Abstract
The outbreak of severe acute respiratory syndrome coronavirus 2 (SARS-CoV-2) infection in December 2019 quickly escalated to pandemic levels and had a severe impact on public health. There are 761 million confirmed coronavirus disease 2019 (COVID-19) cases, with over 6.88 million deaths worldwide till March 2023. Severe cases of the disease caused critical respiratory failure followed by multiorgan involvement. Clinical escalation of COVID-19 has been correlated with markedly increased plasma inflammatory markers [e.g., C-reactive protein (CRP)] and pro-inflammatory cytokine levels [e.g., interleukin (IL)-6, tumor necrosis factor-α (TNF-α)]. Therapeutic options have mostly utilized corticosteroids, antivirals (e.g., remdesivir), and monoclonal antibody-based immunomodulation (e.g., tocilizumab). These existing treatments have adverse side effects, inadequate efficacy, and limitations in administering to patients with comorbidities and other underlying diseases. Monoclonal antibody-based therapies and some of the antivirals are very costly. Many phytochemicals have previously reported anti-inflammatory, antiviral, and antioxidant properties. Studying the effectiveness of such phytochemicals against COVID-19 and identifying new plant-derived molecules with antiviral properties have been a focus since the SARS-CoV-2 outbreak. This review article has documented in vitro, in vivo, and clinical studies encompassing 28 different phytochemicals belonging to various chemical groups (e.g., polyphenols, alkaloids, terpenes) that show anti-COVID-19 activity. These findings suggest that multiple phytochemicals can interfere with virus entry and replication inside the host cell. Many of them can protect from cytokine storm by acting on intracellular signalling pathways in addition to inhibiting virus multiplication. Phytochemicals may prove useful in alleviating post-COVID complications associated with kidney injury, and central nervous system complications, as well. Plant-derived compounds are usually cheaper and have fewer side effects. But, developing new formulations with better absorption and bioavailability remains a priority. This review informs the readers of the current status and indicates the ongoing research in this highly relevant field.
Keywords
Coronavirus disease 2019, severe acute respiratory syndrome coronavirus 2, phytochemicals, inflammation, cytokine storm, antivirals, combination therapyIntroduction
Severe acute respiratory syndrome coronavirus 2 (SARS-CoV-2) has been wreaking havoc globally since initial reports emerged from China in December 2019. World Health Organisation (WHO) declared the disease caused by this virus, coronavirus disease 2019 (COVID-19), as a global pandemic in March 2020, and transmission of this pathogen remains large in populations around the world. The pandemic was terrible for both human health and socio-economic conditions. According to the WHO report, 761,402,282 COVID-19 cases were confirmed globally, with 6,887,000 deaths as of 29th March, 2023 [1].
Scientists, clinicians, and pharmaceutical companies rose to the challenge of putting a break on the pandemic by fast-tracking vaccine development, screening new therapeutics, and evaluating repurposed drugs for COVID-19. Clinical management of COVID-19 cases relied heavily on high-flow oxygen support and advanced non-invasive and invasive ventilation depending on the severity and risk of the admitted patients. Excessive inflammation and cytokine storm in patients with severe or critical COVID-19 are still tackled by systemic use of corticosteroids [2], monoclonal interleukin (IL)-6 receptor-blocking antibodies (tocilizumab, sarilumab) [3] and repurposed rheumatoid arthritis drug baricitinib (Janus kinase inhibitor) [4]. Several broad ranges of antivirals received authorization for use in COVID-19. These mainly interfere with the viral replication process, such as remdesivir [5], molnupiravir [SARS-CoV-2 RNA-dependent RNA polymerase (RdRp) inhibitors] [6], and nirmatrelvir/ritonavir [combination of peptidomimetic inhibitor of SARS-CoV-2 3-chymotrypsin-like protease [3CLpro, also known as main protease (Mpro)], and drug-metabolizing cytochrome P450 (CYP) 3A-inactivator used as a pharmacokinetic enhancer, respectively] [7].
To neutralize viral particles, convalescent plasma therapy was also initially tried to treat severe cases of illness and patients with comorbidities [8]. Structure-based antibodies targeting the SARS-CoV-2 cellular entry process were developed, e.g., meplazumab (anti-CD147 humanized antibody) [9], monoclonal antibodies developed from recovered patients (monoclonal antibody 4A8 [10], 47D11 [11], B38, H4 [12], etc.). The success of monoclonal antibodies inhibiting virus entry remains limited. None of the therapeutic options can defeat COVID-19 completely, and also, these available drugs have multiple drawbacks including high cost and adverse health effects. For example, concerns regarding tocilizumab use include reactivation of latent infections, neutropenia, hypofibrinogenemia, cardiac abnormalities, etc. [13]. Remdesivir may cause acute kidney injury (AKI) and cognitive dysfunction/delirium [14]. Long-term use of corticosteroids, though very affordable, may result in an increased risk of infections, glaucoma, osteoporosis, hypertension, psychological effects, and weight gain [2].
