by Aldh5a1), an enzyme involved in the catabolism of γ-aminobutyrate (GABA), which decreased in the context of previous studies [60]. Interestingly, Ravasz et al. [104] established a link between molecules catabolized through the GABA shunt towards the TCA cycle, and impairment of mitochondrial substrate-level phosphorylation. This observation can also be viewed in the light of the parallel upregulation of Ogdh (discussed above), as both enzymes lead to the production of succinic semialdehyde in the matrix.
Protection against oxidative stress
The association between ROS and cancer progression has been well documented through the role of members of the peroxiredoxin family. Among them, two proteins correspond to mitochondrial ROS scavengers, PRDX3 and PRDX5 [105].
Early studies on differential expression of these enzymes in cancer cells have revealed a regulatory role for elevated PRDX3 levels on oxidation-induced apoptosis in hepatocellular carcinoma [106]. Moreover, serum PRDX3 was found to be significantly higher in patients with hepatocellular carcinoma, compared with liver cirrhosis and healthy controls, suggesting it could be a noninvasive biomarker for diagnosis and/or prognosis [107]. Apart from liver cancer, and in agreement with previous findings [60], chemical-induced acute liver injury produced in mice led to downregulation of Prdx3 [108]. In line with these findings, Geng et al. [109] reported that the decrease in Prdx3 expression observed in nonalcoholic liver disease was inhibited by treatment with a natural diterpenoid, carnosol, with this hepatoprotection being largely abolished by specific PRDX3 siRNA. Finally, Sun et al. [110] revealed that PRDX3 silencing exacerbated hepatic fibrogenesis and HSC activation, confirming that this enzyme acts as a master regulator of mitochondrial oxidative stress.
In contrast with PRDX3, PRDX5 exhibits a remarkably wide subcellular localization, located in the mitochondria when expressed as a long form (with its mitochondrial targeting sequence), while in the cytosol, peroxisomes, and nucleus in its short form [111]. Overexpression of PRDX5 is common in many different types of cancer, and it can additionally be a negative survival predictor in patients [112]. Its protective role was established early in a rat model of liver transplantation, its overexpression attenuating the severe oxidative stress status, leading to an increase in the recipient’s survival [113]. In mice with obesity induced by a high-fat diet, Kim et al. [114] demonstrated that PRDX5 deletion also produced lipid metabolic disorders leading to hepatic steatosis, suggesting a role in adipogenic gene expression. The same team further showed that Prdx5 overexpression suppressed cytosolic and mitochondrial ROS generation in an experimental model of NFLD by regulating the AMP-activated protein kinase pathway [115]. Thus, the decrease in both PRDX3 and PRDX5 abundance observed in the liver of MPM-influenced rats [60] was consistent with an exacerbation of mitochondrial stress.
Conclusions
Investigations into the molecular determinants of liver metastatic colonization are a growing field of research. This review first provides an update to the main features relevant to this exciting topic. Recent breakthroughs deciphered the role of key molecules involved in intercellular communication between CTCs and the heterogeneous population of liver cells, including HSCs, KCs, and LSECs. The role of exosomes derived from the primary tumor, ECM, and liver metabolic reprogramming was also emphasized. Genomic profiling of liver metastases vs. primary tumors, and single cell analyses of their immune landscape also completed these efforts. Secondly, based on previous cross-comparison of liver proteome changes between MPM-bearing rats and normal rats, the role of 25 mitochondrial proteins in tumor invasiveness, identified from a list of 179 candidate biomarkers, was discussed in link with the published literature. Their involvement in lipid metabolism, heme biosynthesis, electron transport chain, energy production, protection against oxidative stress, and mitochondrial dynamics and transport was particularly emphasized, all representing growing fields of interest. This tool is likely to be a good basis for the future design of innovative therapeutic strategies that could benefit patients facing the harmful consequences of liver invasion by aggressive cancers.
Abbreviations
ACADL: | long-chain specific acyl-CoA dehydrogenase, mitochondrial |
ACADM: | medium-chain specific acyl-CoA dehydrogenase, mitochondrial |
ACADS: | short-chain specific acyl-CoA dehydrogenase, mitochondrial |
ACO2: | aconitate hydratase, mitochondrial |
ALDH5A1: | succinate-semialdehyde dehydrogenase, mitochondrial |
CCL20: | C-C motif chemokine ligand 20 |
CPOX: | oxygen-dependent coproporphyrinogen-III oxidase, mitochondrial |
CTCs: | circulating tumor cells |
DECR1: | 2,4-dienoyl-CoA reductase 1 |
DLAT: | dihydrolipoyllysine-residue acetyltransferase component of pyruvate dehydrogenase complex, mitochondrial |
ECM: | extracellular matrix |
EMT: | epithelial-to-mesenchymal transition |
EVs: | extracellular vesicles |
FIS1: | mitochondrial fission 1 protein |
GRPEL1: | GrpE protein homolog 1, mitochondrial |
HADHA: | trifunctional enzyme subunit alpha, mitochondrial |
HCC: | hepatocellular carcinoma |
HMGCS2: | hydroxymethylglutaryl-CoA synthase, mitochondrial |
HSCs: | epatic stellate cells |
IDH3A: | isocitrate dehydrogenase [NAD] subunit alpha, mitochondrial |
ITGBL1: | integrin beta-like 1 |
KCs: | Kupffer cells |
MGST1: | microsomal glutathione S-transferase 1 |
MICU1: | calcium uptake protein 1, mitochondrial |
MMPs: | matrix metalloproteinases |
MPM: | malignant peritoneal mesothelioma |
NDUFA10: | NADH dehydrogenase [ubiquinone] 1 alpha subcomplex subunit 10, mitochondrial |
NFLD: | nonalcoholic fatty liver disease |
NLN: | neurolysin, mitochondrial |
OGDH: | 2-oxoglutarate dehydrogenase, mitochondrial |
PHB: | prohibitin |
PRDX3: | thioredoxin-dependent peroxide reductase, mitochondrial |
PRDX5: | peroxiredoxin-5, mitochondrial |
SARDH: | sarcosine dehydrogenase, mitochondrial |
SLC25A5: | ADP/ATP translocase 2 |
TCA: | tricarboxylic |
TGF-β: | transforming growth factor-beta |
UQCRQ: | cytochrome b-c1 complex subunit 8 |
VDAC2: | voltage-dependent anion-selective channel protein 2 |
Declarations
Author contributions
DLP: Writing—original draft, Writing—review & editing.
Conflicts of interest
The author declares that he has no conflicts of interest.
Ethical approval
Not applicable.
Consent to participate
Not applicable.
Consent to publish
Not applicable.
Availability of data and materials
Not applicable.
Funding
This review was prepared with the support of the University of Angers, including the Mir program “Mobilité Internationale pour la Recherche” between the Universities of Angers (France) and Turin (Italy) in 2020–2021 and 2021–2022. The funder (University of Angers) had no role in study design, data collection and analysis, decision to publish, or preparation of the manuscript.
Copyright
© The Author(s) 2024.