Abstract
Aim:
After the severe acute respiratory syndrome coronavirus 2 (SARS-CoV-2) pandemic and the realization of mass vaccination against the virus, the availability of a reliable, rapid, and easy-to-use system for registering the individual anti-S1 antibody titer could facilitate the personalized assessment of the need for booster vaccine doses and the reduction of social distancing and other measures.
Methods:
The biosensor system is based on immobilized engineered SK-N-SH neuroblastoma cells, bearing the S1 protein, and it can detect immunoglobulin G (IgG) antibodies against the SARS-CoV-2 S1 spike antigen. A disposable electrode strip bearing the engineered mammalian cells is connected to a customized read-out potentiometric device with real-time data transmission to a wireless fidelity (WiFi)-connected smartphone. Blood samples from past-infected individuals and individuals vaccinated against SARS-CoV-2 were used for validation.
Results:
In the present study, a smartphone application (app), capable of analyzing data regarding the levels of anti-S1 antibodies in blood is introduced. The app works in conjunction with a portable, ultra-rapid, and sensitive biosensor transmitting real-time measurements to the smartphone. Both historical and current individual data can be encoded by using the app, resulting in a widely accepted quick response (QR) code, which can then be constantly updated to match a person’s status.
Conclusions:
This novel system could be utilized for the eventual development of a coronavirus disease 2019 (COVID-19) electronic passport, which could be further employed to improve the population-wide, cross-country surveillance of vaccination efficiency, as well as facilitate the implementation of cross-border digital health services in a user-friendly and secure way.
Keywords
Antibody screening, biosensor, health passport, quick response code, severe acute respiratory syndrome coronavirus 2, smartphone, surveillance, vaccinationIntroduction
A coronavirus disease 2019 (COVID-19) electronic passport (or “health passport”) is a digital document that confirms an individual’s COVID-19 status, such as whether they have been vaccinated or have recovered from the virus. The passport can be stored on a smartphone or other digital device and can be used by employers, governments, or other organizations to manage the spread of the virus by restricting access to high-risk individuals. From an ethical standpoint, it has been debated both in terms of privacy and legal concerns [1, 2], and individual discrimination [3], and it has been proven to be a tool of vital importance in keeping the economy alive and society more secure [4]. A major consideration when designing such systems is data security, by storing COVID-19 status information electronically, individuals can have more control over who has access to their health information and can be more confident that their data is being securely managed. However, it is important to ensure that the development and implementation of these “health passports” are guided by robust privacy and security measures to ensure that they are used in a way that is fair and ethical [5].
Said systems rely on off-line reports of vaccination, which can be either outdated or more seriously tampered with. More advanced approaches can include data regarding screening for antibodies, which is an indicator of immunity [6]. Accumulation of the spike S1 glycoprotein antigen and its antibodies in blood plasma is rapidly affected after vaccination, therefore by quantifying this parameter, robust conclusions can be extracted regarding health and immunity against the virus [7]. A lot of high-end system designs have been presented, with tamper-proof, end-to-end secure systems for authentication of COVID certifications [8, 9]. The main flaw of these systems is that an error in the initial test will propagate all the way through the secure channel, which highlights another critical aspect: the time required for testing. Recently, an interesting research paper discussed the use of “antibodies as currency” [10]; it is mandatory for various critical sectors to be able to test for antibodies constantly, at rapid rates, with low-cost and high success, as well as integrating such testing system to their infrastructure.
Creating an application (app) with a quick response (QR) code to support measurement results is critical for several reasons, particularly in the context of a health passport. Firstly, a QR code is an efficient and convenient way to store and transmit large amounts of data in a compact and secure form [11, 12]. A single code can contain all the necessary information regarding an individual’s health, including their measurement results, allowing for easy and accurate tracking of their health status. Additionally, the use of a QR code helps to eliminate the possibility of manual errors that can occur when recording and transmitting data manually. This is particularly important in healthcare settings, where accuracy and efficiency are paramount. Various studies have been conducted that demonstrate the positive impact of this technology on all aspects of a country’s healthcare system [13, 14]. The COVID-19 pandemic has highlighted the importance of using technology to help mitigate the spread of the virus and support public health efforts. QR codes have emerged as a valuable tool in this regard, providing several benefits during the pandemic such as contact tracing, vaccine passports, telemedicine, etc. [15, 16]. Furthermore, QR code-based health information can be easily integrated into existing systems and technologies, making it accessible to a wide range of users, including those who may not have a strong technical background.
