Abstract
The severe acute respiratory syndrome coronavirus-2 (SARS-CoV-2), known to cause the coronavirus disease 2019 (COVID-19), was declared a pandemic in early 2020. During the past time, several infections control methods have been developed. Nevertheless, all of them have certain limitations: uncertainty in duration, limited efficacy of vaccines, and lack of effective drugs for COVID-19 treatment. So, the issue of creating drugs for symptomatic and etiotropic therapy is still relevant. This review summarizes the current knowledge of using natural compounds as anti-SARS-CoV-2 agents by analysing the results of in vitro studies and completed clinical trials (CTs). Also, this work highlighted the most active molecules and discussed the possibility of using some compounds in clinical practice.
Keywords
SARS-CoV-2, COVID-19, medicinal plants, natural products, in vitro studies, clinical trialsIntroduction
The coronavirus disease 2019 (COVID-19) pandemic is remaining an extremely serious public health problem in the world despite the declining numbers of infections and deaths. There were over 750 million confirmed cases of COVID-19. Currently, the pandemic has claimed approximately seven million lives as of February 2023 [1].
Problems with global access, uncertainty about the duration of protection and efficacy of existing vaccines, and the small number of World Health Organization (WHO)-approved drugs indicate the need for further research into new treatments for COVID-19 [2].
The growing interest to investigate the natural compounds against COVID-19 illustrated by the informational search in the PubMed database. Using query “(SARS-CoV-2 OR COVID-19) AND (natural compound OR phytocomponent OR phytoconstituent OR secondary metabolite)” one obtains 374 records in 2020, 804 records in 2021, and 794 records in 2022 (the data for 2022 is still incomplete on the time of manuscript preparation). It is their broad pharmacological spectrum and safety that make natural compounds a rich source of potential anti-coronavirus agents exhibiting symptomatic and etiotropic action [3–5].
This review has collected publications of in vitro studies that provide information on biochemical and cellular assays of natural compounds from medicinal plants. For clinical studies, we have analyzed the studies that evaluate the efficacy of single molecules of medicinal plants but have not considered extracts, since this makes it difficult to determine the effect of a particular molecule.
This study aims to provide information facilitating candidate selection studies for the initiation of clinical trials (CTs) and to evaluate the probability of using a natural compound as a treatment for COVID-19 based on the results of completed CTs. For this purpose, we reviewed several hundred relevant publications and selected the most active in vitro studies (Figure 1) that inhibit severe acute respiratory syndrome coronavirus-2 (SARS-CoV-2) targets [spike protein (S-protein), 3-chymotrypsin-like protease (3CLpro), papain-like protease (PLpro), RNA-dependent RNA polymerase (RdRp), and non-structural protein 13 (nsp13)] or reduce viral replication in various cell cultures [Vero E6, Calu-3, HEK293T-human angiotensin I converting enzyme 2 (ACE2), and Michigan Cancer Foundation-7 (MCF7)]; and report the results of CTs on the treatment of SARS-CoV-2 infection with phytocomponents from medicinal plants. The structure of the most active compounds in in vitro tests is shown below.
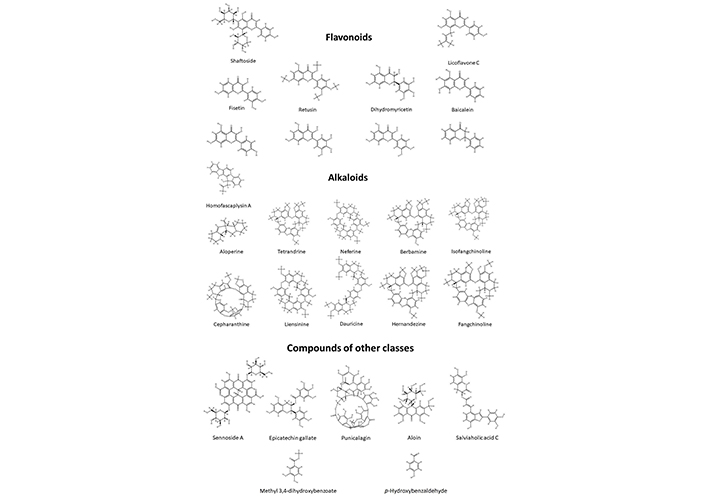
Examples of structures of phytocomponents from medicinal plants that demonstrated anti-coronavirus activity in biochemical or cellular assays
As one may see from Figure 1, ten phytocomponents are belonging to the class of flavonoids (baicalein, kaempferol, etc.), eleven to alkaloids (aloin, berbamine, etc.), while the others are quite diverse in chemical structure and could be broadly classified as “Compounds of other classes”.