Quick emergency use authorization of several vaccines against COVID-19 has helped significantly to manage the pandemic and return to normalcy with the restoration of international travel. The requirement for the vaccine has led to the development of traditional inactivated killed vaccine formulations such as Bharat Biotech BBV152 COVAXIN® vaccine [15] and also fast-tracked vaccines utilizing newer technologies, such as mRNA vaccines (i.e., Pfizer-BioNTech Comirnaty® vaccine [16], Moderna mRNA-1273 [17]), DNA vaccine (ZyCoV-D) [18], and recombinant viral vectored vaccines (i.e., AstraZeneca/AZD1222) [19]. Concerns over the real-world effectiveness of some vaccines, limitations in the prevention of pathogen transmission, the durability of protection, vaccine hesitancy, and low uptake of booster shots remain a hindrance to managing the pandemic through mass vaccination. Newly emerging variants of SARS-CoV-2 and sub-variants that can evade vaccine-induced immunity have forced pharmaceutical companies to come up with modified vaccine formulations targeting these newer variants and recommendation of booster shots at regular intervals [20, 21].
This COVID-19 crisis creates a demand for safe and effective alternative therapeutics, either to be used alone or as an adjunct to existing standard care practices, for treating patients who are at risk of developing serious complications. Many plants derived phytochemicals and phytoconstituents have been proven to have a broad range of antiviral, antibacterial, anti-inflammation, and antioxidant properties. With a good safety profile, phytochemicals can be a much more affordable intervention for patients. Reports of in silico studies identifying phytochemicals binding with viral targets of SARS-CoV-2 provided the initial information regarding their potential [22–24]. This article has reviewed the recent experimental evidence of phytochemicals acting to inhibit viral targets and alleviate COVID-19-related pathologies that have emerged from cell culture, animal model-based studies, and the reports of clinical studies. The potential of phytochemicals for managing post-COVID complications in different organ systems has also been briefly mentioned. This review is intended for the readers to understand the current status of phytochemical-based therapeutics against COVID-19 and their usefulness in preparing against future similar outbreaks of viral diseases.
Literature search
For this review article, published literature has been collected from search engines including PubMed (http://www.ncbi.nlm.nih.gov/pubmed), ScienceDirect (https://www.sciencedirect.com), Google Scholar (http://scholar.google.com), and also the ClinicalTrials.gov database (https://clinicaltrials.gov/). Original articles, review articles, and clinical trial documents published in English have been curated manually for collecting data for writing this article. Searching was conducted using various combinations of keywords including “COVID-19 phytochemicals”, “COVID-19 clinical trials phytochemicals”, “COVID-19 flavonoids”, “COVID-19 curcumin”, “COVID-19 resveratrol”, “COVID-19 pterostilbene”, “COVID-19 phillyrin”, “COVID-19 tannins”, “COVID-19 alkaloids”, “COVID-19 terpenes”, “post-COVID complications phytochemicals”, “COVID-19 inflammation phytochemicals”, etc. The data taken was confirmed for suitability for this review article’s theme. The final data was prepared and has been discussed in the following sections.
SARS-CoV-2 virus life cycle and potential therapeutic targets
SARS-CoV-2 is an RNA virus with positive-sense, a length of approximately 29.9 kilobase pairs long single-stranded RNA genome belonging to the order Nidovirales, family Coronaviridae, and Subfamily Coronavirinae. Among 4 coronavirus genera [alphacoronavirus (α-CoV), betacoronavirus (β-CoV), gammacoronavirus (γ-CoV), and deltacoronavirus (δ-CoV)], SARS-CoV-2 belongs in β genera. The tightly packed long RNA polymers of the virus are enclosed by capsid. The protective capsid protein is called nucleocapsid (N) protein. The outer envelope (E) contains lipids and some specific virus-encoded structural proteins—spike (S) glycoprotein (consists of major two subunits S1 and S2), small membrane (M) protein, and E protein [25]. The viral entry process in the host cell utilizes the binding of the S1 subunit and human angiotensin-converting enzyme 2 (hACE2) cell surface receptor. The S2 subunit promotes virus and host cell membrane fusion. Transmembrane protease serine 2 (TMPRSS2) is present on the host cell surface and promotes viral entry to the host cell and cellular membrane fusion [26]. Two third portions of the genome have 5’ end open reading frame (ORF) 1a and 1b which translate into two large replicase polyproteins 1a and 1b (pp1a/pp1b). Papain-like protease (PLpro) and 3CLpro are two important cysteine proteases that cleave the polyproteins into 16 non-structural proteins (NSPs) 1–16 [27]. These NSPs are involved in the further translation and replication of the viral genome and have immunomodulatory effects. Importantly, NSP12, an RdRp, and its two cofactors, NSP7 and NSP8 replicate the viral RNA [28, 29]. In continuation, the remaining one-third portion of the genome comprises 4 major structural such as N, S, M, and E proteins and lineage-specific accessory proteins (ORF3a, ORF3b, ORF6, ORF7a, ORF7b, ORF8a, ORF8b, and ORF9b) [28]. Following the translation of SARS-CoV-2 mRNA into structural and accessory proteins, virion structural assembly occurs via the endoplasmic reticulum (ER)-Golgi intermediate compartment (ERGIC) into the lumen of secretory vesicles. Finally, mature virions are released from the cell by lysosomal exocytosis [25, 30].
