Abstract
Folic acid (FA) residue is a well-known and widely spread targeting ligand, or biovector, used as a component in engineering artificial nanosized and submicron particles (NSPs) to target various cells and treat a variety of diseases that are associated with FA (or folate) receptors (FR) overexpression. A particular place in the list of these diseases is held by various cancer types, for which overexpression of FR on the cell surface is often accompanied by a more severe disease course, increased resistance to the conventional chemotherapy, and poorer prognosis. The incorporation of albumin into NSPs has been shown to enhance their biocompatibility, give high compatibility with drug molecules, prolong their circulation, reduce thrombogenic activity, and improve their colloidal stability. Nowadays albumin-based NSPs with FA residue as a targeting agent are used for chemotherapy, photothermal and photodynamic therapy, combined therapy, visualization, and theranostics (also known as theragnostics) for various types of cancer: breast, cervical, ovarian, prostate, nasopharyngeal, gastric, colorectal, liver cancer, and brain tumors. A limited number of studies have also focused on the use of NSPs in rheumatoid arthritis treatment. In this review, we discuss the ways of FA-albumin conjugation and conjugation of FA to albumin-modified NSPs systems. An application of nanosized and submicron delivery systems on the base of serum albumin and FA for therapy and diagnostics is discussed.
Keywords
Human serum albumin (HSA), bovine serum albumin (BSA), folic acid, conjugation, folate-albumin conjugate, delivery systems, nano- and submicroparticles (NSPs), carbodiimide reactionIntroduction
Folic acid (FA) is a well-known molecule used for treatment and prevention of different diseases. FA (or folate) receptors (FR) are expressed in all cells of living organisms but overexpressed in some types of cells of malignances, particularly various types of cancer cells [1, 2], osteosarcoma [3], acute myeloid leukemia [4], as well as tumor-associated macrophages, which are often present in the microenvironment of both epithelial and non-epithelial tumors and affect tumor progression, immune evasion, and metastasis [5], and activated macrophages associated with autoimmune diseases such as rheumatoid arthritis [6]. For example, in epidermal carcinoma, FR overexpression can be approximately 100–300 times higher compared to normal cells [7]. There is a positive correlation between the level of FR expression in tumor cells and their resistance to standard chemotherapy. In most cases, FR overexpression on the surface of cancer cells is associated with a severe disease course, for instance, in the case of breast cancer, FR overexpression is linked with worse outcomes including decreased disease-free survival rates [8, 9]. In the case of primary and metastatic head and neck squamous cell carcinomas patients with FR overexpression have a lower survival rate [10].
Using targeting ligands, that generally can be called biovectors (molecules of natural or synthetic origin that are able to target cells) is a rather common approach in modern nanobiotechnology. The conventional approaches in cancer therapy such as chemotherapy and radiotherapy are known to lead to dramatic nontargeted effects on the human body including a decrease in the effective cytotoxic dose effect of therapeutic agents on cancerous cells and growth of inhibitory effect on normal cells [11, 12]. Hybridization of FA residue as a vector to other molecules and confirmation of its effectiveness as a targeting ligand comes back to the 1940s [13]. Then in 50 following years, there were numerous works devoted to FA conjugation to other molecules in PubMed database (only approximately 9% belongs to these 50 years compared of all amount of works devoted to this area of research from the 1940s to nowadays), while later the number of works grows dramatically, and their number in the recent 10 years have reached approximately 55% of the total number.
There are several FA-linked applications for targeted delivery of therapeutic agents that have been tested in the clinic so far. In some cases, the authors call the systems obtained for targeted delivery via FR “FA-targeted”. So, the applications include FA-targeted protein toxins (such as use of FA-Pseudomonas exotoxin conjugate to kill FR-positive cancer cells), FA-targeted chemotherapeutic agents (such as FA-paclitaxel and FA-camptothecin), FA-targeted immunotherapy (such as use of FA/anti-T cell receptor to train the immune system to recognize tumor activating mutations), FA-targeted gene therapy (such as FA-nonviral gene therapy vectors) [14, 15], folate-targeted antisense oligodeoxyribonucleotides (ODN) and small interfering RNAs (siRNA) delivery (such as use of ODN or siRNA to knockout or modulate gene expression) [16], radioimaging and radiotherapic agents [17, 18], and folate-targeted systems for magnetic resonance imaging (MRI) and other. Some of the therapeutic systems including FA residue have been tested in preclinical [19] and clinical studies up to now (for instance NCT04241315 completed in 2023).
Nanosized and submicron particles, or nano- and submicroparticles (NSPs), have been widely engineered for targeted cancer therapy and diagnostics in recent decades. The NSPs can be core-shall, dendrimeric, layer-by-layer structures, mono- and multicomponent particles, micelles, etc. [20–22]. Non-organic, organic, and hybrid particles with components of varied molecular weight have been evaluated. Among natural (albumin, gelatin, chitosan, dextran, hyaluronic acid, lactoferrin) and synthetic [polyethylene glycol, polyamidoamine, polylactic acid, polyethyleneimine, poly(L-lactide)-b-poly(ethylene glycol)] macromolecules, albumin as a component of NSPs possesses a great interest due to its biocompatibility, biodegradability, low immunogenicity, the long half-life, minimal toxicity, good stability, good reactivity, and high compatibility with drug molecules [23]. It has been shown also that incorporation of albumin in the structure of nanoparticles prolongs their circulation [24], reduces the thrombogenic activity [25], and improves their colloidal stability, etc. [26, 27]. Moreover, human serum albumin (HSA) is extremely robust towards pH (this protein is stable in the pH range of 4–10), and temperature (can be heated at 60°C for up to 10 hours) [28].
Albumin in composition of NSPs can also be considered a targeting agent due to the fact that Glycoproteins (Gp18, Gp30, and Gp60) receptor and albumin-binding glycoprotein [secreted protein acidic and rich in cysteine (SPARC)] receptor secreted on the surface of tumor cells can promote preferential uptake of albumin [29, 30] and albumin nanoparticles [31]. SPARC as a major modulator of extracellular matrix proliferation and cell migration was showed to be associated with increased tumor invasion and metastasis in various tumor types [30]. As an efficient energy and nutrition source, albumin can be actively consumed by growing tumors to support amino acid metabolism of cells [32]. Moreover, albumin NSPs can penetrate and concentrate in cancer cells due to enhanced permeability and retention (EPR), what can be called passive targeting. Accumulation of NSPs takes place at tumor sites with leaky vessels, and tumor tissues show selective extravasation and retention of macromolecules and NSPs > 10 nm in a tumor [30]. The sum of these data albumin can be considered an excellent potential carrier for the drug delivery to tumor cells [33]. Albumin is considered an effective carrier also due to that albumin consumption is increased at active sites of inflammation (as well as cancer cells, synovial cells use albumin as nitrogen and energy source), therefore hypoalbuminemia develops [34].