The most active compounds in in vitro assays are discussed below. More in vitro data can be found in the Supplementary materials.
Inhibition of SARS-CoV-2 proteins in vitro
Blocking of S-protein/ACE2 interaction
Coronavirus S-protein plays a key role in the process of receptor recognition and cell membrane fusion. This protein consists of two subunits, S1 and S2. The S1 subunit contains a receptor-binding domain (RBD) that recognizes and binds to the ACE 2 host receptor. The S1 subunit, which induces the production of virus-neutralizing antibodies, is characterized by a high mutation rate, which may reduce the efficacy of vaccines and therapeutic antibodies. The S2 subunit ensures the fusion of the viral and cellular membranes. This process is activated by proteolysis, which releases the fusion peptide at the N-terminal portion of S2. The fusion peptide saturated with hydrophobic residues interacts with the cell membrane, triggering protein structural rearrangements, leading to membrane fusion and the entry of viral material into the cytoplasm. The two heptad repeat (HR) sites of the S2 subunit playing an important role in viral infections are highly conserved, both in structure and in the way they interact [6]. Inhibitors of RBD interaction with ACE2 may be used as anti-coronavirus agents.
We identified four phytocomponents of medicinal plants, which intimidate interaction between RBD and ACE-2 [7–9]. For Withanone potential activity was found in silico using docking and molecular dynamic simulation (scoring function value equals to −9.4 kcal/mol), which was confirmed in in vitro assay [half maximal inhibitory concentration (IC50) = 0.33 ng/mL] [8]. For glycyrrhetinic acid, betulinic acid, and oleanolic acid, good estimates of binding to RBD were found in silico (scoring function values equal to –8.6, –8.1, and –8.2 kcal/mol, respectively). Evaluation of computational predictions in in vitro assays confirmed that those compounds inhibit the interaction of the RBD and ACE2 with IC50 in the range from 0.1 μmol/L to 1 μmol/L [9].
Withanolides are naturally occurring chemical compounds. They are secondary metabolites produced via the oxidation of steroids and structurally consist of a steroid backbone bound to a lactone or its derivatives. They have medicinal value including anti-inflammation, anti-cancer, adaptogenic, and anti-oxidant effects. Withanone is a phytocomponent of Withania somnifera (Linn.) Dunal., also known as Ashwagandha in Indian Ayurvedic medicine [10].
3CLpro inhibitors studies in vitro
The main protease 3CLpro of SARS-CoV-2 plays a crucial role in the viral life cycle. Among all potential viral target proteins, the main protease stands out as the most highly conserved enzyme. It is also worth noting that human proteases with similar cleavage specificity do not exist; therefore, 3CLpro inhibitors are highly likely to be nontoxic [11, 12].
Sixty-six natural compounds from medicinal plants were examined for their ability to inhibit the 3CLpro [13–21]. Compounds with the highest IC50 values are presented in Table 1.
List of the most potent phytocomponents inhibiting 3CLpro
Phytocomponent | IC50 (μmol/L) | References | Scoring function (kcal/mol) | References |
---|---|---|---|---|
Epicatechin gallate | 1.58 | [13] | –8.2 | [22] |
CCG 50014* | 0.23; 0.15 | [13] | NA | NA |
Baicalein | 0.02; 0.94 | [13] | –7.3 | [23] |
Dihydromyricetin | 0.18 | [13] | –6.2 | [24] |
Hematoxylin | 0.22 | [13] | NA | [25] |
Schaftoside | 1.73 | [15] | –8.4 | [26] |
Sennoside A | 1.59 | [13] | –8.6 | [27] |
*: also known as 4-(4-fluorobenzyl)-2-p-tolyl-1,2,4-thiadiazolidine-3,5-dione. NA: not available
As one may see from Table 1, dihydromyricetin, baicalein, hematoxylin, and CCG 50014 are the most active 3CLpro inhibitors with submicromolar IC50 values.
Myricetin is a natural flavonol compound that can be found in many plants. According to the literature, myricetin and its derivatives showed anti-inflammatory, antioxidant, antibacterial, and antiviral activities [28].
Hematoxylin is a naturally occurring compound derived from the logwood tree, Haematoxylon campechianum. As staples of histopathological studies, hematoxylin has become the inimitable scaffold on which many of our dermatological diagnoses are made [29, 30].
The major constituent and most important polyphenolic catechin in green tea (Thea sinensis L.) is epigallocatechin-3-gallate, which has shown numerous beneficial properties, such as anti-inflammatory ability, antioxidative properties, and antiviral effects against various viruses [31].
