Abstract
Severe acute respiratory syndrome coronavirus 2 (SARS-CoV-2) is a novel coronavirus that causes a global epidemic named COVID-19. It still continues to plague humans with severe complications and unique sequelae, causing huge economic losses in the world. Pathophysiological studies showed that important life organs, such as the lungs, brain, kidneys, heart, liver, and immune system, and even reproductive ones are affected directly or indirectly in patients with COVID-19. Classically and newly discovered drugs, concerning antiviral replication, anti-inflammation, blockage of pathogenic processes, alleviation of symptoms, and especially distinctive multi-actions of Traditional Chinese Medicine, were screened out and tested, presenting promising therapeutic effects on the virus before or even though abundant effective vaccines come out. Moreover, other strategies are underway, including the use of plasma therapy, monoclonal neutralizing antibodies, vaccine trials, and emerging drugs with distinct interference mechanisms. This review features the novel progress on the latest-discovered antiviral drugs and the effective Traditional Chinese Medicine, and highlights the advantages and shortages of different therapeutic strategies and the predicted potential targets of the used Traditional Chinese Medicine components, which provides a valuable reference for clinical treatment continuously to combat COVID-19.
Keywords
COVID-19, Western medicine, Traditional Chinese Medicine, therapeutic effect, adverse action, target moleculeIntroduction
COVID-19, a prevailing pneumonia disease in the world, is caused by a new coronavirus that has spread worldwide. Its common symptoms are cough, fever, and fatigue [1–3]. Fewer patients suffer myalgia, headache, sore throat, chest pain, and diarrhea, and nearly half patients may progress to shortness of breath [1, 4, 5]. Complications include acute respiratory distress syndrome (ARDS), arrhythmia, shock, RNAaemia, acute cardiac injury, and secondary infection [1, 2, 4]. Blood cell counting shows that the majority of the patients with COVID-19 exhibit lymphopenia [1, 2, 5–8]. The inflammatory cytokines like IL-6 and IL-10 [1, 3], lactate dehydrogenase, liver transaminase, C-reactive protein (CRP), D-dimer, and erythrocyte sedimentation are elevated in some COVID-19 patients [3, 5]. Most COVID-19 patients have pneumonia, and their chest computed tomography (CT) shows ground-glass opacity or consolidation [1, 2, 4, 8]. The source of infection is mainly from the patients with COVID-19 pneumonia. It can be transmitted from human to human with a wide range of age by respiratory droplets and close contact [9]. Clinical data showed that it takes 1–14 days (most 3–7 days) before symptoms onset [9], which makes defense work more difficult. The World Health Organization (WHO)’s report (2022) presented that there had been over 271 million confirmed patients with COVID-19 and more than 5.3 million deaths worldwide [10].
Genome sequencing showed that this new coronavirus shares nearly 80% identity with severe acute respiratory syndrome coronavirus (SARS-CoV) and 96% identity with a bat coronavirus [11, 12]. Thus, it was lastly designated as SARS-CoV-2 by Coronaviridae Study Group [13]. Coronavirus is an enveloped RNA virus that enters into target cells through first binding cell receptors, and then the clathrin-mediated endocytosis engulfs virus particles and forms a viral endosome [14]. This entering process requires protein spike, a glycoprotein on the viral surface [15, 16] to bind to the cell receptor angiotensin-converting enzyme 2 (ACE2) [17, 18], by which the virus enters into the target cells through a pH-dependent endocytotic reaction. As an RNA virus, SARS-CoV-2 is prone to mutation. Its mutant variants currently circulating around the world include gamma, delta, and omicron, which are more capable of spreading. More and more virus variants appear, further making treatment and prevention more difficult. Moreover, there have been outbreaks of SARS-CoV-2 with the peaks of infection within the past five years, hinting at its coexistence with humans like influenza.
According to reports, many COVID-19 patients may progress to ARDS and many other comorbidities, like hypertension, diabetes, secondary infection, coronary heart disease, and so on [1, 2, 4, 19], which are the main causes of death of COVID-19 patients. With the high transmission ability of SARS-CoV-2, the number of confirmed COVID-19 patients and their deaths increased rapidly. However, there are, to date, no specifically efficient treatment strategies for COVID-19, and the treatment for COVID-19 is mainly based on clinical experience. There are lots of experiments and clinical studies conducted to find efficient drugs and therapeutic routes against COVID-19. Some novel antiviral approaches are constantly emerging and their safety and effectiveness are being tested in clinical trials. Convalescent serum therapy for COVID-19 has been recently evaluated and a variety of vaccines are widely promoted globally. In this review, we systematically summarize current data against COVID-19 and especially feature novel progress on the latest-discovered antiviral drugs and effective Traditional Chinese Medicine and their predicted potential targets so as to provide a valuable reference for clinically treating COVID-19.
Antiviral drug
SARS-CoV-2 is an enveloped RNA virus, and its replication process requires several key enzymes, including RNA-dependent RNA polymerases (RdRps), 3 chymotrypsin-like cysteine protease (3CLpro), and papain-like protease (PLpro) [20–22]. There are compounds that inhibit SARS-CoV-2 enzymes and some of them show potential therapeutic effect. However, compounds that lack a biologically plausible mechanism of action would not usually be effective in clinical studies. Examples of these drugs included chloroquine analogs, favipiravir, and a long tail of other compounds. Besides, interferons (IFNs) are also important for combatting SARS-CoV-2, for IFN signaling pathways are activated in cells infected by many viruses, particularly coronaviruses [23, 24]. In Table 1, we listed the dosage, outcomes, and adverse effects of antiviral drugs, and described them in detail below.
Efficiency of antiviral drugs
Type of antiviral drugs | Antiviral drugs | Dosage | Duration (day) | Outcomes | References |
---|---|---|---|---|---|
Polymerase inhibitors | Remdesivir | 200 mg intravenously on day 1, plus 100 mg daily for the following 9 days | 10 | Significantly reduced the need for oxygen supportAdverse effects: increased hepatic enzymes, diarrhea, rash, renal impairment, and hypotension; multiple organ-dysfunction syndrome, septic shock, and acute kidney injury | [29] |
200 mg on day 1, followed by 100 mg daily | 5 or 10 | Improved clinical symptoms, over half cases were recovery clinically and over half cases were dischargedAdverse effects: nausea and acute respiratory failure | [30] | ||
200 mg on day 1 followed by 100 mg on days 2–10 in single daily infusions | 10 | No benefit in reducing the duration time of clinical symptoms and viral load, decreasing mortality, and decreasing the incidence of adverse eventsAdverse effects: gastrointestinal symptoms (anorexia, nausea, and vomiting), aminotransferase or bilirubin increases, and worsened cardiopulmonary status | [31] | ||
Molnupiravir | 800 mg twice daily | 5 | The risk of hospitalization or death in the placebo group was 9.7% (68/699), and the risk of hospitalization or death in the molnupiravir group was reduced to 6.8 % (48/709) | NCT04405739 | |
200 mg, 400 mg, or 800 mg, twice daily | 5 | 7.3% of patients (28/385) on molnupiravir as compared to 14.1% of patients on placebo (53/377) had either been admitted to the hospital or died, and no death was reported in the molnupiravir group as compared to 8 deaths in the placebo group on day 29Adverse effects: headache, insomnia, and increased levels of alanine aminotransferase (ALT) were the only adverse events reported by more than 4 participants, and 5% and 8.1% of molnupiravir and placebo groups, respectively showed grade 3 level of adverse events | NCT04575597 | ||
Ribavirin | 500 mg/time for adults, 2 to 3 intravenous infusions per day | < 10 | Contraindications and precautions: patients with cardiac diseases are forbidden treating with this drug; pay attention to the adverse effects and drug-drug interactions during treating with this drug | [54] | |
400 mg every 12 h | 14 | Accelerated the negative conversion time for severe acute respiratory syndrome coronavirus 2 (SARS-CoV-2) significantly | [55] | ||
600 mg, twice daily | 14 | No benefit for reducing the number of ICU admissions and the number of deaths, increased the cumulative incidence od recovery significantly | [56] | ||
500 mg every 12 h | - | No benefit for reducing the negative conversion time for SARS-CoV-2 and the mortality of the patients with severe COVID-19 | [57] | ||
600 mg every 12 h | 9 | One-third mortality of severe COVID-19Adverse effects: anemia, gastrointestinal disorders, liver enzymes increased, and sepsis | [58] | ||
Sofosbuvir/daclatasvir | 400/60 mg daily | 14 | Clinical recovery within 14 days was achieved by 29/33 (88%) in the treatment arm and 22/33 (67%) in the control arm (P = 0.076). The treatment arm had a significantly shorter median duration of hospitalization (6 days [Interquartile Range (IQR) 4–8]) than the control group [8 days (IQR 5–13)]; P = 0.029. The cumulative incidence of hospital discharge was significantly higher in the treatment arm versus the control (Gray’s P = 0.041). Three patients died in the treatment arm and five in the control arm. No serious adverse events were reported | [56] | |
400/60 mg daily with ribavirin 60 mg twice daily | 14 | The median duration of hospital stay for all patients was 5 days for the treatment arm and 9 days for the control arm. The need to admit patients to the ICU was lower in the treatment arm (17%) than the control arm (48%). The relative risk of ICU admission for the treatment arm versus the control arm was 0.36 [95% confidence interval (CI) 0.16–0.81, P = 0.014]. The median duration for ICU stay was 3.5 days for the treatment arm and 5 days for the control arm | [58] | ||
Protease inhibitors | PF-07321332 | 300 mg with ritonavir 100 mg every 12 h | 5 | The interim analysis showed an 89% reduction in risk of COVID-19-related hospitalization or death from any cause compared to placebo in patients treated within three days of symptom onset | [66] |
Lopinavir/ritonavir (LPV/r) | 400/100 mg | 10 | Improved clinical symptoms, decreased the need for oxygen support, improved pneumonia | [73] | |
400/100 mg, twice daily, or 800/200 mg daily | 10 | Shorter the duration time of fever and virus nucleic acid turned negative, decreased the denormalized rate of white blood cells, lymphocytes, C-reactive protein (CRP), and platelets | [74] | ||
400/100 mg, twice daily | 5–10 | Accelerated time of virus nucleic acid turned negative significantly, improved cheat computed tomography (CT) manifestations significantly, reduced the exacerbation rate, decreased the incidence of adverse events significantlyAdverse effects: elevated transaminase, elevated bilirubin, nausea, vomiting, abdominal pain, diarrhea, rash, etc. | [75] | ||
400/100 mg, twice daily | 10 | Reduced viral loads, improved clinical symptoms | [76] | ||
Darunavir | Darunavir/cobicistat: 800/150 mg daily | - | Reduced mortality significantly, increased the rate of cases discharged from ICU significantly, reduced the incidence of acute respiratory distress syndrome (ARDS) significantly | [78] | |
10 | SARS-CoV-2 nucleic acid test showed still maintained positive, chest X-ray showed pneumonia still existed, no benefit for improving the clinical symptoms of COVID-19 | [79] | |||
Darunavir/ritonavir: 800/100 mg daily | 5–10 | Adverse effects: liver enzyme elevations and mild diarrhea | [80] | ||
Type I interferons (IFNs) | Type I IFNs | 3 million IU/time, qod | Until two consecutive viral detections turned negative | The combination treatment shortened the average duration time of hospitalization significantly, accelerated the time of viral shedding of the upper respiratory tract | [86] |
-: no accurate datum
Polymerase inhibitor
Remdesivir
Remdesivir, an adenosine analog, was shown to have antiviral activity against the Ebola virus by inhibiting the replication of virus RNA in vitro and exhibited a broad-spectrum antiviral activity to many other pathogenic RNA viruses [25]. Molecular dynamics showed that remdesivir may inhibit SARS-CoV-2 via inhibiting RdRp [26], and virtual screening analysis displayed that the active form (CHEMBL2016761) of remdesivir has a high binding affinity with nucleoside triphosphate (NTP) [27].