The pull of works in PubMed database on conjugates and ligands, NSPs for targeting (3,722 works by the end of 2024) contains approximately 4% of studies devoted to albumin and approximately 8% of studies addressed to folate (Figure 1A). Among the works of this pull, in the last 10 years, the amount of works devoted to folate and albumin simultaneously accounts for approximately 15% of the total number of papers devoted to albumin and approximately 9% of the total number of papers devoted to folate (Figure 1B). There is an increasing trend of research related to folate-albumin-NSPs compared to research with and without relation to NSPs, i.e. in 2024 the portion of the papers related to folate-albumin-NSPs compared to research with and without relation to NSPs was 67% in PubMed (or 77% in Google Scholar), while the amount of works devoted to folate-albumin-NSPs is approximately 1% (or approximately 6% in Google Scholar) of the total number of papers devoted to conjugates and ligands, NSPs for targeting.
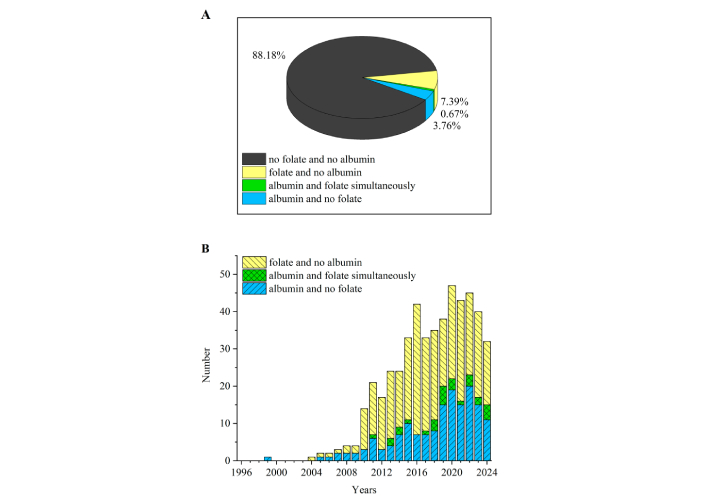
Targeting trends in folate/albumin-modified nanosized/submicron systems via ligand binding/conjugation. (A) Pie graph; (B) histogram. The numbers are taken from PubMed database due to searches “(conjugation OR ligand) (nano OR submicron) targeting folate”, “(conjugation OR ligand) (nano OR submicron) targeting albumin”, “(conjugation OR ligand) (nano OR submicron) targeting folate albumin” and “(conjugation OR ligand) (nano OR submicron) targeting”
In the present review, we demonstrate a variety of folate-functionalized albumin-containing nano- and submicron delivery systems for therapy and diagnostics in terms of their application, composition, and pharmacokinetic parameters.
Conjugation of FA residue to serum albumin
Conjugation of FA residue to serum albumin is used for functionalization of albumin-containing systems with three different types of complexity. The examples of the conjugation for obtaining folate-albumin-NSPs are presented in Figure 2.
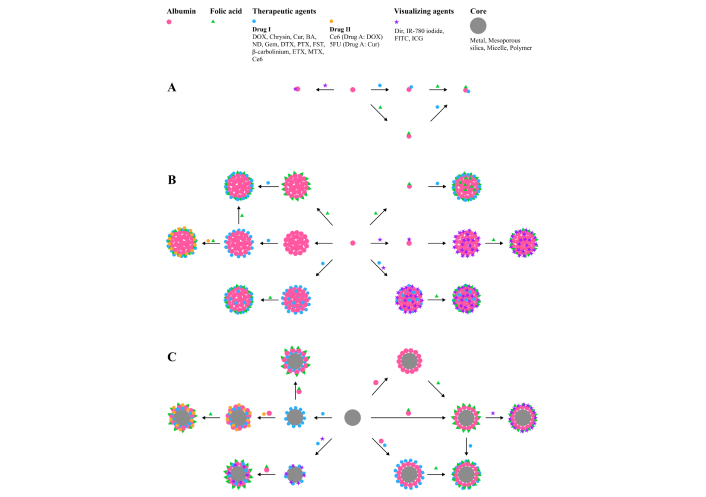
Examples of the ways of obtaining folate-albumin conjugate modified with visualizing agents and drugs [Drug I or Drug II (added after Drug I), covalently or non-covalently bound to albumin or shell@core NSPs]. (A) Based on protein individual molecules; (B) based on protein NSPs; (C) based on other (particularly shell@core) NSPs. DOX: doxorubicin; Cur: curcumin; BA: baicalin; ND: nintedanib; Gem: gemcitabine; DTX: docetaxel; PTX: paclitaxel (Taxol®); FST: fisetin; ETX: etoricoxib; MTX: methotrexate; Ce6: chlorin e6; 5FU: 5-fluorouracil; Dir: dimethylindole red; IR-780 iodide: 2-[2-[2-Chloro-3-[(1,3-dihydro-3,3-dimethyl-1-propyl-2H-indol-2-ylidene)ethylidene]-1-cyclohexen-1-yl]ethenyl]-3,3-dimethyl-1-propylindolium iodide; FITC: fluorescein isothiocyanate; ICG: indocyanine green; NSPs: nanosized and submicron particles (or nano- and submicroparticles)
The simplest systems consist of individual albumin molecules coupled with therapeutic, visualizing, or other agents with specific action without any other modifications of albumin [35]. Another type of system includes cross-linked albumin nanoparticles with chemicals [36–40]. In the third type of system, albumin is used as coverage (coating, shell) for nanoparticles (particularly inorganic). The last type currently receives great interest due to the fact that it offers various functions of NSPs to combine the merits of all components and to mitigate the possible drawbacks of bare nanoparticles, particularly magnetic [41–43].
There are plenty of studies devoted to FA non-covalent binding to serum albumin, but it was demonstrated that systems with non-covalent bound FA are not stable enough in physiological conditions [44], while covalent binding of FA to albumin results in significantly better extent of binding and uptake of systems to cancer cells than physical binding [45].