In this work, a smartphone app is presented, capable of analyzing data regarding anti-S1 antibodies which could be detected at concentrations as low as 5 ng/mL using a previously published novel biosensor by Mavrikou et al. [17]. The app is designed to encode both historical and current data, which are combined with the results from the customized embedded device, resulting in a widely accepted QR code. This QR code includes useful information and can be constantly updated to match a person’s status.
Materials and methods
Data collection
Original input data were derived with the aid of a previously reported bioelectric biosensor by Mavrikou et al. [17]. The biosensor, based on SK-N-SH cells engineered to bear the S1 protein on their surface, is able to detect immunoglobulin G (IgG) antibodies against the severe acute respiratory syndrome coronavirus 2 (SARS-CoV-2) S1 spike antigen in less than 3 min by measuring changes in the cell membrane potential following the binding of antibodies to the membrane-inserted antigens. The biosensor comprises a disposable sensor strip (iMiCROQ S.L., Tarragona, Spain) containing eight screen-printed carbon electrodes [working electrode: carbon, reference: silver/silver chloride (Ag/AgCl)] bearing the immobilized membrane-engineered cells and connected to a customized read-out potentiometric device (see Device-data preparation below). For the measurements, various blood samples from past-infected individuals (four volunteers) and individuals vaccinated against SARS-CoV-2 [three healthy volunteers immunized with the second dose of messenger RNA (mRNA) vaccines] were tested. The same samples were used in a previous study [17] for validating the biosensor system. Samples were collected in 5 mL volume in Becton Dickinson Vacutainer ethylenediaminetetraacetic acid (EDTA) tubes (18 mg, potassium EDTA, K2EDTA). Plasma samples were separated from the EDTA blood after centrifugation at 1,200 g for 15 min and stored at –80°C for further analysis. For validation purposes and prior to biosensor analysis, the antibody titer in the samples was determined with the Roche Elecsys® anti-SARS-CoV-2 and Elecsys® anti-SARS-CoV-2 S immunoassays run on the Roche Cobas e 411 analytical unit, following the manufacturer’s instructions [cut-off index (COI) ≥ 1.0 U/mL and > 0.8 U/mL, respectively, was considered positive].
Device-data preparation
The system block diagram is presented in Figure 1; a short analysis of the discrete blocks follows: the high-bit precision analog front-end is responsible for sensing the bioelectric potential changes from the cell membrane. A specific printed circuit board (PCB) layout has been implemented for the highly sensitive signals, as well as decoupling strategies for noise coming from the surrounding electronics and the environment. The measurements are periodically polled from an ultra-low-power 32-bit Advanced RISC Machine (ARM) Cortex-Microcontroller Family (M4), STMicroelectronics (STM32L476RG) via a serial peripheral interface (SPI) bus at a max speed of 20 MHz. A moving median filter with a Window of 250 measurements per channel is implemented in an isolated real-time operating system (RTOS, FreeRTOS) thread, while an algorithm for on-the-fly programmable gain amplifier (PGA) correction has been implemented for dealing with saturation, depending on the measured potential.