WHO has announced that remdesivir is the most promising agent for COVID-19. Molecular dynamics reveals that it can stop SARS-CoV-2 virus RNA reproduction, acting as a terminator of the virus RNA-chain. Clinical data show that remdesivir can inhibit SARS-CoV-2 effectively with EC50 = 0.77 μM and EC90 = 1.76 μM [28]. Importantly, after the administration of remdesivir, the oxygen-support class of most patients with severe COVID-19 was improved [29]. Recent studies showed that the symptoms of COVID-19 patients were improved and their discharge time was shorter when they were administrated remdesivir within 10 days of the onset of the symptoms, compared to those treated after more than 10 days of the onset of the symptoms [30, 31], suggesting that early use of remdesivir for the treatment of COVID-19 would be better.
Molnupiravir
Molnupiravir, also known as MK-4482 or EIDD-2801, is an oral drug of cytidine analog. Its activity is primarily to cause viral mutagenesis by incorporating into new RNA strands [32, 33]. Deep sequencing studies have confirmed that viral mutagenesis is the main mechanism by which molnupiravir inhibits coronaviruses [33–35].
Molnupiravir has a broad-spectrum antiviral activity against multiple viruses, including SARS-CoV, MERS-CoV, and SARS-CoV-2. The dosage of less than 1 mM of molnupiravir is enough to reach EC50s [33, 36]. It can reduce virus levels and lung damage in mouse models of SARS-CoV and MERS-CoV [33, 37] and in hamsters [35] and ferret models [38] of SARS-CoV-2. Molnupiravir has been tested in phase II clinical trials in COVID-19 patients (NCT04405739). The results from the MOVe-OUT study showed that among all the tested patients (n = 1,408), the risk of hospitalization or death in the placebo group was 9.7% (68/699), and the risk of hospitalization or death in the molnupiravir group was reduced to 6.8 % (48/709). That is, the absolute risk is reduced by 3.0%, and the relative risk is reduced by 30%. Moreover, molnupiravir reduces the risk of hospitalization/death in all subgroups of patients, and the efficacy is not affected by the time of symptom onset and potential risk factors. According to the virus sequencing results of 40% of the patients, molnupiravir showed consistent efficacy against gamma, delta, and mu virus variants (NCT04575597) with a significant reduction in the risk of hospital admission or death by 50% (P = 0.0012) on day 29. It was found that 7.3% of patients (28/385) on molnupiravir had been admitted to hospital or had died, as compared to 14.1% of patients on placebo (53/377), and no death was reported in the molnupiravir group, as compared to 8 deaths in the placebo group on day 29. Moreover, the efficacy of molnupiravir was unaffected by the SARS-CoV-2 variants (gamma, delta, or mu), the time of onset of symptoms, and the underlying risk factors. The incidences of any adverse events (35% vs. 40% in molnupiravir vs. placebo arm) and the drug-related adverse events (12% vs. 11% in molnupiravir vs. placebo arm) were found to be similar in both the molnupiravir and placebo arm. Regarding tolerability, very few and low-grade adverse events were noted in this study. Headache, insomnia, and increased levels of alanine aminotransferase (ALT) were reported by more than 4 participants. 5% of the molnupiravir group and 8.1% of the placebo group had the grade 3 level of the adverse events, respectively. Two of one hundred forty patients (1.4%) in the molnupiravir group had adverse events, as compared to one of sixty-two patients (1.6%) in the placebo group, which led to therapeutic discontinuation [39, 40].
Although molnupiravir has shown promise against multiple RNA viruses, its application might be a risky strategy, as it could conceivably accelerate the emergence of new variants with a higher mutation rate of SARS-CoV-2 [41, 42]. However, there are no data that suggest the likelihood of this happening.
Ribavirin
Ribavirin, a triazole nucleoside [a US Food and Drug Administration (FDA)-approved drug], was found to have antiviral activities on both RNA and DNA viruses [43, 44], such as hepatitis E virus (HEV) [45, 46], human respiratory syncytial virus (RSV) [47], and hepatitis C virus (HCV) [48]. It was shown that ribavirin inhibited the biosynthesis of inosine monophosphate dehydrogenase in the virus-infected cells, resulting in the interruption of the replication of viral RNA and proteins [49]. The antiviral activity of ribavirin on coronavirus is mainly dependent on the specific inhibition of viral RdRp [50, 51]. Moreover, Ribavirin was ever used for the treatment of SARS-CoV, and it cured fever and pneumonia symptoms of SARS-infected patients [52, 53].
According to the guide of the seventh edition published by the National Health Commission of the People’s Republic of China, the dosage of ribavirin for the treatment of COVID-19 was 500 mg/time for adults and 2 to 3 intravenous infusions per day. It was recommended to be combined with IFN or lopinavir/ritonavir (LPV/r) [54]. Wang et al. [28] demonstrated that ribavirin could inhibit SARS-CoV-2 replication with EC50 = 109.50 μM in vitro. Clinical studies showed that the negative conversion time for SARS-CoV-2 was significantly shorter in the cases treated with the combination treatment of LPV/r, ribavirin and IFN-β 1b than in the cases treated with LPV/r only [55]. Another combination treatment strategy with sofosbuvir/daclatasvir/ribavirin also exhibited a higher cumulative incidence of recovery than standard care. There was no significant difference in ICU admission and the number of deaths between the two treatment strategies, though [56]. However, there was a difference in reducing negative conversion time for SARS-CoV-2 and the mortality of patients with severe COVID-19 between the treatment of ribavirin and the supportive care [57]. Another study showed that one-third of the mortality of severe COVID-19 patients treated with ribavirin and all patients had one or more adverse effects, of which anemia and gastrointestinal disorders were the most frequent [58].
Sofosbuvir/daclatasvir
Sofosbuvir, a nucleoside analog, or daclatasvir (a member of the class of biphenyls), is an orally available antiviral agent that inhibits the N-terminus of non-structural protein 5A (NS5A)/B RdRp of HCV and is used in combination with other oral antiviral agents to treat chronic HCV [59]. In light of the key role of RdRp in SARS-CoV-2 virus replication and the conservation of the sofosbuvir/daclatasvir binding residues in members of the beta-coronavirus genus, abundant laboratory research and clinical trials have been performed, suggesting that targeting RdRp is a promising treatment strategy for SARS-CoV-2 infection [56, 58, 60, 61]. One patient-level meta-analysis of three open-label studies on a total of 176 patients reported that the clinical recovery rate within 14 days was significantly greater in the patients receiving sofosbuvir/daclatasvir than in those receiving standard of care [56, 58, 62, 63].
Protease inhibitor
PF-07321332
PF-07321332 is a 3CLpro inhibitor [64, 65]. Its potential advantage is that it can have an effect on all current SARS-CoV-2 variants [65]. The IC50 of PF-07321332 against SARS-CoV-2 is 19 nM, and the EC50s in human airway epithelial cells, HeLa, and A549 cells that can express the ACE2 are 62 nM, 99 nM, and 56 nM, respectively [65]. The current phase I clinical trial (NCT04756531) for patients with mild and moderate COVID-19 is the combination therapy of PF-07321332 with ritonavir (paxlovid). In this trial, only three of the patients who took paxlovid within 3 days of the onset of symptoms required hospitalization, but no one died, and the hospitalization rate was only about 0.8%. Twenty-seven of the patients who took placebo required hospitalization, of which seven patients died unfortunately. Thus, the hospitalization rate is about 7% and the mortality rate is about 1.8%. It was shown that compared with placebo, the patients who took paxlovid within 3 days of the onset of symptoms had an 89% reduction in the risk of hospitalization. In addition, the proportion of treatment-emergent adverse events is almost similar, with about 19% for taking paxlovid and about 21% for taking placebo. As expected, the drug obtained an emergency use authorization (EUA) on December 22, 2021 [66].
LPV/r
LPV/r, a protease inhibitor composed of a mix of lopinavir and ritonavir with a fixed ratio, is more promising than lopinavir or ritonavir alone against virus infection [67]. LPV/r was ever used for the treatment of SARS-CoV and MERS-CoV [68–71]. Molecular docking showed that LPV/r could bind with SARS-CoV 3CLpro [72]. Sequence and structure analysis displayed a high similarity of 3CLpro between SARS-CoV and SARS-CoV-2 (PDB ID: 6LU7), suggesting the possibility of LPV/r for the treatment of COVID-19 through inhibiting 3CLpro.
Clinical usage of LPV/r (400 mg/100 mg) in COVID-19 patient showed improved dyspnea, attenuated lung lesions, and decreased CRP with the administration strategy for 10 days [73, 74]. Clinical reports from the General Hospital of Central Theatre Command demonstrated that with the administration of lopinavir 400 mg and ritonavir 100 mg twice a day for 5 to 10 days, the time of viral nucleic acid turning to negative and the time of pulmonary imaging improved in the patients were significantly shortened, compared to the control group [75]. Another reported case in South Korea showed that the viral load of a COVID-19 patient, who is 54 years old with intermittent fever and mild respiratory symptoms, was significantly decreased since the next day after being treated with LPV/r [76].
Darunavir
Darunavir is a protease inhibitor that is used for HIV infection. As its structure is similar to lopinavir, it was hypothesized to inhibit SARS-CoV-2 too. A computational work showed that darunavir has a significantly larger affinity with SARS-CoV-2 viral main protease (3CLpro), compared to compound 13b, which is a developed potent SARS-CoV-2 inhibitor [22, 77]. 800 mg daily darunavir significantly increased the survival rate of critically ill COVID-19 patients [78]. However, darunavir had no beneficial for inhibiting SARS-CoV-2 infection in HIV patients [79] or reducing mortality of severe COVID-19 patients [80].
Ebselen
Ebselen is a small molecule inhibitor of capsid protein in HIV-1 replication. It mainly inhibits the dimerization of the C-terminal domain (CTD) in the two alpha helix folding regions of capsid protein to prevent HIV-1 infection. In Vero cells infected with the COVID-19 virus, ebselen inhibited 3CLpro with an EC50 of 4.67 μM [81, 82]. It was being studied as an oral drug in two phase II placebo-controlled trials of patients with mild-to-moderate (NCT04484025) and severe (NCT04483973) COVID-19.
Type I IFNs
Type I IFNs (IFN-α/β) are shown a broad-spectrum antiviral activity on RNA viruses by regulating the immune response. Once humans are virus-infected, IFN-α/β can be recognized by IFN receptor (IFNR) and then fixed on IFNR, which leads to the activation of transcription factors and activates the IFN-stimulated genes, which may inhibit the replication of the virus [83]. Both IFN-α and IFN-β can inhibit SARS-CoV-2 replication in Vero cells [84]. The clinical medicinal value of IFNs includes prevention and treatment. The antiviral protein induced by IFN-α is stable in the body for many days, providing a basis for preventive medication. Immediate use of IFNs after early exposure is able to treat COVID-19 efficiently [85]. Moreover, a clinical study showed that the average duration time of hospitalization of the cases treated with IFN-α-2b combined with LPV/r was significantly shorter than that of the ones treated with LPV/r alone and that the combination treatment strategy accelerated the viral shedding in upper respiratory tract significantly [86].
Anti-inflammatory drug
Virus infection targets several systems in the human body, especially the immune system. Most of the COVID-19 patients present severe inflammatory damages in respiratory system organs with the phenotype of the cytokine storm. The patients infected with SARS-CoV-2 exhibited lymphopenia [1, 2, 5–7] and elevated inflammatory cytokine levels like IL-6 and IL-10 [1, 3], suggesting the importance of immune modulation in the treatment of COVID-19. Hence, in addition to drugs that directly target viral components, some anti-inflammatory drugs have played a role in clinical symptomatic treatment, increasing the recovery rate and reducing the mortality rate of the patients. The dosage, outcomes, and adverse effects of anti-inflammatory drugs are listed in Table 2 and described in detail below.