Conjugation of FA to albumin is usually carried out via carbodiimide reaction. Carbodiimides belong to the kind of zerolength crosslinkers [46] which induce the formation of a covalent bond between carboxylates and primary or secondary amines without becoming part of the final amide-bond crosslink between the target molecules. The carbodiimide reaction takes place between the folate carboxy group and the amino groups of proteins [36–39, 45, 47–58], in some cases this is a reaction between folate amino groups and free carboxylic groups of proteins [35, 59]. In the carbodiimide reaction different diimide reagents [N-(3-dimethylaminopropyl)-N-ethylcarbodiimide (EDC) [36, 39, 40, 45, 49–51, 60]; N,N'-dicyclohexylcarbodiimide (DCC) [37, 52–56]; 1-ethyl-3-(3-dimethylaminopropyl) carbodiimide (EDAC) [38] or (EDCI) [58], etc.] are used. There are several main ways of conjugation between FA and albumin leading to different products. According to our analysis, the most popular ways are when in the carbodiimide reaction FA in the form of N-hydroxysuccinimide (NHS)-ester, is conjugated with albumin (Figure 3A). Interaction of NHS-esters with albumin firstly leads to the formation of a bond between the carboxylic group of the NHS-ester and amine group of protein [40] via acylation of nucleophilic residues of albumin, in particular its N-terminus and lysine residues [61, 62]. The less common but feasible way of conjugation of FA to serum albumin—a carbodiimide reaction between amino groups of FA and carboxylic groups of albumin—takes place with formation of folate-albumin conjugate of another type (Figure 3B). In this way, albumin can be taken in the form of NHS-ester [59]. Since L-glutamic acid of FA can stay at the FR pocket entrance, α-carboxylic of the glutamic acid is involved in coupling with FR [63]. Thus, it should be noted that binding affinity between FR and folate-albumin conjugate will depend on type of conjugate between FA and serum albumin [64].
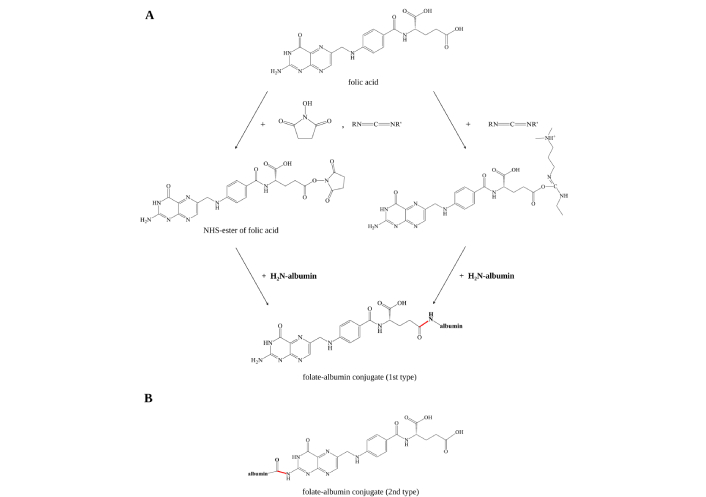
Schematic illustration of folate-albumin conjugate synthesis methods. (A) Widespread synthesis involving formation of NHS-ester or O-acylisourea active ester of FA residue is formed under influence of carbodiimide reagents [EDC, DCC, EDAC (or EDCI)] (on the scheme the reagents are RN=C=NR') and NHS, followed by formation of amide bond between carboxylic group of FA residue and amino group of albumin; (B) the second type of folate-albumin conjugate involves carboxylic group of albumin and amino group of FA. NHS: N-hydroxysuccinimide; EDC: N-(3-dimethylaminopropyl)-N-ethylcarbodiimide; DCC: N,N'-dicyclohexylcarbodiimide; EDAC (or EDCI): 1-ethyl-3-(3-dimethylaminopropyl) carbodiimide; FA: folic acid
HSA contains 59 lysine residues (more than 10 of them are available on the surface [65]), while bovine serum albumin (BSA) contains 58 lysines. Varanko and coauthors noticed that Lys199 is the most reactive lysine residue for conjugation of NHS-esters with human albumin [65], while for ketoprofen-N-hydroxysuccinimidyl ester (equivalent to glucuronide) in a model experiment, there were easily identified 11 ketoprofen-adduction sites (at Lys137, Lys195, Lys199, Lys212, Lys351, Lys402, Lys432, Lys436, Lys525, Lys536, and Lys541) [66]. Using mass spectrometry, it was shown that 6 lysine residues (Lys261, Lys350, Lys413, Lys431, Lys471, Lys474) located on BSA molecule surface are involved in interaction with NHS-ester of FA [67]. After formation of amide bonds through carbodiimide coupling the other amino acids located in the neighborhood of lysins and N-terminus are involved in reaction with NHS-ester [65]. In this reaction serine, tyrosine and threonine, arginine, histidine are reported to be involved [61] if they are available on the protein surface. For a mixture of bovine albumin and NHS-ester of FA, it was shown an engagement in interaction via different mechanisms of the following listed in the brackets amino acid residues: hydrogen bonding (Tyr149, Tyr340, Glu152, Glu339, Gln220, His289, Val342), π-π interaction (Tyr156), hydrophobic interaction (Lys187, Arg194, Arg256) [40].
Systems formed after coupling of NHS-ester of FA and albumin (BSA or HSA) in different forms (individual albumin; albumin in form of protein nanoparticles; albumin on the surface of organic, inorganic or in hybrid particles) are often characterized using methods of UV-Vis spectroscopy [36, 38, 40, 50, 52, 54, 58] and Fourier transform infrared spectroscopy (FTIR) [37, 40, 49, 51, 58, 68]. Methods of size exclusion chromatography [45], high-performance liquid chromatography (HPLC) [69], and gel-filtration chromatography [50, 58] followed by UV-Vis spectroscopy; differential scanning calorimetry (DSC) [40, 51]; ultraviolet matrix-assisted laser desorption/ionization time-of-flight mass spectrometry (UV-MALDI-TOF MS) [35] are also used. Seldom studies include quantification of conjugating via additional chemicals and calculation of: amino groups of albumin through UV-Vis spectroscopy [45] and defined amino acids using fluorescence [70, 71]. Direct biological confirmation of FA-albumin conjugation is also used when NSPs are created for subsequent biomedical usage. The synthesized systems can be tested on biological in vivo and in vitro models, particularly cell lines. For example, folate-modified systems are compared with ones without folate or the differences of an effect of folate-modified systems on cells with and without FR overexpression are compared. The main ways of biological confirmation of folate-albumin conjugation we given below.