Antiviral phytochemicals may attenuate infection of SARS-CoV-2 by targeting different steps of the life cycle of SARS-CoV-2 in the host. It may interfere with the SARS-CoV-2 receptor binding domain (RBD) and host angiotensin-converting enzyme 2 (ACE2) receptor interaction, preventing virus entry into host cell and S protein mediated cell-cell fusion. Phytochemicals may block viral replication, transcription, translation, and virion structural assembly. It has been reported that coronavirus S glycoprotein, TMPRSS2, 3CLpro, PLpro, NSP15, RdRp, and N protein are potential molecular targets of antiviral phytochemicals [31].
Immunopathology of COVID-19
Although inflammation is involved in the first-line of defence of the innate immune system, overexpression of it may be lethal. Normal immune responses are disrupted upon SARS-CoV-2 infection, triggering a dysregulated immune system and uncontrolled inflammatory responses which contribute to acute respiratory distress syndrome (ARDS) along with multiple organ failure [32] such as kidney, lung, liver, heart, central nervous system, and retinal injury in COVID-19 patients. COVID-19 patients are reported to have lymphopenia, characterized by decreased T cells such as CD4(+), CD8(+), B cell, and natural killer (NK) cell; increased neutrophil-lymphocyte ratio (NLR) [33]; monocytes and granulocytes (eosinophils and basophils) anomalies [33]; functional exhaustion of T cells [34] and NK cells [35]. The cytokine storm due to the overproduction of cytokine and chemokine triggered by overactivation of immune cells, may cause tissue damage both at the site of infection as well as at the systemic level [36]. During cytokine storm, increased serum levels of pro-inflammatory factors such as IL-1, IL-2, IL-6, IL-7, IL-12, IL-17, IL-18, interferon (IFN)-γ, tumor necrosis factor (TNF)-α, granulocyte colony-stimulating factor (GSCF), IFN-γ inducible protein-10 (IP-10), monocyte chemoattractant protein-1 (MCP-1), transforming growth factor (TGF)-β, and macrophage inflammatory protein (MIP)-1α have been reported in moderate to severe cases of COVID-19 (especially ICU patients) [37, 38]. Various cytokines trigger acute inflammation and cytokine storm via acting on different intracellular signalling pathways such as Janus kinase/signal transducer and activator of transcription (JAK/STAT), mitogen-activated protein kinases (MAPK), and nuclear factor kappa β (NF-κβ) pathway [36]. On the other hand, SARS-CoV-2 infection involves in dysregulation of reactive oxygen species (ROS), inducible nitric oxide synthase (iNOS), nuclear factor erythroid-derived 2-like 2 (Nrf2), pathways along with extracellular signal-regulated kinase (ERK), protein kinase B (Akt) signalling pathways, etc. [39]. Many studies have been demonstrated to evaluate the therapeutic anti-inflammatory role of various phytochemicals to control cytokine storm or hypercytokinemia.
Detailed discussion on immunopathology in COVID-19 is beyond the scope of this article. For a more in-depth discussion, readers are encouraged to consult review articles related to this topic [36, 38].