PLpro inhibitors studies in vitro
PLpro of SARS-CoV-2 plays an important role in virus maturation, disruption of host inflammation regulation, and antiviral immune response PLpro’s multiple functions make it a promising anti-coronavirus drug target [32].
Thirty-four phytocomponents were analyzed for inhibition of papain-like cysteine protease [14, 15, 21, 33–36]. Compounds with the best results in the context of inhibition activity are shown in Table 2.
List of the most potent phytocomponents inhibiting PLpro
Name of chemical compound | IC50 (μmol/L) | References |
---|---|---|
Methyl 3,4-dihydroxybenzoate | 3.76 | [33] |
p-Hydroxybenzaldehyde | 3.99 | [34] |
Schaftoside | 3.91 | [15] |
Herba Desmodii styracifolii is widely used in Traditional Chinese Medicine (TCM) for inflammation, pyrexia, and kidney stone treatment. Schaftoside, one of the most abundant flavonoids of this plant, possessed different physiological activities including anti-inflammatory. We also found in literature in silico data which show that the scoring function of schaftoside interaction with PLpro is about –8.7 kcal/mol [37, 38].
RdRp in vitro study
The RdRp domain (about 600 amino acid residues) is a replicase, which in complex with other proteins (nsp7, nsp8) realizes replication of genomic RNA and translation of subgenomic RNA. Significant similarity at the level of amino acid sequences is found in different families of viruses with a single-stranded genome sequence. Thus, there is a variety of opportunities for drug repositioning [39, 40].
Information was collected on two compounds (baicalin, baicalein). Both confirmed the capability of chain-termination during RNA synthesis in an in vitro study that may cause by different mechanisms such as inhibition of replicase. In silico tests showed that both compounds could strongly bind to SARS-CoV-2 RdRp with an estimated binding energy (scoring function) of about −8.7 kcal/mol and −7.8 kcal/mol, respectively [41].
Baicalein and his glucuronide baicalin are flavones and the main active compounds of Scutellaria baicalensis George, that are widely used in TCM for treating infection of multiple different viruses. These compounds also exhibit inhibitory activity against the main protease 3CLpro [42].
nsp13 in vitro study
The nsp13 protein functions as a helicase using ATP, by uncoupling double-stranded RNA intermediates. This protein is also involved in cap formation. nsp13 helicase is the key component of the replication-transcription complex (RTC), which is necessary to complete its infectious life cycle [43].
Twenty-two compounds were examined [44, 45]. Compounds with the most inhibition activity are presented in the Table 3 and are taken from paper by Corona et al. [44]. The results of in silico estimations were extracted from different sources.
List of compounds with the highest IC50 value [44]
Name of chemical compound | IC50 (μmol/L) | Scoring function (kcal/mol) | References to in silico |
---|---|---|---|
Baicalein | 2.9 | –8.9 | [46] |
Kaempferol | 0.76 | –6.9 | [47] |
Licoflavone C | 1.34 | NA | NA |
Myricetin | 0.41 | –7.0 | [47] |
Quercetin | 0.53 | –6.9 | [47] |
Kaempferol is a flavonoid extracted from some medicinal herb like Kaempferia galanga L. Pharmacological effects of kaempferol including antioxidant, anti-inflammatory, antimicrobial, and antiviral properties were also reported [48–50].
Licoflavone C is one of the main flavonoids of licorice (Glycyrrhizae Radix or Liquiritiae Radix) that is traditionally used to treat various diseases including inflammation and gastric ulcers. The protective effect of licorice flavonoids may at least in part be due to their antioxidant activity through the nuclear factor erythroid 2-related factor 2 pathway and anti-inflammatory activity through the nuclear factor kappa-light-chain-enhancer of activated B cells pathway [51].
HEK293T-ACE2 in vitro study
HEK293T-ACE2 is constructed by transduction of ACE2 into HEK293T cells, followed by stable cell selection. These cell lines express ACE2 messenger RNA (mRNA) at high levels on the cell surface and are amenable to infection by viruses [52].
One hundred and seventy-five compounds were tested on this cell line [20, 45, 53–57]. The most active compounds in the context of preventing the infiltration of the virus into the cell are shown in Table 4. All information in the table was extracted from the He et al. [20] study.