Application of NSPs containing serum albumin and FA residue
Among NSPs containing serum albumin and FA residue (folate-albumin-NSPs), in most cases, FA residue is covalently bound to NSPs or albumin. The variety of applications of NSPs with albumin and folate in covalently bound form is listed in Table 1 and the advantages of different folate-albumin systems (conjugates, albumin particles, and shell@core structures) are listed in Table 2. The therapeutic agents could be bound covalently (for example, β-carbolinium derivates [35], chlorin e6 (Ce6) [59, 72], 5-fluorouracil (5FU) [69], doxorubicin (DOX) [58]) and non-covalently (for example, DOX [72], paclitaxel [54]) bound to NSPs.
Application of NSPs containing FA residue and albumin
Whole system name | Preparation of system | FR-positive cell lines, with which systems were tested | Application | Method to confirm the binding | Size/diameter, nm | Reference |
---|---|---|---|---|---|---|
Breast cancer | ||||||
Chrysin-BSA-FA NPs | BSA NPs loaded with chrysin and conjugated with FA in form of NHS-ester | MCF-7 | chemotherapy | FTIR, DSC | 97.5 ± 5.8 nm (DLS) | [51] |
FA-BSA-LC/DOPE-PTX | pH-sensitive lipoprotein-mimic nanocarrier with core from DOPE and oleic acid containing paclitaxel and conjugated with FA in form of NHS-ester | MCF-7 | chemotherapy | UV-Vis | 116.10 ± 2.21 nm (DLS) | [50] |
FA-BSANPs/BA | BSA was conjugated with FA in a form of NHS-ester, then NPs were synthesized and they were loaded with BA | MCF-7 | chemotherapy | UV-Vis, FTIR | 228.41 ± 2.36 nm (DLS) | [39] |
ND-FA-BSA NSPs | BSA was conjugated with FA in form of NHS-ester, then microspheres were synthesized and they were loaded with ND | MCF-7 | chemotherapy | - | 764.68 ± 88.46 nm (DLS), approximately 760.00 nm (SEM) | [71] |
FA-Gem-BSA NPs | BSA NPs with Gem were conjugated with FA in a form of NHS-ester | MCF-7 | chemotherapy | UV-Vis | 208.70 ± 1.80 nm (DLS) | [56] |
(BSA)-cis-aconitic anhydride-DOX | DOX prodrug attached to BSA through pH-sensitive linker (cis-aconitic anhydride), this system was conjugated with FA in form of NHS-ester | MCF-7 | chemotherapy | FTIR, UV-Vis | 20 ± 4 nm (LPSA and TEM) | [58] |
DTX-HSA-FA and DTX-HSA-biotin | FA in form of NHS-ester was conjugated with HSA, then system was bonded with DTX | MDA-MB-231, 4T1 | chemotherapy | UV-Vis | 185 nm (DLS) | [52] |
FA-DOX-BSA-IR-780 iodide | BSA NPs were loaded with DOX and IR-780 iodide and then conjugated with FA in a form of NHS-ester | m109 | combined chemotherapy and PTT | - | 178.00 ± 5.82 nm size (DLS) | [73] |
Cervical cancer | ||||||
Mn: CuSe@BSA-FA-Ce6 | Manganese-doped copper selenide nanoparticles (Mn: CuSe) coated with BSA conjugated with Ce6 and FA with subsequent conjugation | HeLa | theranostics (image-guided cancer therapy PPT, PDT, MRI) | UV-Vis | 40 nm (DLS) | [59] |
FA-BSA NPs with DOX | BSA NPs were loaded with DOX. FA was activated with EDAC and conjugated to BSA | HeLa | chemotherapy | UV-Vis | from 176.2 to 191.6 nm (DLS) depending on conditions of preparation of the system | [38] |
FA-BSANPs with FST | BSA conjugated with FA in form of NHS-ester, then NPs were synthesized and they were loaded with FST | HeLa | chemotherapy | FTIR, UV-Vis, DSC, XRD | 150 nm (DLS), 109 nm (FE-SEM) | [40] |
FA-Ce6/DOX/BNPs | BSA NPs with DOX, Ce6 were conjugated with FA in form of NHS-ester | HeLa | combined chemotherapy and PDT | FTIR | 103.5 ± 3.8 nm (DLS) | [72] |
FA-BSA-AuNSs | AuNSs covered with BSA were conjugated with FA in form of NHS-ester | HeLa | PTT | FTIR, UV-Vis | 169.7 ± 11.3 nm (DLS); 100.0 ± 24.4 nm (TEM) | [49] |
HSA-DOX-Au NR NPs with FA | HSA NPs were conjugated with FA activated under EDC and connected with Au NR and DOX | HeLa | combined chemotherapy and PDT | UV-Vis | 50 nm (DLS) | [74] |
(BSA)-cis-aconitic anhydride-DOX | DOX prodrug attached to BSA through pH-sensitive linker (cis-aconitic anhydride), this system was conjugated with FA in form of NHS-ester | MDA-MB-231, BEL-7402 | chemotherapy | FTIR, UV-Vis | 20 ± 4 nm (LPSA and TEM) | [58] |
Ovarian cancer | ||||||
FA-Gem-BSA NPs | BSA NPs with Gem were conjugated with FA in a form of NHS-ester | Ovcar-5 | chemotherapy | UV-Vis | 208.70 ± 1.80 nm (DLS) | [56] |
FA-BSA-MNP | Iron oxide MNPs were coated with BSA to form BSA-MNP, FA was conjugated to the BSA-MNP in a form of FA-PEG2K-NHS | SKOV3 | diagnostics (separating cells from blood for indentification via immunocytochemical staining) | FTIR | 120.2 nm (for BSA-MNP) (DLS) | [68] |
Prostate cancer | ||||||
FA-BSA NPs with PTX | BSA NPs with FA in form of NHS-ester and PTX | PC-3 | chemotherapy | UV-Vis | 217.0 ± 3.6 nm (LLS) | [54] |
Nasopharyngeal tumor | ||||||
FA-DOX-BSA MNPs | MNP from Fe3O4 with addition of BSA and DOX and conjugated with FA in form of NHS-ester | KB | combined chemotherapy and MH | - | 180.0 ± 2.2 nm (DLS) | [55] |
Gastric cancer | ||||||
HMSN-BSA-FA with ICG and PTX | HMSN coated with BSA conjugated with FA in form of NHS-ester and loaded with ICG and PTX | SGC-7901, MGC80-3 | combined chemotherapy and PDT | UV-Vis, FTIR | 241.4 ± 6.0 nm (DLS); 139.4 ± 7.0 nm (TEM) | [57] |
Colorectal cancer | ||||||
GO-Albumin-Cur-FA-5FU | Graphene oxide NPs conjugated with Cur, mixture of 5FU and albumin, and finally conjugated with FA activated by EDC | HT-29 | chemotherapy | HPLC | - | [69] |
Cur-HSA NPs with FA | HSA NPs loaded with Cur and conjugated with FA in form of NHS-ester | HT-29 | chemotherapy | - | 165.6 ± 15.7 nm (LLS) | [53] |
Liver cancer | ||||||
FA-BSA SPIONs with polymeric micelles | Polymeric micelles functionalized with BSA conjugated with FA activated under addition of EDC and loaded with SPIONs | Bel-7402 | diagnostics | FTIR | 196.1 nm (DLS) | [28] |