Efficiency of anti-inflammatory drugs
Type of anti-inflammatory drugs | Anti-inflammatory drugs | Dosage | Duration (day) | Outcomes | References |
---|---|---|---|---|---|
Glucocorticoids | Hydrocortisone, methylprednisolone, or dexamethasone | 80 mg of methylprednisolone sodium succinate per day for the first 3 days, followed by 40 mg per day during the following 3 days, and 20 mg per day for another 3 days before being discontinuedOne patient took it at the dosage of 40 mg per day for 5 days and 20 mg per day for another 5 days | 9 or 10 | Fever disappeared, clinical symptoms improved, chest computed tomography (CT) manifestations improved, severe acute respiratory syndrome coronavirus 2 (SARS-CoV-2) nucleic acid turned negative | [100] |
Median hydrocortisone-equivalent dose of 400.0 mg/day | The average time is 9.5 days | Improved oxygen saturation (SaO2) and arterial oxygen tension (PaO2)/inspiratory oxygen fraction (FiO2) in the first 3–5 days, inhibited furious inflammatory storm treated in the phase of acute respiratory distress syndrome (ARDS). No benefit for increasing survival rate | [99] | ||
1–2 mg/kg/day via intravenous injection | 5–7 | Accelerated the improved time of SpO2, accelerated the decreasing of the levels of C-reactive protein (CRP) and IL-6, shortened the duration time of ICU and hospitalization significantly, improved chest CT manifestations significantly | [97] | ||
- | 3 | Cough was relieved and chest CT manifestations were improved after 3 days of treatment, however, lymphocytes were still reduced, and the erythrocyte sedimentation rate was increased. Fever reoccurred accompanied by a paroxysmal cough, and the whole body’s skin was flushing. CT manifestation images showed exacerbated pneumonia. No benefit for SARS-CoV-2 shedding | [98] | ||
Statins | Atorvastatin, etc. | - | - | Statin was associated with decreased mortality in patients with COVID-19 [relative risk (RR) 0.72 (0.55, 0.95), P = 0.018; I-squared statistic (I2): 84.3%, P < 0.001]. The association of statin and mortality was not significantly affected by age, male gender, diabetes, and hypertension in this pooled analysis | [107] |
Inpatient statin use was associated with a significant reduction in mortality among COVID-19 patients especially those with diabetes mellitus (DM). Diabetic patients who received inpatient statins had significantly reduced mortality [odds ratio (OR), 0.35; 95% confidence interval (CI), 0.21–0.61; P < 0.001], reduced need for ICU admission (OR, 0.56; 95% CI, 0.34–0.90; P = 0.02) as well as a decreased need for mechanical ventilation (OR, 0.39; 95% CI, 0.23–0.65; P < 0.001) | [109] | ||||
Ivermectin | Tocilizumab | Ivermectin 6 mg stat and doxycycline 100 mg twice daily | 5 | Early improvement 60.7% vs. 44.4%, P < 0.03, deterioration 8.7% vs. 17.8%, P < 0.02 | NCT04523831 |
8 mg/kg by two consecutive intravenous infusions 12 h apart, a third infusion was optional based on clinical response | 10 | Improved respiratory functions, improved chest X-ray manifestations, 15% patients discharged from the hospital, improved the inflammatory statusAdverse effects: two patients developed septic shock and died, one had gastrointestinal perforation requiring urgent surgery and was alive on day 10 | [119] | ||
400 mg per time or 600 mg per time, median total dose of 5.7 mg/kg | 14 | Increased the rate of cases relieved fever, increased the rate of cases decreased the level of CRP, the rate of cases need oxygen support from 84% decreased to 28%, 68% cases improved chest CT manifestationsAdverse effects: 92% patients experienced at least one adverse event, the most frequent adverse events were anemia (16.64%), alanine aminotransferase (ALT) rise (11.44%), and QT interval prolongation (5.20%) | [120] | ||
4 mg/kg (400 mg), single infusion | - | Within 24 h, the patient’s oxygenation and hemodynamics improved, as evidenced by a pressure of oxygen to the FiO2 (P/F) ratio of 246. The patient was discharged on day 14 after the infusion of tocilizumab | [121] | ||
162 mg single dose | - | Fever disappeared in two days after the treatment of tocilizumab; the level of IL-6 was improved in a week after the treatment of tocilizumab; decreased the need for oxygen support; CT manifestation images showed improvement in pneumonia | [122] | ||
8 mg/kg, one dose | - | The patients improved rapidly after the treatment of tocilizumab; there is no need for oxygen support after 4 days of the treatment of tocilizumab; the patient was allowed discharged from ICU after 4 days of the treatment of tocilizumab; CT pulmonary angiography showed pneumonia improved | [123] | ||
First dose was 4–8 mg/kg body weight, and the recommended dose was 400 mg through an i.v. drip up to a maximum of 800 mg | - | 75% patients decreased the need for oxygen support in 5 days after the treatment of tocilizumab; CT manifestations of 90.5% patients showed pneumonia improved; the percentage of lymphocytes in peripheral blood was increased after the treatment of tocilizumab; abnormally elevated CRP decreased significantly in 84.2% patients; all patients have been discharged on average 15.1 days after giving tocilizumab | [124] | ||
8 mg/kg, with a second dose 12 h after the first and a possible third dose after a further 24–36 h, according to clinical response | - | The fever of the two cases disappeared in 3 days after the treatment of two dose tocilizumab, improved clinical status, improved the respiratory function, decreased the level of CRP to normal, consecutive test of SARS-CoV-2 nucleic acid of one case showed negative | [125] | ||
8 mg/kg, 2 doses, 12 h apart | - | Decreased CRP level and white blood cell count in 24 h after the treatment of tocilizumab; patient clinical condition progressively improved and ventilatory support was gradually weaned; chest CT showed mark improvement of CT findings on day 7 after the treatment of tocilizumab | [126] | ||
8 mg/kg, no more than 800 mg per time | - | Inflammation level was not significantly decreased, and lymphocyte and prothrombin time did not improve in the 3 patients treated with tocilizumab | [127] | ||
1 or 2 dose | - | Reduced death rate, reduced the duration time of ICU admission | [128] | ||
324 mg via subcutaneous route | - | Fever disappeared, arterial pO2 showed progressive improvement and oxygen treatment was stopped after the treatment of tocilizumab; IL-6 levels were increased at 6 days after administration of tocilizumab | [129] | ||
8 mg/kg tocilizumab (maximum dose 800 mg/day) repeated within 12 h from the first administration | - | Fever disappeared after the treatment of tocilizumab, decreased the level of CRPAdverse effects: high prevalence of candidemia occurred in the patients treated with tocilizumab in a very short period of time | [130] | ||
Cytokine inhibitors | Sarilumab | 400 mg was injected intravenously, one or two dose | - | Accelerated the clinical improvement time of COVID-19 patients with lung consolidation < 17% | [132] |
10 out of 15 critical ill COVID-19 patients survived, 5 patients were died; improved inflammatory status | [133] | ||||
Siltuximab | 11 mg/kg was injected intravenously over one hour, or a second dose was injected depending on the patient’s condition | - | Reduced 30-day mortality of COVID-19 patients significantly, 16 out of 30 patients were discharged, 10 out of 30 patients were died | [135] | |
Olokizumab | 160 mg/mL 0.4 mL was injected intravenously, one dose | - | Decreased CRP level significantly, improved clinical parameters | [136] | |
Anakinra | Anakinra was administrated subcutaneously at 100 mg/12 h from day (D) 1 to D3, then at 100 mg/24 h from D4 to D10 | 10 | Relieved fever in three days after the treatment of anakinra, improved respiratory function and inflammatory conditions, decreased the level of CRP and the level of 5/8 patients recovered to normal on day 11 from admission, the extension of lesions had stopped | [138] | |
100 mg three times a day or 200 mg intravenously twice a day | Case 1 was stopped to take it at the next due to improved respiratory functions, 7 days for case 2, and 10 days for case 3 | Improved respiratory functions, relieved fever, decreased the level of ferritin, improved pneumonia status and clinical conditions | [139] | ||
100 mg every 8 h (300 mg/daily) for 24 h to 48 h, followed by tapering, according to clinical response | 6–13 | All 5 patients experienced rapid resolution of systemic inflammation, and remarkable improvement in respiratory parameters, with the reduction of oxygen support requirement and early amelioration of chest CT scan abnormalities before discharge in 3 patients. All patients were discharged 6 to 13 days after the start of anakinra. No secondary infections or other adverse events were observed | [140] | ||
100 mg subcutaneously every 6 h | The maximum time is 19 days | Of the 14 patients who met the criteria, 11 patients received anakinra for a maximum of 19 days. Seven of the patients who started anakinra treatment ≤ 36 h after the onset of acute hypoxemic respiratory failure did not require mechanical ventilation, and all were discharged home. Four patients who started anakinra ≥ 4 days after the onset of acute hypoxemic respiratory failure required mechanical ventilation. Of those, 3 patients were extubated (2 discharged home and 1 remained hospitalized), and 1 died | [141] | ||
150 mg two times per day | 7 | Reduced the need for oxygen support, relieved fever, significantly reduced the level of ferritin and CRP with 48 h after the treatment of anakinra, improved respiratory functions and blood parameters after 7 days treatment of anakinra | [142] | ||
100 mg twice a day for 72 h, then 100 mg daily for 7 days | 10 | Need for invasive mechanical ventilation or death occurred in 13 (25%) of 52 patients in the anakinra group compared with 32 (73%) of 44 patients in the historical group. Among the 39 patients in the anakinra group, the need for oxygen was decreased; decreased the level of CRP; a higher rate of cases had an increase in liver aminotransferase (more than three times the upper limit of normal); a higher rate of cases developed a thromboembolic event during the hospital stay; seven (13%) had a pulmonary embolism, three (6%) had deep vein thrombosis of the lower limbs, and one (2%) had arterial thrombosis in the anakinra group; none of the patients in the anakinra group had a documented bacterial infection during the hospital stay | [143] | ||
Mavrilimumab | 6 mg/kg injected intravenously, one dose | - | Increased clinical improvement rate significantly, accelerated the time of clinical improvement and fever resolution | [144] | |
No significant difference in survival rate | [145] | ||||
C5a antibody | Eculizumab | 900 mg per time, two doses | - | Improved inflammatory status, all laboratory tests were better when the cases were discharged; decreased the level of CRP, improved CT manifestations | [146] |
900 mg per week | At most three weeks | Higher level of PaO2 and the ratio of PaO2/FiO2, decreased the level of D-dimer, increased platelet counts, improved CT manifestations, decreased the duration time of hospitalization | [147] | ||
900 mg per time or 1,200 mg per time | - | Significantly reduced the level of D-dimer and neutrophil counts; liver function and creatinine were normalized; severe heart failure and acute kidney injury had remissions; one case was died for respiratory failure | [148] | ||
Chemokine receptor 5 (CCR5) antibody | Leronlimab | 700 mg was injected subcutaneously, second dose was injected on Day 7 | - | IL-6 levels and inflammatory status were recovered; plasma viremia reduced | [149] |
700 mg was administered via two abdominal subcutaneous injections of 350 mg each, with repeat dosing if they remained hospitalized after 7 days | - | 17 out of 23 patients were recovered, and 4 out of 23 patients were died in 7 days. Reduced IL-6 level, CRP level was decreased dramatically after the second dose of leronlimab | [150] | ||
CD6 antibody | Itolizumab | 1.6 mg/kg totally | 14 | 7 out of 20 patients in the treatment arm did not survive and the 1-month mortality rate was 35%, compared to the 1-month mortality of 60% in the control arm (130 patients) | [152] |
Janus kinase (JAK) inhibitors | Ruxolitinib | 10 mg twice a day | 11 | Reduced the need for oxygen support, inhibited the proliferation of SARS-CoV-2 in blood, decreased the level of IL-6 and ferritin | [154] |
Started with 5 mg twice daily, the dosage was increased to 10 mg BID after 24–48 h in case there was no improvement of respiratory function and/or oxygen support from baseline, provided no adverse event ≥ grade 3 was observed; further escalation to 25 mg daily was allowed after an additional 48 h | 28 days, the median time is 13 days | 85.3% patients were discharged, 82.4% patients gained clinical improvement, 67.6% cases were PCR-negative on double check of upper respiratory tract swab at a median of 21 days after initiation of treatment with ruxolitinib, improved the frequencies of interferon (IFN)-γ producing T cells and TNF-α producing NK cells, improved inflammatory status, decreased the increased level of CRP induced by COVID-19Adverse effects: anemia, urinary tract infection, increase of creatinine, thrombocytopenia, increase of aminotransferases. Three thrombotic events were recorded: pulmonary embolism, brachial vein thrombosis following the positioning of the venous catheter, and peripheral arterial thrombosis | [155] | ||
Baricitinib | 4 mg/day | 2 weeks | Fever, SpO2, PaO2/FiO2, CRP, and Modified Early Warning Score (MEWS) significantly improved, all clinical characteristics and respiratory function parameters significantly improved and CRP values significantly decreased both at week 1 and week 2, increased the rate of cases discharged | [156] | |
Tofacitinib | 10 mg twice daily | 14 | Increased survival rate of COVID-19 patients significantly when compared to the treatment of dexamethasone only | [158] | |
BCR-ABL1 inhibitor | Imatinib | 400 mg once daily | 5 | Fever relieved; clinical symptoms improved and disappeared; blood tests turned out to be normal; pulmonary opacities had almost disappeared | [167] |
Bruton tyrosine kinase (BTK) inhibitors | Acalabrutinib | 100 mg orally or per enteric feeding tube twice daily | 10 days for patients on supplemental oxygen or 14 days for patients on mechanical ventilation | Increased SaO2, absolute lymphocyte count, and decreased CRP | [169] |
Ibrutinib | 420 mg/day | The median time was 52 months | No dyspnea was observed in 5 COVID-19 patients with Waldenström macroglobulinemia, they did not require hospitalization. Oxygenation improved, and CRP levels decreased were observed in one patient | [171] |
-: no accurate datum
Glucocorticoids
Glucocorticoids (GCs) are anti-inflammatory drugs, including hydrocortisone, methylprednisolone, dexamethasone, etc. They can inhibit inflammatory responses by suppressing the expression or transcription of related genes encoding pro-inflammatory cytokines [87–89]. It was reported that low doses of GCs were ever used for the treatment of severe pneumonia caused by influenza [90–92] and SARS [93–96].