List of compounds with the highest results in cell line HEK293T-ACE2 [20]
Name of chemical compound | EC50 (μmol/L) | CC50 (μmol/L) | SI |
---|---|---|---|
Aloperine | 0.28 | > 50 | > 176.87 |
Berbamine | 1.28 | > 50 | > 38.94 |
Cepharanthine | 0.32 | > 50 | > 158.58 |
Dauricine | 1.43 | > 50 | > 34.99 |
Fangchinoline | 0.94 | > 50 | > 53.39 |
Hernandezine | 0.11 | > 50 | > 448.83 |
Isofangchinoline | 1.11 | > 50 | > 45 |
Isoliensinine | 0.923 | > 50 | > 54.17 |
Liensinine | 1 | > 50 | > 49.8 |
Neferine | 0.95 | > 50 | > 52.87 |
Tetrandrine | 0.9 | > 50 | > 55.8 |
EC50: half maximal effective concentration; CC50: half cytotoxic concentration; SI: selectivity index
Aloperine, a quinolizidine-type alkaloid, was first isolated from the seeds and leaves of the herbal plant, Sophora alopecuroides L. It has known for its antitumor, anti-microbial, anti-oxidative, and immune regulator effects [58].
Cepharanthine is the only approved drug for human use in the large class of bisbenzylisoquinoline alkaloid. This natural product, mainly isolated from the plant Stephania cephalantha Hayata and exhibits multiple pharmacological properties including anti-oxidative, anti-inflammatory, immuno-regulatory, and anti-viral [59].
Hernandezine is an alkaloid isolated from the Chinese medicinal herb manyleaf meadowure rhizome and root. Hernandezine is tested like an anticancer and antidiabetic agent. Also, in vitro assay demonstrates its capability to inhibit tumor necrosis factor alpha (TNF-α) expression, which causes an anti-inflammatory effect [60–62].
Berbamine is one of the main components in various species of Berberis L. This substance is known for its anti-cancer properties that are realized by various mechanisms, including anti-inflammatory [63–65].
Vero E6 in vitro study
Vero E6 is a cell line exhibiting epithelial morphology that was isolated from the kidney of an African green monkey. The Vero cells are the most employed for their high susceptibility to the replication of a wide range of viruses, including SARS-CoV- 2 [66].
Twenty-five compounds were tested on this cell line [7, 14, 15, 17, 43, 56, 67–72]. The list of compounds that most effectively inhibit SARS-CoV-2 replication is shown in Table 5.
List of compounds with the best EC50 values obtained in cell line Vero E6
Name of chemical compound | EC50 (μmol/L) | References |
---|---|---|
Punicalagin | 0.196 | [45] |
Retusin | 0.4 | [67] |
Salvianolic acid C | 3.41 | [56] |
Aloin | 0.095 | [72] |
Cepharanthine | 0.35 | [71] |
Punicalagin is an ellagitannin found in the peel of the pomegranate (Punica granatum L.) and is its main constituent. A number of studies prove the anti-inflammatory and antioxidant properties of this compound [73].
Aloin is an active ingredient found in the leaves of medicinal plants of the genus Aloe. Aloin has been investigated in in vitro and in vivo research for its anti-inflammatory, anticancer, antibacterial, and antioxidant activities [74].
Calu-3 in vitro study
Calu-3 cells are a human lung epithelial cell line that can support the propagation of SARS-CoV-2. These cells can promote the replication of SARS-CoV-2 viruses expressing the spike gene with an intact furin cleavage site [75].
Fifteen compounds were tested on this cell line [41, 53, 67, 76, 77]. The list of compounds that most effectively inhibit SARS-CoV-2 replication is shown in Table 6.
List of compounds with the best EC50 values obtained in cell line Calu-3
Name of chemical compound | EC50 (μmol/L) | CC50 (μmol/L) | SI | References |
---|---|---|---|---|
Baicalein | 1.2 | 91 | 76 | [41] |
Fisetin | 2.03 | 256 | 126 | [76] |
Homofascaplysin A | 1.1 | 5 | 5 | [77] |
Kumatakenin | 0.3 | 214 | 716 | [67] |
Myricetin | 0.91 | 716 | 787 | [76] |
Quercetin | 2.4 | 852 | 355 | [76] |
Retusin | 0.4 | 3,333 | 8,333 | [67] |
Fisetin is a flavonol that is an isolate from Rhus cotinus L. A number of in vitro studies have confirmed the antioxidant and anti-inflammatory biological activity [78, 79].
Retusin is a methylated flavonoid that occurs in different plants like Larrea cuneifolia, Solanum pubescens, and Siparuna cristata. In vitro studies showed the potential free radical-scavenging capacities of this compound [80].
MCF7 in vitro study
MCF7 are epithelial cells isolated from the breast tissue of a 69-year-old female patient with metastatic adenocarcinoma. These cells demonstrate a high replication capacity of SARS-CoV-2 [81, 82].
Three compounds (caffeic acid phenethyl ester, Withaferin-A, and Withanone) were tested on this cell line. All of them were characterized as active inhibitors of SARS-CoV-2 replication [83].