Other carcinomas | ||||||
folate-BSA-β-carbolinium derivates | BSA covalently linked to photosensitizers (β-carbolinium derivates). FA activated by diimide reagent was added to BSA-β-carbolinium | KB | PDT | UV-MALDI-TOF MS | - | [35] |
FA-Dir-BSA NPs | BSA NPs with Dir were conjugated with FA in form of NHS-folate | KB | cell-targeted imaging | UV-Vis | 120 nm (TEM) | [36] |
Brain tumors | ||||||
FA-HSA NPs | HSA NPs with conjugated with FA activated with EDC or adsorbed FA | UKF-NB-3, 101/8 | chemotherapy | Size exclusion chromatography | 239 ± 26 nm for conjugated FA (DLS); 280 ± 92 nm for NPs with adsorbed FA (DLS) | [45] |
BSA-SPIONPs with FA and FITC | Iron oxide nanoparticles coated with BSA and conjugated with FA in form of NHS-ester. Then FA-BSA-SPIONPs were labeled with FITC | U251 | diagnostics, detection | - | from 15 to 20 nm for SPIONS without modifications (TEM) | [75] |
Rheumatoid arthritis | ||||||
FA-ETX-BSA NPs | ETX-BSA NPs conjugated with FA in a form of NHS-ester | - | treatment of rheumatoid arthritis | FTIR | 215.8 ± 3.2 nm (DLS) | [37] |
MTX-BSA-FA NSPs | BSA NSPs coupled with MTX and conjugated with FA in form of NHS-ester | - | treatment of rheumatoid arthritis | UV-Vis | 73.59 ± 19.47 nm (AFM); 84.66 ± 16.85 nm (SEM); 157.48 ± 27.47 nm (DLS) | [76] |
FA-HSA NCs with FITC | HSA NCs labeled with FITC conjugated with FA in a form of NHS-ester | - | treatment of rheumatoid arthritis | UV-Vis | 443.5 ± 9.0 nm (DLS) | [6] |
5FU: 5-fluorouracil; AFM: atomic force microscope; Au NR: gold nanorods; AuNSs: gold nanostars; BA: baicalin; BSA: bovine serum albumin; BSANPs: bovine serum albumin nanoparticles; Ce6: chlorin e6; Cur: curcumin; DSC: differential scanning calorimetry; FE-SEM: field emission scanning electron microscopy; Dir: dimethylindole red; DOPE: 1,2-dioleoyl-sn-glycero-3-phosphoethanolamine; DOX: doxorubicin; DLS: dynamic light scattering; DTX: docetaxel; EDAC: 1-ethyl-3-(3-dimethylaminopropyl) carbodiimide; EDC: N-(3-dimethylaminopropyl)-N-ethylcarbodiimide; ETX: etoricoxib; FA: folic acid; FITC: fluorescein isothiocyanate; FR: FA (or folate) receptors; FST: fisetin; FTIR: Fourier transform infrared spectroscopy; Gem: gemcitabine; GO: graphene oxide nanoparticles; HMSN: mesoporous silica nanoparticle; HPLC: high-performance liquid chromatography; ICG: indocyanine green; IR-780 iodide: 2-[2-[2-Chloro-3-[(1,3-dihydro-3,3-dimethyl-1-propyl-2H-indol-2-ylidene)ethylidene]-1-cyclohexen-1-yl]ethenyl]-3,3-dimethyl-1-propylindolium; LLS: laser light scattering; LPSA: laser particle size analyzer; MH: magnetic hyperthermia; MNP: magnetic nanoparticles; MRI: magnetic resonance imaging; MTX: methotrexate; NCs: nanocapsules; ND: nintedanib; NHS: N-hydroxysuccinimide; NPs: nanoparticles; NSPs: nanosized and submicron particles (or nano- and submicroparticles); PDT: photodynamic therapy; PEG2K: polyethylene glycol with nominal Mw 2,000 Da; PTT: photothermal therapy; PTX: paclitaxel (Taxol®); SPIONs (or SPIONPs): superparamagnetic iron oxide nanoparticles; SEM: scanning electron microscopy; TEM: transmission electron microscopy; UV-MALDI-TOF MS: ultraviolet matrix-assisted laser desorption/ionization time-of-flight mass spectrometry; UV-Vis: UV-Vis spectroscopy; XRD: X-Ray diffraction; -: not applicable. In the table we use abbreviations of the researchers despite in some cases the term “nanoparticles (NPs)” is used for submicroparticles
Advantages of different folate-albumin systems (conjugates, albumin particles, and shell@core structures) depending on their composition
Property | Folate-albumin conjugate![]() | Folate-albumin NSPs![]() | Folate-albumin shell@core NSPs![]() |
---|---|---|---|
Tumor-targeting properties | |||
targeting via folate receptors and albumin-binding receptors (Gp18, Gp30, Gp60, and SPARC) | + | + | + |
albumin as a resource of amino acids and energy for cells | + | + | + |
Performance of the systems in vivo | |||
biocompatibility | + | + | + |
biodegradability | + | + | +/– |
low immunogenicity | + | + | + |
long half-life, prolonged circulation in bloodstream | + | + | + |
minimal toxicity | + | + | +/– |
reduced thrombogenic activity | + | + | + |
colloidal stability | + | + | + |
Areas of application | |||
ligand-derived applications (drug transport, PDT, visualization, etc.) | + | + | + |
core-derived applications (MRI, magnetic hyperthermia, etc.) | N/A | N/A | + |
MRI: magnetic resonance imaging; NSPs: nanosized and submicron particles (or nano- and submicroparticles); PDT: photodynamic therapy; SPARC: secreted protein acidic and rich in cysteine; N/A: not applicable
Analysis of different studies helps to reveal that biomedical effectiveness of FA residue as targeting agent is confirmed mainly through in three ways: (1) comparison of folate-albumin-NSPs and non-modified albumin-NSPs on FR-positive cells [35–40, 49–51, 53–58, 69, 72, 75, 76]; (2) analyzing of folate-albumin-NSPs influence on cells with different amount of FRs [59, 68]; (3) comparison of folate-albumin-NSPs containing drug with the individual drug on FR-positive cells [52, 58, 71]. In all considered studies, FA residue acts as a targeting agent, which significantly improves targeting properties of NSPs by FR-specific endocytosis [77]. Nevertheless, it is worth noting that the NSPs without folate also can be characterized by enhanced targeting resulting from EPR effect, while retention effect is due to glycoprotein trap in cancer cells [78]. Combination of the EPR effect with active targeting is one of the important advantages of folate-albumin-NSPs in cancer diagnosis and therapy.