A low dose of GCs can short ICU station and hospitalization of COVID-19 patients [97], and improve cough and chest CT results [98]. They also increase arterial oxygen tension rapidly [97, 99], and inhibit an inflammatory storm when patients progress to ARDS [99]. A family cluster of COVID-19 patients was recovered after short-term treatment with methylprednisolone [100]. A recovery trial showed that dexamethasone increased the survival rate of COVID-19 patients who received respiratory support [101] and that several cases were discharged from ICU after the treatment of dexamethasone [102]. Clinical parameters showed that compared to standard care alone, dexamethasone combined with standard care increased days of survival and ventilator-free in moderate or severe COVID-19 patients [103]. On the contrary, less literature reported that after 3-day administration of GCs, the patient’s symptoms become more severe in the following days [98]. Russell et al. [104] showed that GCs have no benefit in treating various viral infections. We speculate that their effects may depend on the timing of medication, i.e., the critical time point where cytokines burst and cell damage is imminent.
Statins
Statins are a class of drugs used to lower intracellular cholesterol synthesis by functioning as an HMG-CoA reductase inhibitor [105], including atorvastatin, rosuvastatin, lovastatin, simvastatin, fluvastatin, etc. The current studies proved that statins are not only safe but also protective against a severe COVID-19 infection with the reduction of mortality of COVID-19 patients through their known anti-inflammatory effect and binding capability that potentially stop the progression of the virus [106, 107]. Moreover, COVID-19 patients with prior treatment and continuous treatment of statins showed a favorable prognosis [108, 109], further suggesting that the anti-inflammatory treatment strategy is a preferred alternative in clinical practice.
Ivermectin
Ivermectin is an orally bioavailable macrocyclic lactone derived from Streptomyces avermitilis with antiparasitic and potential antiviral activities. Upon administration, ivermectin exerts its anthelmintic effect through binding and activating glutamate-gated chloride channels (GluCls) expressed on nematode neurons and pharyngeal muscle cells, which increases the permeability of chloride ions, inducing the state of hyperpolarization and resulting in the paralysis and death of the parasite [110]. Notably, the antiviral effect of ivermectin has been proved, which includes the activity against SARS-CoV-2 by binding to the importin α/β1 heterodimer that is responsible for the nuclear import of viral proteins such as integrase [111–114].
In the 363 mild patients (NCT04523831), ivermectin increased the early improvement rate (60.7% vs. 44.4%, P < 0.03) and reduced the clinical deterioration rate (8.7% vs. 17.8%, P < 0.02). Among the 280 hospitalized patients, the mortality rate was significantly reduced after ivermectin treatment (15.0% vs. 25.2%, P = 0.03). In the subgroup of patients with severe lung injury, the mortality rate after ivermectin treatment was also significantly reduced (38.8% vs. 80.7%, P = 0.001) [115]. Moreover, in some countries that implemented large-scale ivermectin administration programs to prevent parasitic infections, the number of COVID-19 cases had decreased significantly (P < 0.001) [116].
Immunomodulatory factor inhibitor
IL-6 antibody
Tocilizumab, a humanized monoclonal antibody against IL-6 receptor, is usually used to treat rheumatoid arthritis by inhibiting IL-6 signaling [117]. A retrospective analysis showed that tocilizumab is safe and sufficient for inhibiting cytokine storm induced by chimeric antigen receptor T (CAR-T) cell therapy [118]. Recently, emerging evidence demonstrated that tocilizumab can improve severe clinical signs, such as increased blood oxygen saturation, ameliorated chest CT image, and decreased body temperature, CRP, and lymphocytes [119–126]. Although there was evidence that tocilizumab did not affect COVID-19 [127, 128], it reduced the mortality and ICU hospitalization rate of COVID-19 patients [128]. However, the side effects of tocilizumab should not be ignored. The most common adverse effects of tocilizumab are anemia, elevated ALT, and QT interval prolongation [120]. It may also induce leukopenia in kidney transplant recipients [129] after tocilizumab treatment. Additionally, there were three patients who suffered candidemia after the treatment with tocilizumab [130].
Sarilumab is an antagonist antibody of IL-6R [131]. An open-label study conducted in Italy showed that sarilumab significantly facilitated the time of clinical improvement in patients with lung consolidation < 17% at baseline [132]. A retrospective study of 15 COVID-19 patients in Italy showed that the respiratory parameters of 67 percent (10/15) of patients were rapidly improved after sarilumab administration with improved inflammatory status, such as the decreased levels of IL-6 and D-dimer, and the reduced neutrophil to lymphocyte ratio [133]. For those patients with severe COVID-19, the survival rate was also significantly elevated after sarilumab administration [134].
Siltuximab is an antibody of IL-6 and targets the released cytokine. Clinical data from Italy showed that 33 percent (7/21) of COVID-19 patients were improved in clinical condition, and 43 percent (9/21) appeared stable, though the clinical situation worsened in 24 percent (5/21) of the patients. Another clinical study reported that there was a lower death ratio in 30 patients treated with 11 mg/kg siltuximab, compared to the 188 patients with supportive care, which may be due to the reduced levels of IL-8 and pentraxin 3 (PTX3) [135].
Olokizumab, another antibody of IL-6, is a humanized antibody that binds and neutralizes IL-6 selectively. The experience of the application of olokizumab to the treatment of COVID-19 could improve the parameters in clinical and experimental conditions [136].
IL-1 receptor antagonist
Anakinra is an IL-1 receptor antagonist and is usually used for rheumatoid arthritis [137]. It appears beneficial for the treatment of COVID-19 [138, 139]. A study showed that the treatment of 2 to 4 days with anakinra resolved systemic inflammation rapidly and improved respiratory function significantly, and all the patients were discharged 6 to 13 days after the treatment [140]. Another study also demonstrated that anakinra could improve the cytokine storm syndrome of COVID-19 patients, and 7 of 11 patients were discharged [141, 142]. Moreover, anakinra reduced both the need for invasive mechanical ventilation in ICU and the mortality in patients with severe forms of COVID-19 without serious side effects [143].
GM-CSFRα antibody
Mavrilimumab, a monoclonal antibody targeting GM-CSFRα, was used for treating rheumatoid arthritis to regulate the inflammatory response. Thirteen non-mechanically ventilated COVID-19 patients with hyperinflammation got significant improvement in clinical outcomes and their survival rates were increased after receiving mavrilimumab, compared to the control patients with standard care [144]. On the contrary, another report showed that mavrilimumab had no significant effects on increasing survival rate and reducing the rate of patients needing oxygen support [145].
C5a antibody
Eculizumab is an anti-C5a complement monoclonal antibody. When combined with anticoagulant and antiviral therapy, eculizumab reduced the inflammatory markers and CRP level [146], improved the respiratory symptoms, and decreased the D-dimer level [147, 148] of COVID-19 patients with severe pneumonia or ARDS. However, eculizumab could not completely save the critical COVID-19, as one patient died from respiratory failure and others suffered persistent renal failure [148].
Chemokine receptor 5 antibody
Leronlimab is a monoclonal antibody of chemokine receptor 5 (CCR5). When treated with leronlimab, ten COVID-19 patients had markedly reduced SARS-CoV-2 plasma viremia, though there was no placebo control in this study and four patients died due to disease complications and medical equipment limitation [149]. Another clinical trial showed that 17 out of 23 severe/critical COVID-19 patients recovered, and especially 4 out of 7 patients who were intubated before leronlimab administration were recovered without further oxygen support [150].
CD6 antibody
Itolizumab is a humanized recombinant monoclonal antibody that targets the extracellular domain of CD6. It has been approved for the treatment of psoriasis [151] and was also done by the Drug Controller General of India (DCGI) for the treatment of COVID-19. Clinical use of itolizumab revealed the increased survival rate of the twenty COVID-19 patients with acute respiratory syndrome [152].
Tyrosine kinase inhibitor
Janus kinase inhibitors
Janus kinase/signal transducer and activator of transcription (JAK/STAT) signaling pathway mediates cellular responses to cytokines (e.g., IL-6) and growth factors (e.g., EGF). JAKs were identified as a unique class of tyrosine kinases. Because of the causative role of JAKs in immune response, they have become attractive targets for COVID-19 treatment.
A meta-analysis showed that the use of JAK inhibitors, such as ruxolitinib, baricitinib, and tofacitinib, improved the clinical outcomes of COVID-19 patients [153]. According to clinical data, ruxolitinib, baricitinib, and tofacitinib all attenuated severe COVID-19 outcomes significantly, ameliorating the respiratory function, the blood oxygen level, and the discharged ratio of COVID-19 patients [154–159]. However, several adverse events occurred in the treatment with JAK inhibitors. For example, anemia and thrombocytopenia are the most frequent side effects, which may further increase the risk of COVID-19 [155, 160]. Early studies revealed that tofacitinib decreased the level of cytokines that regulate the development of lymphocytes, which would result in severe infections by bacteria and viruses [161–163].
BCR-ABL1 inhibitor
Imatinib is a BCR-ABL1 tyrosine kinase inhibitor which was usually used for chronic myeloid leukemia. It was demonstrated that imatinib was able to inhibit SARS and MERS-CoV infection in vitro [164–166], due to its ability to inhibit ABL2 which is required for SARS-CoV fusion with cell membrane [164, 165]. A clinical study showed that the clinical symptoms of COVID-19 patients were significantly improved and the patient was discharged five days after the treatment with imatinib [167], though in vitro study exhibited that it did not inhibit SARS-CoV-2.