Caffeic acid phenethyl ester is a natural bioactive compound, which occurs in many plants, and also has known as the most extensively investigated active component of ethanol and butanol extracts of the plant which possess many biological activities including antibacterial, antiviral, antioxidant, and anti-inflammatory effects [84–86].
Clinic trials
As a result of the analysis of available data on CTs, 265 trials of potential drugs for the etiotropic and symptomatic treatment of COVID-19 are underway or have been completed. Of these, 32 were conducted using medicinal plant compounds as a pharmaceutical substance. Summarizes information about these studies is presented in Table 7 [87–143]. The results of CTs were extracted from corresponding publications.
List of results of CTs of potential drugs for the treatment of COVID-19 using natural compounds from medicinal plants
Name of chemical compound | Study design; sample size (control group/investigate group) | References on protocol studies | Results | References on results |
---|---|---|---|---|
Artemisinin | An open-label, non-randomized, controlled trial; 41 (23/18) | [87] | Positive effects | [111] |
Artesunate | Not fully described; 43 (25/18) | - | Positive effects | [112] |
Berberine | Not fully described; 35 (17/18) | - | No significant difference | [113] |
Cannabidiol | A single-center, randomized, parallel, double-blind, placebo-controlled CT; 104 (52/52) | [88] | No significant difference | [114] |
Colchicine | A randomized controlled CT; 90 (45/45) | [89] | Positive effects | [115] |
Colchicine | A randomized, open-labeled, CT; 80 (40/40) | [90] | Positive effects | [116] |
Colchicine | A randomized, double-blinded, placebo-controlled CT; 72 (36/36) | [91] | Positive effects | [117] |
Colchicine | A multicenter, randomized CT; 152 (89/63) | [92] | Positive effects | [118] |
Colchicine | A randomized controlled CT; 112 (34/78) | - | Positive effects | [119] |
Colchicine | A randomized controlled CT; 336 (165/171) | - | Positive effects | [120] |
Curcumin | A randomized double-blind placebo-controlled trial; 48 (24/24) | [93] | Positive effects | [121] |
Curcumin | A randomized controlled, double-blind, placebo-controlled CT; 60 (30/30) | [94] | Positive effects | [122] |
Curcumin | A non-randomized open-label, parallel-group CT; 41 (21/20) | [95] | Positive effects | [123] |
Curcumin | Randomized double-blind CT; 40 (20/20) | [96] | Positive effects | [124] |
Curcumin | A non-randomized open-label, parallel-group CT; 60 (30/30) | [97] | Positive effects | [125] |
Curcumin | A randomized, double-blind, placebo-controlled trial; 50 (25/25) | [98] | Positive effects | [126] |
Curcumin | A randomized, parallel-group trial; 140 (70/70) | [99] | Positive effects | [127] |
Glycyrrhizin | A single-center, randomized, double-blind, placebo-controlled CT; 50 (25/25) | [100] | Positive effects | [128] |
Glycyrrhizin | A randomized triple-blind placebo-controlled CT; 125 (62/63) | [101] | Positive effects | [129] |
Glycyrrhizin | Retrospective study [in study compares two groups of people: those with the disease under study (cases) and a similar group of people who do not have the disease (controls)]; 147 (66/81) | - | Positive effects | [130] |
Hesperidin | Control group, with parallel, double-blind, and randomized groups; 40 (20/20) | [102] | No significant difference | [131] |
Hesperidin | Randomized, double-blind, placebo-controlled study; 216 (109/107) | [103] | Positive effects | [132] |
Luteolin | Randomized-controlled pilot study; 12 (7/5) | - | Positive effects | [133] |
Luteolin | Randomized-controlled study; 69 (59/10) | - | Positive effects | [134] |
Plitidepsin | Randomized, parallel, open-label; 30 (15/15) | [104] | Positive effects | [135] |
Plitidepsin | Case study (research of one case); 1 | - | Positive effects | [136] |
Quercetin | Single center, randomized, controlled trial; 429 (380/49) | [105] | No significant differences | [137] |
Quercetin | Randomized, parallel assignment, placebo-controlled CT; 60 (30/30) | [106] | Positive effects | [138] |
Quercetin | Single-center, prospective, randomized, controlled cohort study; 120 (60/60) | [107] | Positive effects | [139] |
Quercetin | Pilot, randomized, controlled and open-label CT; 42 (21/21) | - | Positive effects | [140] |
Resveratrol | Randomized, placebo-controlled trial; 30 (14/16) | [108] | No significant differences | [141] |
Resveratrol | Not fully described; 230 (200/30) | [109] | Positive effects | [142] |
Resveratrol | Double-blind, randomized, placebo-controlled trial; 100 (50/50) | [110] | Positive effects | [143] |
-: not applicable
As shown in Table 7, a total of 33 CTs were. Of these, 28 reported a positive effect and 5 reported no significant difference. These studies considered 12 compounds and only 2 of them not reported positive results (berberine and cannabidiol) [87–143]. The most studied compounds are considered below.