Additional nanosized cores in the biomedical delivery systems can improve their ways of application. Particularly, magnetic cores can make the systems applicable in MRI, magnetic hyperthermia (MH) (heating under the action of alternating fields) to tumors, and make possible magnetic concentrating in tumor tissues (due to magnetic characteristics of particles).
Most of the elaborated delivery systems including serum albumin and FA residue are developed for cancer therapy [including chemotherapy, photothermal therapy (PTT), photodynamic therapy (PDT), and combined therapy] and imaging. A minor part of the work is devoted to treatment of other diseases, particularly rheumatoid arthritis.
All the examples will be given below.
Folate-albumin-NSPs for cancer chemotherapy
The delivery systems, which include FA conjugated to albumin (HSA or BSA), developed for cancer chemotherapy traditionally contain a single treating agent. The complex particles often include organic or inorganic nano cores. Conventional anticancer drugs (i.e. DOX [79], docetaxel (DTX) [52], paclitaxel [54], baicalin [39], nintedanib [71], gemcitabine [56]) and other biologically active substances with antitumor activity (i.e. curcumin [53, 69], fisetin (FST) [40], 5FU [69]) are widely used as anticancer agents. The use of FA as a component increases the effectiveness of targeting of these drugs and decreases their side effects (that can take place due to non-targeted distribution of the hybrid system). Furthermore, incorporation of drugs to folate-albumin-NSPs improves water solubility of the drug, for instance, nintedanib [71].
Sometimes hybrid systems for chemotherapy have the ability to release a treating agent in a pH-dependent manner (it is well-known that the microenvironment of tumors produces acidic pH values [80]). The pH-dependence of properties of the systems with FA residue can be reached due to addition of pH-sensitive linker between agent and albumin [58] or by using a pH-sensitive nanocarrier [i.e. lipid core formed from 1,2-dioleoyl-sn-glycero-3-phosphoethanolamine (DOPE) and oleic acid] [50].
Several studies in breast cancer cell lines noted superior cytotoxic effect [51, 52, 56], enhanced cellular uptake of folate-albumin-NSPs by FR-positive cells [39, 49, 56, 71], increased inhibition rates [71] and improved in vitro targeting ability [58]. Tests in vivo showed for folate-albumin-NSPs in comparison with albumin-NSPs (systems with FA compared to ones without FA) inhibition of tumor growth [39, 50, 52, 58], tumor size reduction and increasing the survival rate of mice with tumors for folate-albumin-NSPs [52].
Folate-albumin-NSPs for PTT and PDT
PDT is a noninvasive treatment based with the use of light-sensitive substances (photosensitizers). Irradiation using certain wavelength of light leads to the generation of reactive oxygen species (ROS) resulting in apoptosis or necrosis of cells in target tissues [81]. The example of folate-albumin-NSPs for PDT therapy of human carcinomas was developed by [35] with polar β-carboline derivatives inducing DNA damage.
Another type of tumor therapy is PTT based on the usage of photothermal agent absorbing near-infrared (NIR) light and converting this light into heat-inducing thermal ablation of the tumors. Like PDT, PTT is a low-damaging kind of treatment in terms of side effects.
Li and colleagues [49] used gold nanostars (AuNSs) as agents with high photothermal conversion efficiency for the targeted photothermal ablation of cervical cancer cells. It was shown that AuNSs strongly absorbed NIR light with temperature increase in its microenvironment. The BSA-FA-AuNS system demonstrated great potential as a PTT conversion agent for the receptor-mediated treatment of cancer cells.
Folate-albumin-NSPs for combined therapy
Combination of different therapies improves therapeutic efficiency of the hybrid systems. Particularly synergistic anti-tumor effects can take place [72–74].
Combined chemotherapy and PDT are used, for example, for treatment of cervical cancer [72, 74] and gastric cancer [57]. Lee and researchers [72] used Ce6 as a photosensitizer and accompanied PDT with DOX treatment, Encinas-Basurto and colleagues [74] used gold nanorods (Au NR) for PTT and for DOX chemotherapy, Zhang and Liang [57] used indocyanine green (ICG) as photosensitizer and paclitaxel (Taxol®) (PTX) as chemotherapy. These authors [57] developed the system including hollow mesoporous silica nanoparticles (HMSNs) [57] loaded with DOX and ICG. ICG has an exceptional ability to convert absorbed light to ROS, furthermore, ICG is an NIR fluorescence dye and is shown to allow imaging of tumors and tissues (for instance, it can be used for real-time monitoring during surgical procedures [82]). Zhang and Liang [57] connected folate-conjugated BSA with HMSNs via imine bonds which were pH/redox sensitive. After the addition of these folate-albumin-NSPs to the cells, the following changes were observed. Firstly, through FR-mediated endocytosis, the systems entered the cell. Secondly, under high glutathione concentration and acid environment the complex HMSNs-BSA was destroyed, the released ICG under irradiation produced ROS, a cytotoxic PTX further damaged the target cells and enhanced treatment efficacy.
Lee and coauthors [72] used BSA nanoparticles as a nanocarrier which was connected with FA, DOX, and Ce6. Fluorescence of Ce6 allowed to analyze uptake of the systems by HeLa cells using confocal microscopy. Also, Ce6 as a photosensitizer after irradiation induced ROS production in mitochondria leading to apoptosis and cell death. Slightly acidic conditions (around pH 6.5) in the tumor environment led to the BSA protonation. It is well-known that protonated BSA can attenuate binding between DOX and nanoparticles due to electrostatic and hydrophobic interactions [83], thus, DOX can be effectively released from hybrid systems.