Bruton tyrosine kinase inhibitor
Bruton tyrosine kinase (BTK), a tyrosine kinase expressed in cytoplasm, plays a critical role in regulating the signaling of B cells and innate immunity [168]. Acalabrutinib, a selective inhibitor of BTK, decreased the hospitalization rate of severe COVID-19 patients who need oxygen support and improved the inflammatory status [169]. Ibrutinib is a covalent inhibitor of BTK. In vitro study showed that ibrutinib could promote Th1 immunity and decrease the level of cytokines released from peripheral blood mononuclear cells of COVID-19 patients [170]. It was revealed that six COVID-19 patients’ Waldenström macroglobulinemia fully recovered after ibrutinib administration [171], and the discharge rate of the patients with severe COVID-19 was increased [172].
C-kit inhibitor
Masitinib, a member of the class of benzamides, is a highly selective oral c-kit tyrosine kinase inhibitor. Masitinib was approved for the treatment of mast cell tumors in dogs and evaluated in phase II and III clinical trials in humans for the treatment of cancer, asthma, Alzheimer’s disease, multiple sclerosis, and amyotrophic lateral sclerosis [173]. Recently, in silico screen and in vitro cell screen presented that masitinib inhibited SARS-CoV-2 infection (EC50 = 3.2 μM) through competitively binding with the catalytic residues of 3CLpro. Moreover, the treatment in mice showed that masitinib effectively reduced SARS-CoV-2 viral load (reducing > 99% of the viral load on day 6) and inflammatory signatures [174, 175].
Traditional Chinese Medicine
Traditional Chinese Medicine is medical crystallization in the long history of China and provides practical protection for the health of Chinese people. At a forum on COVID-19 diagnosis and treatment held by the Chinese Academy of Medical Sciences, academician Wang proposed COVID-19 as the cold-dampness pestilence [176]. Chinese medicine compounds have played an irreplaceable role in the defense and treatment of COVID-19, especially presenting the multi-component, multi-target, and multi-path mechanism of inhibition of virus replication and reducing the local inflammation leakage [177]. The third to eighth editions of Diagnosis and Treatment Protocol for Novel Coronavirus Pneumonia (DTPNCP), published by the National Health Commission of the People’s Republic of China, all recommended Chinese medicines to treat different stages of COVID-19 [178], and more and more Chinese medicines, including various dosage forms granule, capsule, and injection, were found to have the effects on the treatment of COVID-19 [179]. In Table 3, we listed the dosage, outcomes, and adverse effects of Traditional Chinese Medicine, and described them in detail below.
Efficiency of Chinese medicines
Chinese medicine | Dosage | Duration (day) | Outcomes | References |
---|---|---|---|---|
Lianhua Qingwen granules | 6 g/pack, one pack/time, thrice a day | 7 | The clinical symptoms of COVID-19 were improved significantly, laboratory tests showed significant improvement, the cases treated with Lianhua Qingwen granules had a higher improve rate of computed tomography (CT) manifestation, no adverse effects related to Lianhua Qingwen granules were noted | [185, 187, 188, 191] |
10 | The clinical symptoms of COVID-19 were improved significantly and reduced the rate of case deterioration, no severe adverse events related to Lianhua Qingwen granules were noted | [190] | ||
14 | The treatment with Lianhua Qingwen granules showed a significantly higher recovery rate and lower deterioration rate, accelerated the recovery time of clinical symptoms, improved CT manifestation significantly; the most common adverse event was the elevated alanine aminotransferase (ALT) levels or aspartate aminotransferase levels | [192] | ||
Jinhua Qinggan granules | 10 g/time, thrice a day | 5 | The clinical symptoms of COVID-19 were significantly improved and the rate of cases deteriorated was lowerAdverse effects: diarrhea | [196] |
6 g/time, twice a day | 7 | Accelerated the time of severe acute respiratory syndrome coronavirus 2 (SARS-CoV-2) shedding and pneumonia recovery, increased the clearance rate of SARS-CoV-2 | [197] | |
Qingfei Paidu decoction | One dose daily, thrice a day | 9 | Clinical symptoms and pneumonia were improved, the test of the nucleoid acid of SARS-CoV-2 showed negative | [199] |
One dose daily, twice a day | 9 | Clinical symptoms disappeared, CT manifestation improved, the test of the nucleoid acid of SARS-CoV-2 showed negative, the two cases were cured and discharged | [200] | |
Laboratory tests showed significant improvement. 41.13% cases were curedAdverse effects: 4 cases occurred nausea and vomit, 2 cases had dizziness, and 1 case had rash during the treatment | [201] | |||
3 doses a treatment course | Higher rate of cured cases, accelerated the improved time of clinical symptoms and CT manifestations, reduced the rate of cases deteriorated and adverse eventsAdverse effect: nausea | [202] | ||
Huopu Xialing decoction | One cell with 150 mL warm water a time, thrice a day | 6 | Clinical symptoms of COVID-19 disappeared, the repeat test for SARS-CoV-2 nucleoid acid showed negative, the case was discharged for isolation and observation | [204] |
Shuanghuanglian oral liquid | 20 mL per time, thrice a day | 9 | Clinical symptoms disappeared, CT manifestation images showed pneumonia improved | [206] |
Shufeng Jiedu capsule | 2.08 g/time, thrice a day | 10 | Accelerated the improved time of clinical symptoms and the time for nucleoid acid of SARS-CoV-2 turned negative significantly, increased the rate of cases cured and discharged | [211] |
14 | White blood cell count and the percentage of lymphocytes were increased significantly, the duration time of fever was shortened, and pneumonia was improved significantly with a higher total effective rateAdverse effects: 1 case appeared drug allergy, 2 cases appeared abdominal pain and diarrhea | [213] | ||
XiYanPing injection | 100 mg into liquid intravenously | 10 | Clinical symptoms were improved, blood oxygen saturation values were normalized, CT manifestations showed pneumonia were improved, 2 consecutive viral nucleoid acid tests showed negative, patients were discharged at last | [223] |
XueBiJing injection | The concentration is 500 mg/mL, 100 mL per time, twice a day | 7 | Improved pneumonia significantly, alleviated the increased level of inflammatory cytokines induced by COVID-19 | [214] |
Improved inflammatory status and pneumonia significantly, shortened the time virus nucleoid acid turned negative, decreased the number of severe patients significantly | [215, 217] | |||
ReDuNing injection | 20 mL intravenously in drip | 5–7 | Improved inflammatory status significantly, shortened the time of hospitalization and the duration time in ICU | [221] |
Lianhua Qingwen granule
Lianhua Qingwen granules are usually used to treat influenza-induced colds [180] through antiviral, anti-inflammatory, and immune modulation [181]. It is also commonly harnessed for patients with fever or high fever, chills, sore muscles, nasal congestion, runny nose, cough, headache, dry throat, sore throat, reddish tongue, and yellow greasiness. Lianhua Qingwen granules showed inhibitory activity on the replication of SARS-CoV and MERS in vitro [182–184]. Gene Ontology (GO) analysis and Kyoto Encyclopedia of Genes and Genomes (KEGG) pathway analysis showed that Lianhua Qingwen granules against COVID-19 might function through pathways like advanced glycation end product-receptor for advanced glycation end products (AGE-RAGE), TNF, and IL-7 signals [185]. The treatment of Lianhua Qingwen granules exerted higher disappearance rates of clinical symptoms like fever and cough [184, 186–190] and only a lower rate of cases turned severe [191]. In addition, 14-day treatment with Lianhua Qingwen granules increased the recovery rate of COVID-19 patients significantly, in which the recovery time was shorter than the control group [192]. Lianhua Qingwen granules combined with conventional therapy have been recommended by the Evidence-Based Medicine Chapter of China International Exchange and Promotive Association for Medical and Health Care for the treatment of patients with mild or moderate COVID-19 [193].
Jinhua Qinggan granules
Jinhua Qinggan granules are usually used for the treatment of fever and applied to colds induced by various influenza [194]. GO analysis showed that CASP3, ALB, IL-6, MAPK1, MAPK8, and MAPK14 might be the key molecules on which Jinhua Qinggan granules act for the treatment of COVID-19 [195]. Clinical studies showed that Jinhua Qinggan granules could improve the symptoms of COVID-19 patients and decrease the hospitalization rate [196]. This may be due to its ability to accelerate viral shedding in COVID-19 patients [197].
Qingfei Paidu decoction
Qingfei Paidu decoction is a Chinese medicine innovated from Zhang Zhongjing’s classical prescriptions. The National Health Commission and National Administration of Traditional Chinese Medicine recommended the use of Qingfei Paidu decoction for the treatment of COVID-19. Several core compounds of Qingfei Paidu decoction have an affinity to 3CLpro and ACE2 of SARS-CoV-2 [198], suggesting it is a possible treatment for COVID-19. Clinical use of Qingfei Paidu decoction showed that the symptoms of the patients with COVID-19, such as cough and shortness of breath, were significantly improved [199–202], and the nucleic acid test appeared negative [199, 200]. Another study showed that the total effective rate of Qingfei Paidu decoction combined with common anti-virus drugs for the treatment of COVID-19 reached 90% [200]. However, several patients had some adverse effects, such as skin rash, dizziness, nausea, and vomiting [199].
Huopu Xialing decoction
Huopu Xialing decoction is usually used for treating diseases with respiration, digestion, and endocrine systems and plays an important role in anti-inflammatory, anti-tumor, immune regulation, and metabolism improvement [177]. Regarding its mechanism, Huopu Xialing decoction might regulate the inflammatory response in COVID-19 patients [203]. The dialectical use of Huopu Xialing decoction significantly improved the symptoms of the patients with COVID-19, and SARS-CoV-2 RNA detection turned negative [204].
Shuanghuanglian oral liquid
Shuanghuanglian oral liquid is composed of honeysuckle, baicalin, and forsythia. It has the effects of clearing heat and detoxifying, and is usually used for colds, fever, coughs, and sore throat. Chinese Academy of Sciences demonstrated that Shuanghuanglian oral liquid had antiviral activity on COVID-19 on January 31, 2020 [205]. COVID-19 patients from a family recovered from the clinical use of Shuanghuanglian oral liquid without side effects [206].
Shufeng Jiedu capsule
Shufeng Jiedu capsule is usually used for acute upper respiratory tract infection which belongs to a wind-heat syndrome. Shufeng Jiedu capsule showed a definite curative effect on upper respiratory tract infections with no toxic and side effects [207–209]. It was known that Shufeng Jiedu capsule had broad-spectrum antiviral activities on H1N1, herpes simplex virus, RSV, parainfluenza virus, adenovirus, and coxsackievirus [210]. A retrospective study in Anhui province, China, showed that Shufeng Jiedu capsule enhanced the treatment effect of arbidol on COVID-19, shortened the detection time of SARS-CoV-2 RNA turning to negative, and increased the discharge rate [211]. With the treatment of Shufeng Jiedu capsule combined with LPV/r for 6–15 days, the symptoms of COVID-19 patients were obviously improved and the detection of SARS-CoV-2 appeared negative [212, 213]. Shufeng Jiedu capsule was recommended by the National Health Commission of the People’s Republic of China to treat COVID-19 patients under medical observation [54].
XueBiJing injection
XueBiJing injection is widely used in the clinical treatment of severe pneumonia, chronic obstructive pulmonary disease, ARDS, and other critical diseases. In vitro study showed that XueBiJing could improve the cell survival rate by inhibiting viral proliferation and balancing the pro-inflammatory cytokines that were deregulated by SARS-CoV-2 infection [214]. It might cure fever and improve CT imaging of COVID-19 patients [214, 215]. XueBiJing injection attenuated the severity index of critically ill patients with severe community-acquired pneumonia and reduced the mortality rate, compared to the placebo controls [216]. In addition, XueBiJing injection also increased white blood cell count and lymphocyte count, and decreased a CRP level in COVID-19 patients 7 days after the treatment. However, there was no significant difference in the negative rate of SARS-CoV-2 nucleic acid test between the routine treatment group and the XueBiJing treatment group [217].