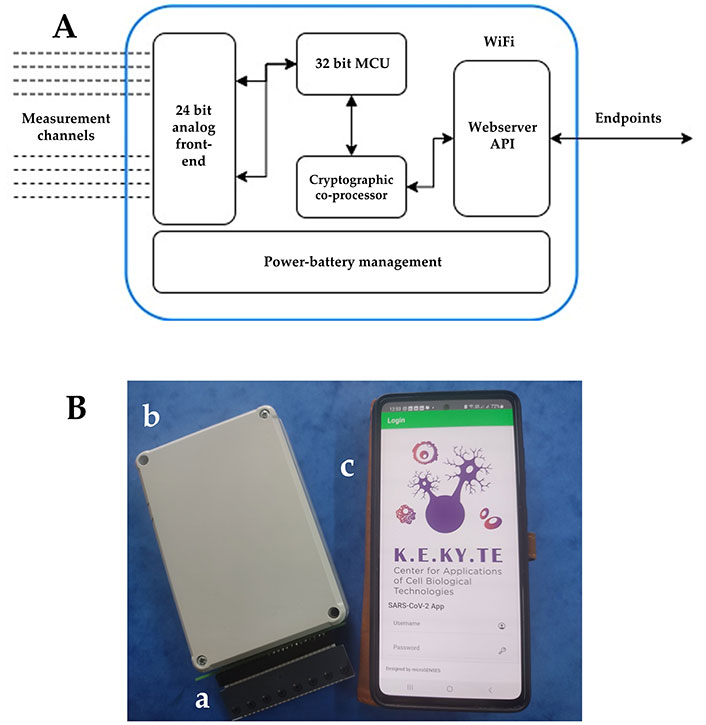
Integrated smartphone/biosensor system. (A) System block diagram; (B) integrated biosensor system with real-time data transmission to the wireless fidelity (WiFi)-connected smartphone: (a) disposable electrode strip with the biosensing consumable; (b) read-out potentiometer; (c) smartphone with the embedded app. MCU: microcontroller unit; API: app programming interface
Finally, the system is powered using a rechargeable Lithium Polymer (LiPo) battery with the appropriate charging and protection circuit on-board. By using a battery and linear regulation, all sources of noise coming from high-frequency switching regulation blocks have been avoided. Results from each sample were expressed as a ratio to control [mean ± standard deviation (SD)] according to the following equation:
Results (normalized biosensor responses) above the COI of 3 were considered positive, i.e. they represented an antibody titer higher than 0.8 U/mL.
All communication between the device and the smartphone is implemented via a custom API over hypertext transfer protocol secure (HTTPS), to ensure up-to-date data safety. Additional security measures taken are: a) the device and the smartphone operate over WiFi protected access 2 (WPA2)-Counter mode with Cipher block chaining Message authentication code Protocol (CCMP), with the capability for expanding to WPA3; b) the data is validated with hash-based message authentication code (HMAC)-secure hash algorithm 256 (SHA-256) using a custom scheme; c) additional measures regarding datapoint originality have been implemented (timestamp cross-checking, etc.).
In the context of detecting potential malware or unauthorized access on the local network, the implementation of an additional layer of authentication between the device and the smartphone is being considered. This additional security measure involves combining the registered username with a unique identifier, such as the media access control (MAC) address. Additionally, a warning could appear in case of the detection of multiple clients to the device’s webserver.
Smartphone app development
Choosing the right development environment and tools is a vital step in implementing an app [18, 19]. For this project, the Ionic Framework (V6.20.3) has been chosen, an open-source framework that facilitates the development of hybrid mobile apps. By utilizing hypertext markup language 5 (HTML5), the framework creates a native-like look and feel while providing developers with the flexibility to tailor the user interface (UI) to fit the conventions of the target platform, such as Android, iPhone operating system (iOS), or others [20]. The goal is to achieve optimal user experience and platform adaptability.
Results
The developed smartphone app is designed to be user-friendly with a minimalist approach. It consists of two functional screens: the login screen and the data input/measuring/QR code generation screen (as illustrated in Figure 2). Upon successful authentication, the app loads the user’s personalized settings, including their username and status. The second screen allows users to input their personal data, such as their name, surname, gender, age, date, and vaccination status. The app accommodates two vaccination status scenarios, allowing users to indicate whether they are fully vaccinated or not alongside all necessary information (number of doses, date of last dose, date of last SARS-CoV-2 infection). Once the user completes the data entry form, they can enable the measurement button, which triggers the app to communicate with the biosensor and retrieve the measurement results automatically. That means, that after the button is pressed, a full experiment is performed, and the results are transferred wirelessly to the smartphone for creating the QR code. This streamlined process eliminates the possibility of manual errors and saves valuable time for users. After the measurement data is entered, the app generates a unique QR code that encodes all the information, including the user’s personal details and the measurement data. This QR code serves as a digital record of the user’s health status and can be easily scanned by authorities or health professionals. The app provides a convenient and secure way for users to share their health status with others. Users can automatically transmit the personalized QR code picture along with their essential information via email or short message service (SMS).