Curcumin
Curcumin is a polyphenol contained in the root of the Curcuma longa. This compound has a wide range of pharmacological effects, including anti-inflammatory properties. One possible mechanism is inhibition of nuclear factor kappa-light-chain-enhancer of activated B, which leads to lower levels of cytokines and proinflammatory chemokines such as interleukin-1β (IL-1β), IL-18, IL-6, TNF-α, and monocyte chemoattractant protein-1 [144]. The result of some in silico and in vitro studies present in Figure 2.
The wide range of pharmacological properties is not the only reason why this molecule was chosen as a subject in CTs. As can be seen in Figure 2, curcumin also demonstrates inhibitory activity to SARS-CoV-2 in in silico and in vitro studies [145–147]. This suggests that the effect of curcumin not be limited to its anti-inflammatory properties, but may also be the result of direct anti-SARS-CoV-2 action.
In this review, 7 CTs were selected in which curcumin was studied as a potential drug for the treatment of COVID-19. A summary of these studies is presented in Table 8.
Results of CTs of curcumin
CT phase | Evaluated parameters | Patient groups (placebo/control) | Results | References |
---|---|---|---|---|
Not specified | Clinical symptoms, O2 saturation, general analysis blood, C-reactive protein (CRP), CT | 24/24 | Decrease in overall Wisconsin Questionnaire score. Level differentiation, high-sensitivity CRP levels, and the degree of lung damage on CT were not different between the two groups. | [93] |
3 | Levels of serum secretion of inflammatory cytokines IL-1β, IL-6, and TNF-α | 30/30 | Curcumin can significantly increase the frequency and function natural killer cells compared to the group that received a placebo. | [94] |
3 | Symptoms (fever, chills, myalgia, cough), O2 saturation | 21/20 | Reduced hospitalization time, O2 saturation was significantly higher in the group treatment. | [95] |
Not specified | Clinical signs and gene expression of T-box transcription factor 21, GATA binding protein 3, RAR-related orphan receptor C, and forkhead box P3, serum levels of cytokines interferons-γ (IFN-γ), IL-4, IL-17, and transforming growth factor beta | 20/20 | Serum levels of IFN-γ and IL-17 decreased, and IL-4 and transforming growth factor beta increased in the curcumin group compared with the placebo. TBX21 and FOXP3 gene expression was significantly reduced. | [96] |
3 | Clinical signs and mRNA of IFN-γ, IL-1β, IL-6, monocyte chemoattractant protein-1, and TNF-α and levels of the inflammatory mediators IL-1β, IL-6, and TNF-α in serum | 30/30 | Clinical manifestations and laboratory parameters improved after curcumin treatment, IFN-γ and TNF-α mRNA expression decreased significantly. There was a significant difference between curcumin and the control group in the expression of IFN-γ, IL-1β, and IL-6; and a significant difference between curcumin and controls in serum levels of IL-1β. | [97] |
Not studied | Clinical symptoms, O2 saturation | 70/70 | Patients with moderate and severe symptoms who received curcumin treatment showed an earlier recovery compared to patients in the control group. Curcumin treatment shortened the duration of hospitalization in patients with moderate to severe symptoms; the curcumin treatment group had fewer deaths. | [99] |
As can be seen from Table 8, all presented CTs indicate an improvement in clinical and biochemical parameters in the curcumin treatment groups compared with the placebo. However, the design features of these CTs, which include small patient sample groups and lack of “blinding” do not allow objective conclusions to be drawn about the feasibility of curcumin used in the general population.
Quercetin
Quercetin is a natural flavonoid found in various fruits, vegetables, leaves, and grains like red onion or bog blueberry. It is well known for its antioxidant and anti-inflammatory properties. Quercetin prevents TNF-α from directly activating extracellular signal-related kinase, which are potent inducers of inflammatory gene expression and protein secretion [148].
As in the case of curcumin, interest in quercetin is supported not only by its safety and anti-inflammatory activity but also by the positive results of the in silico and in vitro studies discussed earlier and summarized in Figure 3. And, as before, we can assume that the anti-COVID-19 properties are may achieved both by the symptomatic and etiotropic type of action.
Two CTs were reviewed for quercetin (Table 9).