ReDuNing injection
ReDuNing injection is used for colds, coughs, and upper respiratory tract infections [218]. It has antiviral activity on viruses H1N1, H5N1, EV71, and CVB3 [219]. Network pharmacology analysis showed that ReDuNing injection could be used for the treatment of COVID-19 through the activity of anti-inflammation and immunomodulation [220]. Clinical data demonstrated that ReDuNing injection combined with methylprednisolone could reduce the inflammatory cytokines and shorten the length of stay in the hospital ICU, compared to the treatment of methylprednisolone alone [221].
XiYanPing injection
The key effective component of XiYanPing injection is the total sulfonate of andrographolide, which acts through anti-inflammation and has a certain effect on viral pneumonia and upper respiratory tract infection [222]. Clinical data showed that three critical COVID-19 patients were cured after the treatment of XiYanPing injection combined with other Chinese medicines and Western medicines [223].
Although positive effects of Chinese medicine have been shown for treating COVID-19, some adverse events may occur in the treatment of several Chinese medicinal formulae. For example, ALT levels or aspartate aminotransferase levels were elevated in partial patients treated with Lianhua Qingwen granules [192]. Several patients suffered diarrhea after the treatment of Jinhua Qinggan granules [196]. Qingfei Paidu decoction caused nausea and vomiting [201, 202]. One case had drug allergy and two cases suffered abdominal pain and diarrhea after treated with Shufeng Jiedu capsule [213]. However, the mechanisms by which these adverse events occur are still unknown. This is the reason why we tried to predict potential targets of the Chinese medicinal formulae for COVID-19.
In order to explore targets of Traditional Chinese Medicine for COVID-19, we searched from “GeneCards” and “The Human Gene Database” online software with the keywords “new coronavirus”, and obtained 791 targets related to COVID-19. Then, the therapeutic targets of Traditional Chinese Medicine were analyzed. Since nine Chinese medicine formulae could be used to treat COVID-19, we wanted to know the potential mechanisms of these nine Chinese medicine formulae for the treatment of COVID-19. The relevant literatures on the treatment of COVID-19 with the Traditional Chinese Medicine were searched for from China National Knowledge Infrastructure (CNKI) and National Center for Biotechnology Information (NCBI), compared to the efficacy of nine Chinese medicine formulae. Five Chinese medicine formulae with better efficacy were selected for subsequent analysis (Figure 1a). First, we calculated the appearance frequency of each herb in the five Chinese medicine formulae (Table 3). The results showed that five Chinese medicine formulae contain 42 herbs, of which 10 herbs appear in at least two Chinese medicine formulae (Figure 1b). The most frequently used component is Radix Glycyrrhizae, which appears in four Chinese medicine formulae, followed by Semen Armeniacae Amarum, Herba Ephedra sinica, and Fructus Forsythiae Suspensae, which appear in three Chinese medicine formulae, and Flos Lonicerae, Mentha haplocalyx, Radix Scutellaria baicalensis, Herba Agastache rugosa, Radix Isatis indigotica, and Radix Bupleuri Chinensis appear in two Chinese medicine formulae. Recently, it was shown that two extracts of Herba Ephedra sinica could block the interaction between ACE2 and the S-receptor-binding domain (RBD) of four SARS-CoV-2 phenotypes [224]. Then, we searched for ingredients and potential targets in these 10 herbs through the Traditional Chinese Medicine Systems Pharmacology Database and Analysis Platform (TCMSP), which is a systematic pharmacology platform that links drugs, targets, and diseases into the network for analysis, found that these 10 herbs contain 297 targets, and compared these targets with the targets related to COVID-19 through the GeneCards database, 35 common targets are obtained (Figure 1b). These common targets might be the targets of 10 herbs for the treatment of COVID-19. In order to more accurately analyze the targets of five prescriptions for COVID-19 treatment, we analyzed the common ingredients in 10 common herbs (Figure 1c) and collected 232 targets of the common ingredients. 33 of them are the same targets as COVID-19. Compared with the previous 35 COVID-19 targets obtained from the 10 common herbs, STAT3 and CDK2 were missing. Finally, the ingredients contained in each of the five Chinese medicine formulae were analyzed to find that there are 25 ingredients appearing in at least two of the five Chinese medicine formulae. We got 239 targets from these 25 ingredients, of which 33 targets are the same as COVID-19 related targets. Compared with the previous 35 COVID-19 targets obtained from the 10 common herbs, DPP4 and MAPK14 are missing (Figure 1d). Therefore, the potential targets of Traditional Chinese Medicine for the treatment of COVID-19 are the 35 COVID-19 targets from the 10 common single medicines. Among them, STAT3, CDK2, DPP4, and MAPK14 do not play a major role in the treatment of COVID-19 (Figure 1e).
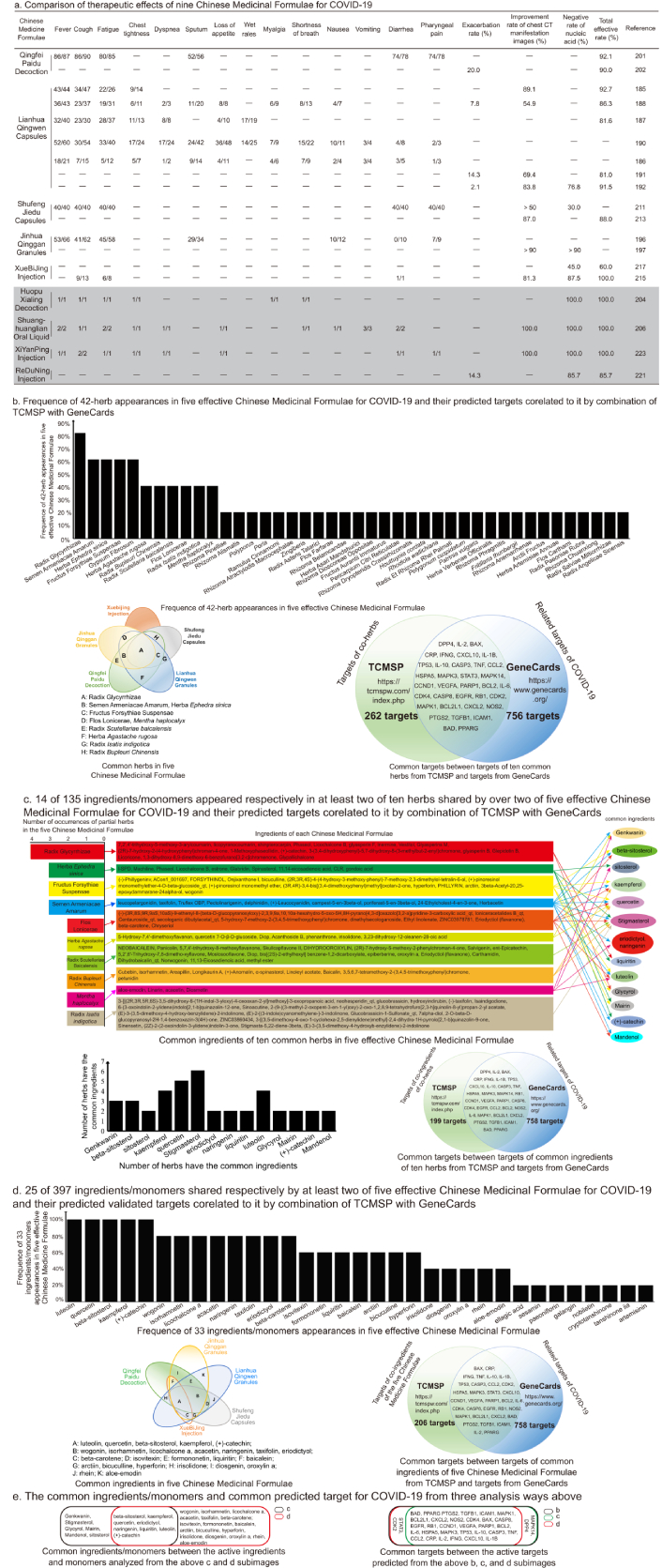
Therapeutic effects of nine Chinese medicine formulae on COVID-19 and predicted targets of their shared traditional herbs and ingredients/monomers. a. The numerator represents the disappeared number of cases with various symptoms after treatment, and the denominator represents the existing number of cases with various symptoms before treatment; b. five crossed color circles show the herbs in each Chinese medicine and the ones shared by two or more Chinese medicines, where each color circle represents one Chinese medicine. Each letter in the circle cross represents the herbs shared by the five Chinese medicines. Among these five Chinese medicines, no herb in XueBiJing injection is shared with the other four Chinese medicines. The herbs represented by letters are listed under the color circles. The histogram shows the appearance frequency of each herb in the five Chinese medicines. The two crossed color circles display the related targets of the common herbs for the treatment of COVID-19. The green circle represents the 297 targets of ten herbs that were obtained through analyzing the Traditional Chinese Medicine Systems Pharmacology Database and Analysis Platform (TCMSP). The blue circle represents the 791 targets related to COVID-19 that were obtained through analyzing GeneCards with the keywords “novel coronavirus”. The molecules in the cross of two circles are the targets of the herbs for the treatment of COVID-19. The molecules in the cross of the two color circles are the same target ones of both the herbs and COVID-19, which means that these molecules may be the targets of the common herbs for the treatment of COVID-19; c. the color squares in the left column represent the herbs listed in Figure 1b. The ingredients of each herb are in the squares in the middle column with the same color as the left. The right columns exhibit the common ingredients and the ingredients shared by two or more herbs. The ingredients pointed by each color arrow belong to the herbs with the same color. The two crossed color circles show the related targets of the common ingredients shared by two or more herbs for the treatment of COVID-19. The green circle represents the 232 targets of ten herbs that were obtained through analyzing TCMSP. The blue circle represents the 791 targets related to COVID-19 that were obtained through analyzing GeneCards with the keywords “novel coronavirus”. The molecules in the cross of two circles are the targets of the herbs for the treatment of COVID-19. The molecules in the cross of the two circles are the same target ones of both the ingredients and COVID-19, which means that these molecules may be the targets of the common ingredients of the ten herbs for the treatment of COVID-19; d. five crossed color circles show the ingredients containing validated targets in each Chinese medicine and the ingredients shared by two or more Chinese medicines. Each color circle represents one Chinese medicine. The letters in the cross of circles indicate that there are two or more Chinese medicines sharing the ingredients. The ingredients represented by each letter are listed under the color circles. The histogram shows the appearance frequency of each ingredient containing validated targets in the five Chinese medicines. The two crossed color circles show the related targets of the common ingredients for the treatment of COVID-19. The green circle represents the 239 targets of the common ingredients that were obtained through analyzing TCMSP. The blue circle represents the 791 targets related to COVID-19 that were obtained through analyzing GeneCards with the keywords “novel coronavirus”. The molecules in the cross of two circles are the targets of the herbs for the treatment of COVID-19. The molecules in the cross of the two circles are the same target ones of both the common ingredients and COVID-19, which means that these molecules may be the targets of the common ingredients containing the validated targets for the treatment of COVID-19; e. the common ingredients/monomers and common predicted targets for COVID-19 were analyzed from the above b, c, and d subimages. The left crossed rectangles indicate the common ingredients/monomers between common ingredients/monomers of ten herbs and common ingredients/monomers of five Chinese medicinal formulae. The black rectangle represents the common ingredients/monomers of ten herbs, and the red rectangle represents the common ingredients/monomers of five Chinese medicinal formulae. The right crossed rectangles indicate the common targets between the common target molecules predicted from the above b, c, and d subimages. The black rectangle represents the common targets of the targets of ten common herbs from TCMSP and the targets from GeneCards predicted from the b image. The green rectangle represents the common targets of common ingredients of ten herbs from TCMSP and the targets from GeneCards predicted from the c image. The red rectangle represents the common targets of targets of common ingredients of five Chinese medicinal formulae from TCMSP and the targets from GeneCards predicted from the d image. CT: computed tomography
S protein-targeted therapy and vaccine
Convalescent plasma
Since there is no proven drug therapy, monoclonal neutralizing antibody (nAb), or effective vaccine to treat or prevent COVID-19, convalescent plasma therapy fully exhibits its advantages of rapid application in COVID-19 treatment. Convalescent plasma therapy has been listed in the DTPNCP since trial version 4 [225]. China National Biotec Group first successfully prepared anti-SARS-CoV-2 positive plasma for a clinical trial for the treatment of critically ill patients on January 20, 2020. Ten patients of COVID-19 received 200 mL convalescent plasma (1:640) transfusion, respectively. After treatment, the concentration of the antibody in the plasma of five patients increased rapidly to 1:640, and the viral load in seven patients with viremia decreased to an undetectable level. The absorption of pulmonary lesions was improved within 7 days after the convalescent plasma treatment, many auxiliary detection indexes were improved and no obvious adverse reactions were observed [226]. Liu et al. [227] used a propensity score-matched control study to observe the treatment effect of thirty-nine patients with severe or life-threatening COVID-19 after receiving the convalescent plasma therapy and found that the survival rates of convalescent plasma recipients were improved. However, Li et al. [228] confirmed that compared with standard treatment alone, standard treatment combined with recovery plasma did not significantly improve the clinical condition in patients with critical COVID-19 within 28 days. More evidence shows that patients gain the most obvious effects produced by convalescent plasma therapy before the numbers of symptoms appear because the patients do not produce high levels of antibodies at this time [227]. Furthermore, the plasma therapy also has risks such as allergic reactions. Thus, more expanded clinical trials remain to be fulfilled. Bloch et al. [229] compiled a guideline on how to use the convalescent plasma of COVID-19 survivors to improve the treatment capabilities of medical institutions and outlined the criteria for qualified plasma donors, the risks and potential benefits of the therapy, and how the hospital mobilizes donors and cooperate with local and national blood centers.