The combination of PTT and chemotherapy is presented in a study by Li and colleagues [73], who developed a folate-albumin-NSPs for the treatment of breast cancer. This folate-albumin-NSPs containing BSA nanoparticles as a carrier of 2-[2-[2-Chloro-3-[(1,3-dihydro-3,3-dimethyl-1-propyl-2H-indol-2-ylidene)ethylidene]-1-cyclohexen-1-yl]ethenyl]-3,3-dimethyl-1-propylindolium iodide (IR-780 iodide) as a NIR fluorescent dye showed excellent photothermal effect. DOX as an anti-cancer drug and FA residue as a targeting ligand exhibited significant tumor inhibition efficacy without recurrent tumor growth in vivo. Additionally, fluorescent characteristics of IR-780 iodide allowed it to be used for visualization of affected areas.
Yang and coauthors [55] developed a folate-albumin-NSPs for combined chemotherapy and MH. Hyperthermia is an approach based on local heat generation in the tumor region through the direct delivery of magnetic nanoparticles (MNP) activated by exposure to an external alternating magnetic field. An additional antitumor effect is provided by DOX, which in sum with MH results in inhibition rate of tumor volume equal to 97% and inhibition rate of tumor weight equal to 77% in vivo.
Folate-albumin-NSPs for visualization
Another bunch of applications of NSPs, including shall@core structures, functionalized with FA residue, is the visualization and detection of tumor cells. These approaches can be based on the physical properties of magnetic particles in the hybrid systems (for example, MRI) or of additional substances, preferably ones with fluorescent activity allowing high-sensitivity determination of presence of tiny amounts of the substances in cells/tissues. Hereafter we will refer to two substances belonging to the group of dyes or labels, they are fluorescein isothiocyanate (FITC) and dimethylindole red (Dir).
It is worth noting that beyond visualization methods, MRI is the most abundant one [49, 59, 75] applied to detect cancer cells of tumors with different localization, particularly brain, liver, and breast cancers.
Some authors combined dual imaging properties inside a single system. Wang and colleagues [75] for MRI application incorporated SPIONs in their system and also labeled it with FITC. The usage of FITC allowed to visualize hybrid system after cellular uptake and internalization by glioma U251 cells. It was shown that this system was well suitable for both approaches (MRI and FITC-visualization) and also due to the presence of BSA in the composition allowed to bring different drugs and therefore could be applied for theranostics (also known as theragnostics, see Folate-albumin-NSPs for theranostics). There were systems that visualized tumors only through fluorescent dye—it was a platform in [36] for cell-targeted imaging of KB carcinoma cells involving application of Dir which was connected with BSA nanoparticles. It was shown that selective interaction of Dir with BSA enhanced its fluorescence up to 130-fold, therefore, the whole system possesses high fluorescence quantum yield, which enables targeted tumor imaging. In both studies, the fluorescence was detected through confocal laser scanning microscopy.
Moving to the studies based on the magnetic properties of particles the research of Li and coauthors should be mentioned [68]. This group developed system consisting of iron oxide MNP, BSA, and FA. They demonstrated that these folate-albumin-NSPs could effectively bind with the ovarian cancer SKOV3 cell line. The system allowed the capture of ovarian cancer circulating tumor cells from blood, which made the system suitable for noninvasive early-stage cancer diagnostics and detecting circulating tumor cells.
Folate-albumin-NSPs for theranostics
Less explored but very promising application of NSPs, including shall@core structures, functionalized with FA residues and containing serum albumin is theranostics integrating diagnostic imaging and treatment with various agents in single theranostic systems. An example of such a dual system is a nanohybrid created by Dehvari and colleagues [59] for theranostics of cervical cancer. In this system, manganese-doped copper selenide nanoparticles (Mn: CuSe) possess excellent biocompatibility and NIR absorption. Copper selenide nanoparticles play the role of PTT agent after irradiation in NIR region, Mn enhances the heat generating of the system and provides detection of this system by MRI. Looking at other components of this system, BSA offers an opportunity to bind various drugs and other biologically active molecules, Ce6 acts as a photosensitizer, and FA as a target molecule. Authors mentioned that the developed platform exhibits synergistic PTT/PDT effect in vitro, which holds the promise for image-guided cancer therapy since the systems are capable for MRI. The amount of injected drug needed to achieve theranostics goal due to target incorporation of folate-albumin-NSPs to cells via receptor-mediated endocytosis is decreased compared to the amount of drug needed without using folate-albumin-NSPs. The light-activated Mn: CuSe@BSA-FA-Ce6 platform can act as an on-demand treatment to eradicate the cancer cells remotely while the MRI contrast agent can detect particular tumor areas and monitor efficacy of treatment.
Folate-albumin-NSPs for inflammatory diseases
As it is described previously, FR receptors are overexpressed on the surface of activated macrophages and, therefore, FA can be used as a targeting molecule for autoimmune diseases such as rheumatoid arthritis. The conjugation of FA to albumin in form of NHS-ester allows to avoid usage of toxic cross-linkers and agents during preparation of the folate-albumin-NSPs for rheumatoid arthritis treatment [6]. Albumin is known to preferentially accumulate in the inflamed joints due to increased permeability of the blood vessels there [34].
In case of inflammatory diseases, folate-albumin-NSPs are used as carriers of therapeutic agents. For instance, Bilthariya and researchers [37] created folate-albumin-NSPs on the base on BSA nanoparticles, containing therapeutic drug etoricoxib (ETX) for its delivery to affected joints. One more example of application of BSA NSPs with FA has been investigated in study [76]. These researchers developed a hybrid system containing BSA particles conjugated with methotrexate (MTX) and FA. MTX is a drug that reduces the production of the pro-inflammatory cytokines which play a major role in inflammation processes and articular destruction which takes place during rheumatoid arthritis. In this system, FA acts as targeting molecule and reduces the toxicity, dose-dependent side effects, intestinal absorption problems caused by MTX, and extends short half-life of MTX.