Quercetin CT results
CT phase | Estimated options | Patient groups (placebo/control) | Results | References |
---|---|---|---|---|
3 | Level CRP, alkaline phosphatase, lactate dehydrogenase (LDH) | 30/30 | Quercetin administration was significantly associated with earlier discharge and decreased serum alkaline phosphatase levels, CRP, and LDH. | [138] |
Unknown | Clinical symptoms, polymerase chain reaction test | Standard therapy + quercetin—21; standard therapy—21 | In the standard therapy group, 2 patients received a negative test for SARS-CoV-2 and 4 patient’s symptoms partially improved. After 2 weeks, the remaining 5 patients in the quercetin group tested negative for SARS-CoV-2, then while in the standard care group, of the remaining 19 patients, 17 showed a negative result at week 2, one negative result at week 3, and one patient, still positive, died at day 20. On the background of quercetin therapy, LDH, and ferritin levels decreased, CRP and D-dimer. | [140] |
Quercetin CTs data presented in Table 9 show a positive effect of the drug on the course of COVID-19 in combination with remdesivir or favipiravir in small samples of patients. However, additional trials are required to confirm the efficacy.
Colchicine
Colchicine is a plant alkaloid isolated from plants of the genus Colchicum L. It is a drug approved by the Food and Drug Administration as a treatment for gout, that has a wide range of anti-inflammatory effects, including decreasing the expression of adhesion molecules on neutrophil membranes and modulating the production of anti-inflammatory cytokines (IL-1 and IL-6) as well as TNF-α. These properties and a long history of use have attracted the attention of researchers to this molecule as a potential anti-COVID-19 agent [149]. Six CTs were selected, the results of which are shown in Table 10.
Colchicine CT results
Phase | Estimate options | Patient groups (placebo/control) | Results | References | |
---|---|---|---|---|---|
4 | Need for artificial ventilation, length of stay in intensive care, and mortality | Ivermectin + colchicine + standard care—45; colchicine + standard care—45; standard care—45 | Colchicine was associated with clinically significant reductions in oxygen demand days, intensive care unit length of stay, and less mortality, while ivermectin added no beneficial effect. | [115] | |
2 | Need for artificial lung ventilation, length of stay intensive therapy, mortality | 40/40 | Serum ferritin levels in most patients who received colchicine, returned to normal in contrast to the control group, whose serum ferritin levels remained high. Similarly, the mean CRP and D-dimer values after treatment among participants in the colchicine group were significantly lower than in the control group. Patients in the colchicine group stayed in the hospital for a shorter period compared with the control group. | [116] | |
3 | Need for artificial lung ventilation, length of stay in intensive care, mortality | 36/36 | Colchicine reduced the duration of additional oxygen therapy and hospitalization. The drug was well tolerated. Since death was a rare event, one cannot argue that colchicine reduced COVID-19 mortality. | [117] | |
3 | CT | Colchicine + standard therapy—89; standard therapy—63 | According to this study, colchicine can improve clinical outcomes and reduce pulmonary infiltration in patients with COVID-19. | [118] | |
Not studied about | Inflammatory markers, mortality | Colchicine + standard treatment—34; standard treatment—78 | Patients in the colchicine group had lower mortality rates, lower intubation rates, and a higher percentage of discharge. | [119] | |
Not studied about | Length of hospital stay | Colchicine + standard treatment—165; standard treatment—171 | The average length of hospital stay in the colchicine group was significantly short. | [120] |
As can be seen in Table 10, in the studied trials colchicine was used in combination with COVID-19 standard therapy that is not specified in the available publications. In 6 of them, the effectiveness of colchicine in combination with standard therapy was established. Reduced hospitalization time, good tolerability of the drug, and improved biochemical parameters were shown. However, these studies are characterized by disadvantages similar to those of curcumin. It is also worth noting that in the latest WHO recommendations, colchicine is not recommended for use in patients with non-severe COVID-19.
Possible synergistic/additive effects of secondary metabolites
Since the present review considers mainly natural compounds from medicinal plants, we evaluated the prospects for studying the anti-coronaviral activity of plant extracts taking into account the potential synergistic/additive action of their phytocomponents. We found groups of compounds represented in the phytochemical composition of a single plant during the analysis of our results. Information about the content of the previously discussed compounds in plants is given in Table 11.