Monoclonal nAb
Convalescent serum therapy is an important method for the treatment of COVID-19 patients, which actually contains the nAb produced by the human body’s immune response to pathogenic microorganisms. However, the main disadvantage of this therapy is that the source is limited, it is difficult to control quality, and the titer of nAb is low. Therefore, the research and development of monoclonal neutralizing antibodies that are easy to produce and to be quality-controlled may be a potential “specific effective drug” for the treatment of COVID-19. This has been fully demonstrated in the development of nAbs against the Ebola virus, such as mAb114 and REGN-EB3 (cocktail therapy of 3 monoclonal antibodies) that can reduce mortality from 67% to 11% and 6% [230, 231].
The target of the monoclonal nAb is basically SARS-CoV-2’s Spike protein that binds to the human receptor ACE2 with RBD domain (Figure 2a and b) [232, 233]. Wu et al. [234] isolated two human monoclonal antibodies (B38 and H4), which can prevent the binding of the coronavirus S protein RBD to the human cell receptor ACE2. Xie et al. [203] successfully identified 14 highly active nAbs from sixty convalescent patients, of which the antibody numbered BD-368-2 performed outstandingly. It was also confirmed that the treatment of hACE2 transgenic mice infected with SARS-CoV-2 with BD-368-2 reduced the viral load by 2,400 times, and the injection of BD-368-2 could completely inhibit the viral infection in the mouse model, indicating that the selected nAbs have both therapeutic and short-term preventive applications [235]. There are other nAbs targeting RBD, such as nAbs BD23, CV30, EY6A, VHH-72, and S309 [235–239]. Although RBD is the core target for nAbs against SARS-CoV-2, non-RBD-targeted nAbs were also reported and the patients may benefit from the strategy of nAbs cocktail therapeutics. Other nAbs were reported to target the N-terminal domain (NTD) of Spike protein, such as nAbs 4A8, COV57, 2-17, and 5-24 [240, 241]. Specially, nAb 4A8 discovered by Chi et al. [240], is the first highly effective monoclonal nAb targeting the NTD. To date, many nAbs showing promising therapeutic potential have been evaluated clinically. The binding sites that interacted with S protein of the nAbs mentioned above are listed in Table 4.
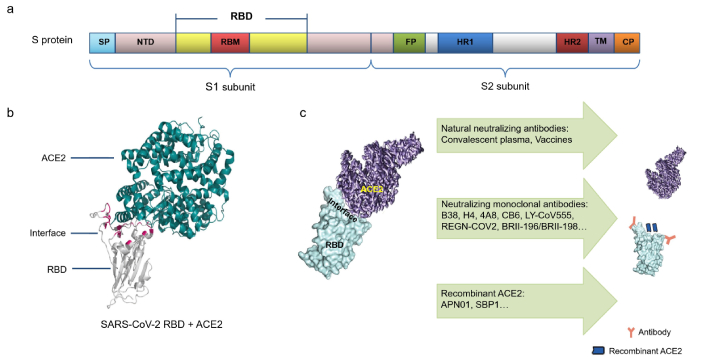
S protein-targeted therapies and vaccines. a. Schematic of the S protein; b. the crystal structures of the RBD (the core structure is gray and the loop RBM appears pink) and the receptor ACE2 (dark green) form a complex; c. pattern of dissociating RBD/ACE2 complex. Upper lane: Convalescent plasma contains or vaccines produce natural neutralizing antibodies; middle lane: monoclonal neutralizing antibodies; lower lane: recombinant ACE2 blocking ACE2-RBD binding (interface is marked blue) [242]. SP: signal peptide; NTD: N-terminal domain; RBD: receptor-binding domain; RBM: receptor binding motif; FP: fusion peptide; HR1: heptad repeat region 1; TM: transmembrane region; CP: cytoplasmic tail; ACE2: angiotensin-converting enzyme 2; SARS-CoV-2: severe acute respiratory syndrome coronavirus 2
Note. Figure 2 is adapted from “Receptor-binding domain-specific human neutralizing monoclonal antibodies against SARS-CoV and SARS-CoV-2” by Yu F, Xiang R, Deng X, Wang L, Yu Z, Tian S, et al. Signal Transduct Target Ther. 2020;5:212 (https://doi.org/10.1038/s41392-020-00318-0). CC BY.
Binding sites of neutralizing antibody or recombinant ACE2 in S protein
S protein-targeted agents | Name | Binding sites in S protein | References |
---|---|---|---|
Neutralizing antibody | B38 | RBD: R403, D405-E406, Q409, T415-K417, D420-Y421, Y452, L454-N460, Y473-S477, F486-N487, Y489-F490, Q493-G496, Q498, T500-V503, Y505 | [231] |
H4 | RBD | [231] | |
BD-368-2 | RBD | [232] | |
BD-23 | RBD: G446, Y449, L452, T470, E484-F486, Y489-F490, L492-S494, G496, Q498, T500-N501, Y505 | [232] | |
CV30 | RBD: R403, T415-K417, D420-Y421, Y453, L455-N460, Y473-S477, F486-N487, Y489, Q493, T500, G502, Y505 | [233] | |
EY6A | RBD: Y369, F374-S375, F377-K386, N388, L390, P412-G413, D427-F429, L517 | [234] | |
VHH-72 | RBD: Y356-T359, F361-C366, A371-T372, G391-D392, R395, N424, I489, Y494 | [235] | |
S309 | RBD: T333-L335, P337, G339-V341, N343-T345, K356-C361 | [236] | |
4A8 | NTD: R246, K150, K147, H146, W153, Y145, N149 | [237] | |
COV57 | NTD | [238] | |
2-17 | NTD | [238] | |
5-24 | NTD | [238] | |
LY-CoV016 | RBD: R403, D405-E406, R408-Q409, T415-K417, D420-Y421, L455-N460, Y473-S477, F486-N487, Y489, Q493, Y495, N501-G502, G504-Y505 | [230] | |
LY-CoV555 | RBD: TYR32, ARG50, LEU55, TYR92, ARG96, TYR101, GLU102, ARG104, LEU452, GLU484, PHE486, PHE490, GLN493, SER494 | [239] | |
REGN10933 | RBD: R403, K417, Y421, Y453, L455-F456, A475-G476, E484-Y489, Q493 | [240] | |
REGN10987 | RBD: R346, N439-L441, K443-N450, Q498, T500 | [240] | |
BRII-196 | RBD | [230] | |
BRII-198 | RBD | [230] | |
Recombinant ACE2 | APN01 | RBD | [243] |
SBP1 | RBD | [245] |
ACE2: angiotensin-converting enzyme 2; RBD: receptor-binding domain; NTD: N-terminal domain
Etesevimab/bamlanivimab
Etesevimab/bamlanivimab cocktail under development had received EUA from the FDA for mild to moderate COVID-19 patients. Etesevimab (LY-CoV016) is an RBD-directed antibody jointly developed by Junshi Biosciences and the Institute of Microbiology, Chinese Academy of Sciences (IMCAS) [233]. Bamlanivimab (LY-CoV555) is the first antibody to enter phase III clinical trials to verify whether it can effectively prevent SARS-CoV-2 infection (NCT04497987) [242]. In a clinical trial involving 1,035 patients with mild to moderate COVID-19, there existed a 70% reduction in risk. Eleven patients in the treatment group were hospitalized or died, but thirty-six ones in the placebo group were hospitalized or died (P = 0.0004). In another clinical trial, there were also 10 deaths in the placebo group, but no deaths in the treatment group (NCT04427501).
REGN-COV2
A combination of REGN10987 and REGN10933 antibodies is being used to form the REGN-COV2 cocktail against COVID-19, which also obtained EUA from FDA. The REGN10933 binding site extensively overlaps the receptor binding motif (RBM) and precludes hACE2 binding, while REGN10987 binds away from the RBD and has little to overlap with the hACE2 binding site [243]. In phase III clinical trials (REGN-COV2069), subcutaneous injection of REGN-COV2 could reduce the risk of symptomatic infection in uninfected individuals by 81%. Moreover, in 204 asymptomatic infections randomized to receive subcutaneous injections of REGN-COV2 or placebo, the symptomatic infections in the treatment group were reduced by 31%. The data from this trial confirmed the potential dual value of REGN-COV2 in reducing SARS-CoV-2 infection and the burden of COVID-19.
BRII-196/BRII-198
In China, BRII-196/BRII-198 was officially approved by the National Medical Products Administration (NMPA) for the treatment of patients with mild to moderate COVID-19. P2C-1F11 is the precursor of BRII-196 and has neutralizing activity against delta mutant strains and other varieties [233–244]. The half-life of BRII-196/BRII-198 is 2–3 times of ordinary antibodies, extending from 21 days to 46–76 days [245]. A phase 2/3 clinical study (NCT04518410) showed that the risk of hospitalization and death in the BRII-196/BRII-198 treatment group was reduced by 80% (P < 0.00001). There was no death in the treatment group but nine patients died in the placebo group during the 28-day treatment period.
Recombinant ACE2
Similar to monoclonal nAb against S protein, the mechanism of exogenous ACE2 or ACE2 fragment is to disrupt coronavirus to invade host cells through S protein-ACE2 interaction. Monteil et al. [246] revealed that a clinical-grade human recombinant soluble ACE2 (hrsACE2), named APN01, could reduce viral growth by a factor of 1,000–5,000 through binding to RBD in in vitro cell-culture experiments and the engineered human blood vessel and kidney organoids at the early stage of infection (Table 4). Moreover, APN01 was also used to treat one patient with critical COVID-19, which showed that the clinical manifestation was improved gradually without obvious drug-related side effects, and might prevent cytokine storm [247]. Zhang et al. [248] chemically synthesized a 23-mer peptide fragment of the ACE2 (SBP1) and proved SBP1 binding to recombinant SARS-CoV-2-RBD (Table 4), indicating that SBP1 has the potential to inhibit the virus entering human cells by disrupting the SARS-CoV-2-RBD binding to ACE2. APN01 and SBP1 had the function of dissociating RBD/ACE2 complex, which is similar to convalescent plasma, monoclonal neutralizing antibodies, and vaccines, and might be a promising strategy for COVID-19 (Figure 2c).