Conclusions
Nano- and submicron delivery systems including conjugate of FA and serum albumin (folate-albumin-NSPs) are widely used in research devoted to medical purposes, particularly for disease treatment and diagnostics. The prevailing number of these systems are aimed at cancer therapy through additional chemical substances and/or physical effects (i.e. chemotherapy, PDT, PTT, and MH). Fewer studies have focused on the detection and visualization of tumor nodes and singular cells via MRI and/or additional labelling of NSPs with dyes. It is worth noting that such complex hybrid particles as folate-albumin-NSPs are vital for tumor types with FR overexpression which often is associated with worse outcomes of disease (i.e. breast cancer, metastatic squamous cell carcinomas). Among such upgraded systems, we can list the systems with dual targeting (when one of the biovectors is FA residue), stimuli-response systems [which change their state according to different characteristics of microenvironment (i.e. pH, composition, etc.)]. Furthermore, in recent years the number of complicated systems for combined cancer therapy is growing. In these modern systems, the sum of different therapy approaches synergistically enhances the effects of each one, which results in a significant increase in total effectiveness. Additionally, there are systems for theranostics allowing identifying the disease in its early stages, avoiding side-effects of drugs, and monitoring the effectiveness of the therapeutic agents and treatment process. Substantially lower amounts of studies have been focused on the development of treating systems for inflammatory diseases. In all these systems FA residue is used as a targeting agent which allows to enhance targeting of systems and decrease their toxicity and side-effects. Serum albumin is used as a biocompatible component carrying out drug transport and bringing additional targeting to delivery nanosystems. Using of folate residue and serum albumin contributes to advantages of NSPs. The use of additional nanosized cores in the biomedical systems can improve their applications. Particularly, magnetic cores can make the systems applicable in MRI, MH to tumors, magnetic concentrating in tumor tissues.
So, design of the new complex systems aimed at cancer treatment is currently actively progressing and the grade of complexity of systems is constantly increasing.
However, there are only a few FA-linked applications for targeted delivery of therapeutic agents that have been tested in the clinic, despite hybrid systems containing FA conjugated to albumin (particularly supramolecular systems) are promising agents for medicine, from diagnostics and therapy of cancer to treatment of inflammatory diseases. A number of underlying issues can be considered. First of all, complex hybrid particles consist of multiple components: carrier (or platform), targeting ligand, and pharmaсeutically active compounds. These complex systems are hardly reproducible when transferred from laboratory testing to commercial manufacturing. Secondly, clinical studies of FR-targeting have shown limited sensitivity and sensitivity as diagnostic tools, thus FR-targeting using FA residue in the folate-albumin NSPs is less specific than some other possible ways of targeting (that is confirmed by a constant level of studies devoted to folate-albumin NSPs compared to all NSPs for targeting: approximately 1% in PubMed or approximately 6% in Google Scholar).
The most common form in which FA is covalently bound with albumin is NHS-ester form, and we have briefly shown in the review the ways of folate-albumin conjugate obtaining by this and other paths of carbodiimide chemistry. Confirmation of FA residue conjugation to NSPs in the prevailing number of research was received through testing the synthesized systems on in vivo and in vitro models, including usage of cell lines. Additionally, some researchers characterized hybrid systems and/or folate-albumin conjugates with physical/physicochemical methods: UV-Vis spectroscopy, FTIR, DSC and X-ray diffraction, UV-MALDI-TOF MS, high-performance liquid and size exclusion chromatography. We suppose that a thorough analysis of modern studies (including those, characterizing quantitative parameters of binding) is undoubtedly needed for characterization of both non-covalent and covalent binding between FA and serum albumin. Such an analysis could reveal more patterns for design of folate-albumin NSPs that we consider rather important due to two reasons. Firstly, there are some examples of successful biomedical usage of FA and serum albumin both with and without their conjugation (hybridization), so further engineering of folate-albumin NSPs definitely makes sense. Secondly, conjugation between FA and albumin is just an example of ligand conjugation with albumin applicable for hundreds targeting agents and drugs.
Abbreviations
5FU: | 5-fluorouracil |
AuNSs: | gold nanostars |
BSA: | bovine serum albumin |
Ce6: | chlorin e6 |
Cur: | curcumin |
DCC: | N,N'-dicyclohexylcarbodiimide |
Dir: | dimethylindole red |
DOX: | doxorubicin |
DOPE: | 1,2-dioleoyl-sn-glycero-3-phosphoethanolamine |
DSC: | differential scanning calorimetry |
EDAC: | 1-ethyl-3-(3-dimethylaminopropyl) carbodiimide |
EDC: | N-(3-dimethylaminopropyl)-N-ethylcarbodiimide |
EPR: | enhanced permeability and retention |
FA: | folic acid |
FITC: | fluorescein isothiocyanate |
FR: | folic acid (or folate) receptors |
FTIR: | Fourier transform infrared spectroscopy |
HMSNs: | hollow mesoporous silica nanoparticles |
HSA: | human serum albumin |
ICG: | indocyanine green |
IR-780 iodide: | 2-[2-[2-Chloro-3-[(1,3-dihydro-3,3-dimethyl-1-propyl-2H-indol-2-ylidene)ethylidene]-1-cyclohexen-1-yl]ethenyl]-3,3-dimethyl-1-propylindolium iodide |
MNP: | magnetic nanoparticles |
MRI: | magnetic resonance imaging |
MTX: | methotrexate |
NHS: | N-hydroxysuccinimide |
NIR: | near-infrared |
NSPs: | nanosized and submicron particles (or nano- and submicroparticles) |
ODN: | oligodeoxyribonucleotides |
PDT: | photodynamic therapy |
PTT: | photothermal therapy |
PTX: | paclitaxel (Taxol®) |
ROS: | reactive oxygen species |
siRNA: | small interfering RNAs |
SPARC: | secreted protein acidic and rich in cysteine |
SPIONPs: | superparamagnetic iron oxide nanoparticles |
UV-MALDI-TOF MS: | ultraviolet matrix-assisted laser desorption/ionization time-of-flight mass spectrometry |
Declarations
Author contributions
MGG and AVB: Conceptualization, Writing—original draft, Writing—review & editing. AVT and MIA: Writing—original draft, Writing—review & editing. VSP: Writing—review & editing. DSK: Conceptualization, Writing—review & editing.
Conflicts of interest
The authors declare that they have no conflicts of interest.
Ethical approval
Not applicable.
Consent to participate
Not applicable.
Consent to publication
Not applicable.
Availability of data and materials
Not applicable.
Funding
The work was funded by the Russian Science Foundation (RSF) [grant number 22-75-10150]. The funders had no role in study design, data collection and analysis, decision to publish, or preparation of the manuscript.
Copyright
© The Author(s) 2025.
Publisher’s note
Open Exploration maintains a neutral stance on jurisdictional claims in published institutional affiliations and maps. All opinions expressed in this article are the personal views of the author(s) and do not represent the stance of the editorial team or the publisher.