The list of plants in the phytochemical composition of which the largest number of phytochemical components considered
Plant’s name | Compound’s name | References |
---|---|---|
Polygonum aviculare L. (Polygonaceae) | Kaempferol; myricetin; oleanolic acid; luteolin; quercetin | [150] |
Vitis vinifera L. (Vitaceae) | Myricetin; kaempferol; oleanolic acid; resveratrol; quercetin; luteolin | [151] |
Nelumbo nucifera Gaertn. (Nelumbonaceae) | Isoliensinine; liensinine; neferine | [152] |
Punica granatum L. (Punicaceae) | Luteolin; betulinic acid; punicalagin | [153] |
As shown by Yu et al. [150], kaempferol, myricetin, luteolin, and quercetin can be isolated from ethanol and butanol extracts of the plant Polygonum aviculare L. Kaempferol, quercetin, and myricetin are capable of inhibiting nsp13 (Table 3) as described above. Additionally, myricetin has been described as having an inhibitory activity against 3CLpro (Table 1) and quercetin has been demonstrated to inhibit viral replication on Calu-3 cells (Table 6). Two CTs of luteolin and 2 CTs of quercetin have shown a positive effect on the course of COVID-19 (Table 7). The described results make it reasonable to test ethyl and butanol extracts of Polygonum aviculare L. for their antiviral activity taking into account the possible synergistic/additive effects of its constituent chemical compounds.
Vitis vinifera L. has a similar composition of phytocomponents with laboratory-confirmed anti-coronaviral activity. It contains resveratrol for which the inhibition of coronavirus replication on Vero E6 cells was confirmed, as well as a positive effect on the course of COVID-19 in two CTs (Table 7) [154] in addition to the phytocomponents described above. Laboratory testing of the extract from the leaves of Vitis vinifera L. was carried out by Zannella et al. [151]. This study established the possibility of inhibiting SARS-CoV-2 replication in Vero cells.
Isoliensinine, liensinine, and neferine are phytocomponents of Nelumbo nucifera Gaertn. that can be isolated from its seeds. These compounds have been shown to inhibit SARS-CoV-2 entry into HEK293T-ACE2 cells. These facts discuss the feasibility of testing the anticoronavirus activity of Nelumbo nucifera Gaertn. seed extract.
The inhibition of viral entry into the cell and the inhibition of viral replication were shown in biochemical and cellular assays, or betulinic acid and punicalagin, respectively. However, as shown in a study by de Oliveira et al. [155], an extract from the rind of Punica granatum L. demonstrated not only the ability to inhibit virus entry into the cell by interaction with the S-protein but also a decrease in 3CLpro activity.
Conclusions
In this review, we summarized the current information about compounds from medicine plants that inhibit SARS-CoV-2 targets, reduce its replication in cell lines and/or studied different CTs. In in vitro assay, most research compounds are flavonoids like baicalein, kaempferol, myricetin, quercetin, and others. They demonstrate good inhibition activity in biochemical and cell assay. Since flavonoids have long been used as dietary supplements and their anti-inflammatory and immunostimulatory activity have been supported by a number of studies, they may be good candidates to launch new CTs or to start larger and more reliable studies for previously clinically tested compounds.
In spite of the fact that in the majority of CTs, preparations containing compounds of natural origin obtained positive results, it is extremely difficult to draw reliable conclusions about the effectiveness and prospects of the studied compounds. This is primarily due to the design of the studies themselves and the small groups of subjects. More than 90% of the studies were performed without blind control. Among the trials presented, there are trials with contradictory results under the same conditions. However, given the fact that for most of the presented chemical compounds a wide experience of use as dietary supplements and a low probability of side effects is available, some of these phytocomponents might be an effective adjunct to the standard therapy according to the WHO recommendations.
Abbreviations
3CLpro: |
3-chymotrypsin-like protease |
ACE2: |
angiotensin I converting enzyme 2 |
COVID-19: |
coronavirus disease 2019 |
CTs: |
clinical trials |
IC50: |
half maximal inhibitory concentration |
IL-1β: |
interleukin-1β |
MCF7: |
Michigan Cancer Foundation-7 |
mRNA: |
messenger RNA |
nsp13: |
non-structural protein 13 |
PLpro: |
papain-like protease |
RBD: |
receptor-binding domain |
RdRp: |
RNA-dependent RNA polymerase |
SARS-CoV-2: |
severe acute respiratory syndrome coronavirus-2 |
S-protein: |
spike protein |
TNF-α: |
tumor necrosis factor alpha |
WHO: |
World Health Organization |
Supplementary materials
The supplementary material for this article is available at: https://www.explorationpub.com/uploads/Article/file/100817_sup_1.pdf.
Declarations
Author contributions
AK: Data curation, Visualization, Writing—original draft. NI: Data curation, Writing—original draft. IG and VP: Conceptualization, Supervision.
Conflicts of interest
The authors declare that they have no conflicts of interest.
Ethical approval
Not applicable.
Consent to participate
Not applicable.
Consent to publication
Not applicable.
Availability of data and materials
Not applicable.
Funding
The study is performed in the framework of the
Copyright
© The Author(s) 2023.