Vaccines
Vaccine is a top priority in the follow-up fight against the epidemic. According to the latest statistics from WHO as of December 19, 2021, a total of 8,387,658,165 vaccine doses have been administered [249]. Zhu et al. [250] released the world’s first COVID-19 vaccine human clinical data. It was conducted in 108 healthy adults and no serious adverse events were found within 28 days after vaccination. Neutralizing antibodies increased significantly on the 14th day and reached the peak on the 28th day after inoculation (humoral immunity), and the specific T cell response reached the peak on the 14th day after inoculation (cellular immunity). Different vaccine platforms such as inactivated virus, recombinant protein subunit, adenovirus, and nucleic acid vaccine are under development. Among them, the development of mRNA vaccine is making rapid progress, and it has more advantages in safety than traditional peptides and DNA vaccines. Jackson et al. [251] developed an mRNA vaccine against SARS-CoV-2, which is called mRNA-1273. The phase I trial of mRNA-1273 showed that the nAb was observed in 100% of the subjects, and the geometric mean titer (GMT) of the nAb in the 100 μg dose group was higher than that in the serum of recovered patients. The phase III trial demonstrated that the overall protection rate of mRNA-1273 had reached 94.1% [252]. Polack et al. [253] surveyed 21,720 participants who received injections with mRNA vaccine BNT162b2 and demonstrated that a two-dose regimen of BNT162b2 conferred 95% protection against COVID-19 in persons aged 16 years or older. WHO summarized the latest information on COVID-19 vaccine candidates in clinical and pre-clinical development, and some of these vaccines entered phase III clinical trials and have been widely used for population vaccination prevention, such as CoronaVac, mRNA-1273, BNT162b2, AZD1222 [254].
However, Wang et al. [255] followed up with thirty patients with COVID-19 (the observation period is more than 3 months) and confirmed that the nAb titer of COVID-19 patients during the recovery period would decrease significantly over time, suggesting that SARS-CoV-2 may not bring about a single time lifelong immunity for its infection. The above studies may provide references for formulating long-term prevention and control for SARS-CoV-2 and vaccine immunization strategies.
Others
Vitamin C, a well known antioxidant vitamin, can protect cells and tissues by scavenging damaged reactive oxygen species. It also maintains the healthy immune function of human beings. Vitamin C is decreased in infected people and the requirement of vitamin C depends on how severe the infection is [256], which makes it possible that vitamin C has benefits for the treatment of COVID-19. It was found that there is a low vitamin C level in the serum of COVID-19 patients [257, 258]. Administration of vitamin C could decrease the D-dimer and ferritin levels in COVID-19 patients, but it also showed 12% mortality with 17.6% cases that received respiratory support [259]. However, excessive administration of vitamin C may induce oxalate nephropathy in COVID-19 patients [260].
Vitamin D can promote calcium absorption [261], which has been shown to reduce the risk of influenza infection by reducing the virus replication rate and the levels of pro-inflammatory cytokines [262]. Vitamin D could reduce the risk of viral infections and death through many mechanisms, one of which is that vitamin D can induce the expressions of antimicrobial peptides like cathelicidin [263] and 1,25-dihdroxyvitamin D [264] that have antiviral activity. Ecological research showed that serum 25(OH)D concentration may reduce the risk induced by influenza [262]. Its concentration was shown negatively related to cytokine storm [265], as the higher serum 25(OH)D concentration reduces the incidence of cytokine storm, suggesting the possibility of the treatment of COVID-19. Clinical evidence showed that the patients with COVID-19 had lower serum 25(OH)D concentrations [266]. Analysis of the relationship between the COVID-19 incidence and serum 25(OH)D concentration showed that the regional people with lower serum 25(OH)D concentration have higher COVID-19 incidence [267–269], suggesting that keeping a relatively high serum 25(OH)D concentration may be helpful for preventing and treating COVID-19.
Moxifloxacin is a fluoroquinolone and has a broad-spectrum antibacterial activity on aerobic gram-negative and gram-positive bacteria. Moxifloxacin could inhibit bacterial DNA gyrase and topoisomerase IV, which are required for the synthesis of bacterial mRNAs (transcription) and DNA replication [270]. Moxifloxacin administration may protect virus-infected patients whose immunity is reduced from bacterium infection. It has been approved by the United States for the treatment of mild-to-moderate bacterial infections like bronchitis and community-acquired pneumonia [271].
Other antibacterial drugs, like ceftriaxone and azithromycin, were also used for antibacterial therapy of COVID-19 [2]. Azithromycin is a broad-spectrum antibacterial drug. A study demonstrated that azithromycin could inhibit SARS-CoV-2 in vitro with EC50 = 2.12 μM [272], while another study showed that it had no inhibitory effect on SARS-CoV-2, but inhibited SARS-CoV-2 replication in vitro when combined with hydroxychloroquine [273], and SARS-CoV-2 RNA detection turned negative rapidly after the patients administrated with azithromycin combined with hydroxychloroquine [274].
Conclusions
COVID-19 infection has developed into a global public health event. Patients with COVID-19 suffer the decreased lymphocytes and even repeated infections. We should prepare to co-exist with SARS-CoV-2 for a long time. To date, therapeutic strategies for it mainly focus on antiviral, anti-inflammation, symptomatic treatment, neutralizing antibodies, immune conditioning, Vitamin D, vaccine, and so on (Figure 3). Antiviral therapy is to inhibit viral replication. The key enzymes required for viral replication include RdRp, 3CLpro, and PLpro, which have become the targets for searching drugs against COVID-19. Remdesivir, molnupiravir, ribavirin, LPV/r, PF-07321332, and Type I IFN, all have antiviral activities on COVID-19 by inhibiting viral replication in cells through different mechanisms. Compared to other Western medicines for antiviral treatment of COVID-19, sofosbuvir/daclatasvir and LPV/r may be a better choice as the both show fewer adverse events though their effectiveness and duration time have no significant difference.
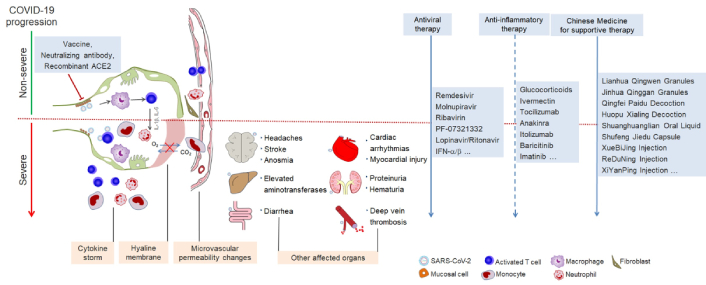
Summary of the application of therapeutic strategies against COVID-19. COVID-19 progression, upper: non-severe (green line); lower: severe (red line). Antiviral therapy recommended to treat COVID-19 throughout the procession (solid line); anti-inflammatory therapy recommended to treat COVID-19 at different procession (dotted line), dependent on drugs; Chinese medicine recommended to treat COVID-19 throughout the procession (solid line). ACE2: angiotensin-converting enzyme 2; SARS-CoV-2: severe acute respiratory syndrome coronavirus 2; IFN: interferon
Rationally, anti-inflammatory therapy is also indispensable for the treatment of COVID-19 as elevated pro-inflammatory cytokines and cytokine storms can occur in some COVID-19 patients. GCs display efficacious anti-inflammatory effect on patients. However, the commonality of these chemical drugs is their greater cytotoxicity, and long-term administration of GCs might result in extra transmission of SARS-CoV-2. Compared to other Western medicines for the anti-inflammatory treatment of COVID-19, anakinra and statins appear greater advantageous in the improved inflammatory status and respiratory function, and in the reduced mortality rate.
In particular, it has been revealed that Chinese medicinal herbs and their formulae are also effective means against COVID-19, especially in the case of Lianhua Qingwen granules and Qingfei Paidu decoction. Actually, the Chinese medicines usually used in the treatment of influenza infection and respiratory-related diseases, including their granules, capsules, decoctions, and injections, all have effects on COVID-19 to different extends. They are mild and less toxic, and exhibit a unique impact on inhibiting SARS-CoV-2, mitigating cytokine storm, synergizing innate and adaptive immune responses, and maneuvering the functional state of the whole organism. Compared to other Traditional Chinese Medicine formulae for the treatment of COVID-19, Qingfei Paidu decoration and Lianhua Qingwen capsules present higher effective rates. Compared further to other drug dosage forms, the injections of these Chinese medicinal formulae seem to be of a higher effective rate. However, Chinese medicine is a combination of different herbs and its key molecules still need to be further explored. More stringent treatment protocols for Traditional Chinese Medicine remain to be developed and refined for ultimately realizing its standardization and normalization. Considering that Western medicine and Chinese medicine have different advantages and shortages in the process of treating COVID-19, their combination usage for the treatment of COVID-19 may be a better option than monotherapy.
Additionally, the screening of active ingredients, monomers, and their potential targets for COVID-19 in different Traditional Chinese Medicine formulations can be obtained and determined through the analysis of the TCMSP database combined with the GeneCards database in the current review. At present, other pharmacological approaches or platforms are emerging to calculate and screen potential therapeutic drugs by identifying drug targets and disease-related targets, such as the ingredients-based pharmacotranscriptomic platform integrated Traditional Chinese Medicine (ITCM) [275] and the contribution of the immune microenvironment and metabolic reprogramming (COIMMR) [276]. The Traditional Chinese Medicine integrated databases (TCMID) (http://www.megabionet.org/tcmid/), the TCM Database@Taiwan (http://tcm.cmu.edu.tw/), and TCMBank (https://tcmbank.cn) may also be employed for drug target analysis [185]. The online mendelian inheritance in man (OMIM) database and the universal protein resource (UniProt) database may be used for screening COVID-19 targets as well [185, 198, 220]. Furthermore, a new computational and analytic platform for COVID-19, Encyclopedia of Traditional Chinese Medicine (ETCM), has been developed [277]. However, these pharmacology approaches for drug screening need further in vitro and in vivo experiments to verify the effectiveness of drugs or targets.
Overall, COVID-19 may turn into a chronic, long-term disease like influenza. Vaccines are key to solving the global epidemic. According to a document released by the WHO on July 31st, 2021, there are 26 experimental COVID-19 vaccine projects currently undergoing clinical trials. As a supplement to the vaccine, perhaps convalescent serum therapy, monoclonal neutralizing antibodies, and immune cell therapy will become effective methods for the treatment of COVID-19.
Abbreviations
3CLpro: | 3 chymotrypsin-like cysteine protease |
ACE2: | angiotensin-converting enzyme 2 |
ALT: | alanine aminotransferase |
ARDS: | acute respiratory distress syndrome |
BTK: | Bruton tyrosine kinase |
CRP: | C-reactive protein |
CT: | computed tomography |
EUA: | emergency use authorization |
FDA: | Food and Drug Administration |
GCs: | glucocorticoids |
HCV: | hepatitis C virus |
IFNs: | interferons |
JAK: | Janus kinase |
LPV/r: | lopinavir/ritonavir |
nAb: | neutralizing antibody |
RBD: | receptor-binding domain |
RdRps: | RNA-dependent RNA polymerases |
SARS-CoV-2: | severe acute respiratory syndrome coronavirus 2 |
STAT: | signal transducer and activator of transcription |
TCMSP: | Traditional Chinese Medicine Systems Pharmacology Database and Analysis Platform |
WHO: | World Health Organization |
Declarations
Author contributions
FX: Conceptualization, Writing—original draft, Visualization, Writing—review & editing, Funding acquisition. YX: Writing—original draft, Visualization, Writing—review & editing. YL: Writing—original draft, Visualization.
Conflicts of interest
The authors declare no conflicts of interest.
Ethical approval
Not applicable.
Consent to participate
Not applicable.
Consent to publication
Not applicable.
Availability of data and materials
Not applicable.
Funding
This work was supported by Guangzhou Science and Technology Program Synergistic Innovation Major Project [No.: 201604020146] to FX. The funders had no role in study design, data collection and analysis, decision to publish, or preparation of the manuscript.
Copyright
© The Author(s) 2025.
Publisher’s note
Open Exploration maintains a neutral stance on jurisdictional claims in published institutional affiliations and maps. All opinions expressed in this article are the personal views of the author(s) and do not represent the stance of the editorial team or the publisher.