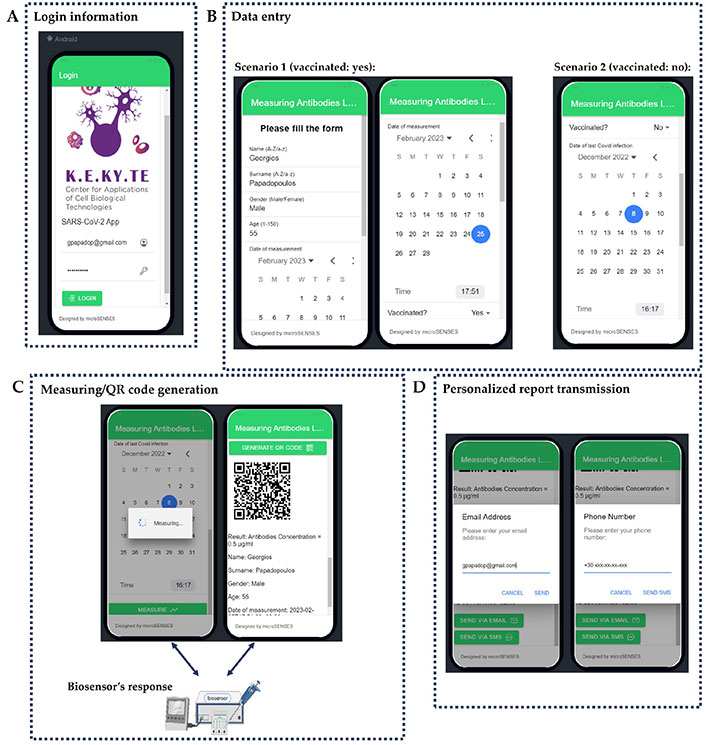
App prototype basic working screens. (A) Login information; (B) data entry; (C) measuring/QR code generation; (D) personalized report transmission
Additionally, it should be mentioned that the chosen method of development uses a hybrid mobile approach which allows the simultaneous development of both Android, iOS and Windows-based UIs versions as shown in Figure 3. In addition, the chosen development framework provides ample opportunities for customization, enabling developers to tailor user experiences to specific platforms. This ensures that the app is optimized for each platform’s conventions, resulting in a better overall user experience. The approach of developing an app simultaneously on multiple platforms with a shared codebase offers numerous advantages. It not only saves time and resources but also ensures a high-quality product that can be accessible to a wider range of users.
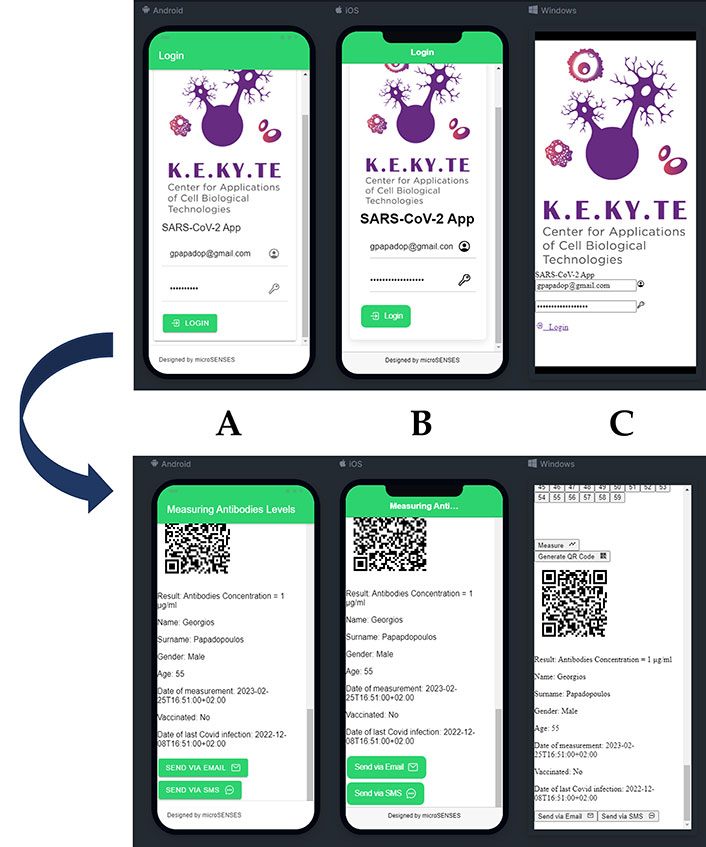
Multi-platform deployment. (A) Android; (B) iOS; (C) Windows-based UI. Subgraph A, B, and C corresponds to the upper and lower images respectively
Discussion
Beyond individual protection against COVID-19, one of the primary goals of global vaccination programs is the building up of population-level (“herd”) immunity [21]. Achieving an efficient degree of acquired immunity is a highly personalized process since it depends on various factors, including age, sex, underlying disease, and also previous infection [22]. It has been demonstrated that the titer of SARS-CoV-2 neutralizing antibodies decreases over time to non-detectable levels, in part depending on the aforementioned individual factors [23, 24]. This fact is reflected in the validity of current vaccination certificates: for example, European Union (EU) member states must accept vaccination certificates for a period of nine months after the administration of the last dose of the primary vaccination and then be subject to renewal [25]. Digital COVID certificates are being remarkably adopted as standard tools for cross-country epidemiological surveillance, along with the global spread of healthcare digitalization. For example, more than 2 billion EU digital COVID certificates have been issued, while 49 third countries and territories across five continents have joined the system, in addition to the 27 member states. The value of the vaccination certificates is indisputable since they allow for unrestricted movement across system-complied territory. Even though the possession of digital COVID certificates is currently considered only on a voluntary basis, they may become obligatory if the epidemiological situation worsens severely. Even under normal conditions, the certificate would serve as proof of vaccination or recovery, to facilitate access to healthcare units, workplaces, schools, gyms, recreational centers, hotels, and transportation means.
On the other hand, current digital COVID certificates only use the date of last vaccination as the time point of reference, thus entirely disregarding the individual profile of acquired immunity. Currently, digital certificates may be issued based on either molecular nucleic acid amplification tests (NAAT) including those using reverse transcription polymerase chain reaction (RT-PCR), or rapid antigen tests. For the later information to be embedded in a digital certificate, a frequent update on the individual anti-SARS-CoV-2 antibody titer would be required. This is feasible only by using low-cost, rapid, and user-friendly antibody tests which would allow for the regular, mass-screening determination of antibody concentrations in the wider population. In addition, these tests should be configured to allow the rapid and easy transmission of the test results to the digital certificate issuing system, especially during periods of high demand (e.g., holidays).
This is precisely the need covered by the app reported in this study, which is coupled to an antibody test based on a bioelectric biosensor able to deliver results in a fast and readily digitalized format, therefore allowing for an entirely streamlined process. The wide dissemination and eventual use of the app could lead to a drastically improved assessment of the level of vaccination efficiency in the general population and help design the recommendations of the booster dose to those individuals with a justified need, i.e. low immunity documented by low antibody titer, as unequivocally reported in their personal digital certificate. Such a development could contribute to a radical rationalization of global vaccination strategies, allowing for optimizing the distribution of resources to cope with emerging coronavirus variants.
The app developed in the present study could be possibly expanded to include certification of other vaccination targets as well as the authentication of documents in the health domain. It could also boost the successful implementation of cross-border digital health services in a user-friendly way, at the same time securing personal data, e.g., in the context of the development of the European Health Data Space. Finally, it could help health agencies to better adapt to seasonality SARS-CoV-2 infection patterns due to various factors, such as climate.
Abbreviations
app: | application |
COVID-19: | coronavirus disease 2019 |
EDTA: | ethylenediaminetetraacetic acid |
iOS: | iPhone operating system |
QR: | quick response |
SARS-CoV-2: | severe acute respiratory syndrome coronavirus 2 |
UI: | user interface |
WiFi: | wireless fidelity |
Declarations
Author contributions
AA: Conceptualization, Methodology, Software, Validation, Writing—original draft. DB: Conceptualization, Validation, Writing—original draft. SM, GMP, VT, KH, EK, and MT: Methodology, Investigation. AM, GPC, and CKG: Methodology, Investigation, Supervision. GK: Conceptualization, Supervision. SK: Conceptualization, Writing—original draft, Writing—review & editing, Supervision. All authors read and approved the submitted version.
Conflicts of interest
The authors declare that they have no conflicts of interest.
Ethical approval
The clinical study was approved by the Scientific Committee of the “Aghia Sophia” Children’s Hospital. The study was conducted according to the guidelines of the Declaration of Helsinki.
Consent to participate
Informed consent to participate in the study was obtained from all participants.
Consent to publication
Informed consent to publication was obtained from relevant participants.
Availability of data and materials
The data presented in this study are not publicly available due to medical confidentiality and privacy. However, the data are available on request from the corresponding author.
Funding
Not applicable.
Copyright
© The Author(s) 2024.