Phytochemicals and COVID-19
Several classes and groups of the United States Food and Drug Administration (FDA)-approved phytochemicals and natural derivatives have the potential to treat SARS-CoV-2 infection. Numerous in vitro, in vivo, and in silico studies with phytochemicals have been reported to show minimum or no toxicity and have antiviral, antibacterial, anticancer, antimalarial, antifungal, and anti-inflammatory properties. Phytochemicals and plant extracts are capable of curing several viral diseases like dengue virus, hepatitis virus, rotavirus, chikungunya virus, Japanese encephalitis virus (JEV), herpes virus (HV), Zika virus (ZIKV), influenza virus, human immunodeficiency virus (HIV), human papillomavirus (HPV), etc. On the basis of their antiviral properties, phytochemicals have been classified into several categories. Alkaloids, polyphenols, and terpenes are among the major categories of phytochemicals that possess antiviral properties [40]. Several natural compounds are selected in this study that affect potential targets of COVID-19 infection including, proteins like PLpro, 3CLpro, RdRp protein, ACE2 receptor, nucleotide-binding domain, leucine-rich-containing family, pyrin domain-containing protein (NLRP) 3 inflammasome, pathways like ROS, iNOS, JAK/STAT, ERK, Akt, etc. Phytochemicals are reported to downregulate cytokine levels and block viral-cell fusion, viral entry, viral replication, and transcription. Along with this, according to multiple clinical studies, infection time, hospitalization rate, and mortality rate are also lowered by several phytochemicals in SARS-CoV-2 treatment. In this study, the potential role of a few phytochemicals in the therapy of COVID-19 has been discussed.
Polyphenols
Plants have various bioactive polyphenolic compounds. They can be categorized based on their molecular mass, chemical construction, and intricacy into flavonoids (flavones, flavonols, flavanones, flavanonols, isoflavonoids, flavanols, and anthocyanidins) and non-flavonoids (phenolic acids, stilbenes, curcuminoids, lignans, tannins, and others). Polyphenol components have received considerable attention for their established biological properties, such as antiviral, antibacterial, antioxidative, and anti-inflammatory effects.
Flavonoids
Flavonoids represent natural phenolic phytochemicals commonly found in fruit, vegetables, grains, flowers, stems, bark, roots, tea, and wine. Their several biological activities, i.e., antiviral, antioxidant, immunomodulatory, anti-inflammatory, and antithrombotic activities have made them potential candidates to assess in therapy and management of COVID-19. Flavonoids are reported to suppress different inflammatory mediators by modulating cytokines as a potential therapeutic approach for the treatment of inflammation-related diseases like COVID-19.
Several preclinical and clinical studies involving flavonoids for the treatment and prophylaxis of SARS-CoV-2 infection are mentioned in the next section.
Quercetin
Quercetin [2-(3,4-dihydroxyphenyl)-3,5,7-trihydroxychromen-4-one or 3,3’,4’,5,7-pentahydroxyflavone] is a plant flavonoid found in many fruits and vegetables such as berries, lovage, capers, cilantro, dill, apples, and red onions possessing antiviral, antioxidant, anti-inflammatory, and immunomodulatory properties.
A study demonstrated the efficacy of quercetin in suppressing the SARS-CoV-2-S viral entry into Vero E6 cells with a half-maximal effective concentration (EC50) of 83.4 µmol/L and with a low half-maximal cytotoxic concentration (CC50 = 3.32 mmol/L) [41]. In the SARS-CoV-2 NSP15 enzyme assay, quercetin has been shown to inhibit NSP15 with a half-maximal inhibitory concentration (IC50) of approximately 13.79 μmol/L (4.17 μg/mL) [42]. In the lipopolysaccharide (LPS)‐induced acute lung injury model in C57/BL6 mice, quercetin reduced the levels of IL‐6, IL‐1β, and TNF‐α via downregulating the expression of cyclooxygenase‐2 (COX-2), high mobility group box 1 (HMGB1), iNOS, and NF‐κβ p65 phosphorylation [43].
A clinical trial has demonstrated the adjuvant effect of Quercetin Phytosome® (QP, containing quercetin combined with sunflower lecithin to increase uptake) supplementation, which may improve symptoms during the early stage of COVID-19 infection and prevent progression to severe disease when used alongside standard anti-COVID-19 therapies [44]. In a clinical study, involving 80 patients with early-stage mild to moderate symptoms of COVID-19, the efficacy of oral administration of quercetin in addition to standard care has been evaluated. The result showed that a reduction of lactate dehydrogenase (LDH, −35.5%), ferritin (−40%), C-reactive protein (CRP, −54.8%), and D-dimer (−11.9%) levels in patients that received quercetin. It also demonstrated speedy clearance of SARS-CoV-2, early improvement of the acute symptoms, and resolution of the host’s hyperinflammatory response compared to the control group [45]. Daily 1,000 mg quercetin supplementation for a week in combination with antivirals (i.e., remdesivir or favipiravir) is also safe and effective in improving COVID-19-associated markers such as serum levels of alkaline phosphatase (ALP), CRP, and LDH among hospitalized patients [46].
One of the main challenges during the COVID-19 pandemic has been the management of patients with pre-existing diseases and comorbidities. A recent study in diabetic mice with older age and pre-existing diabetic kidney disease (DKD), has demonstrated that quercetin treatment can protect from SARS-CoV-2 N protein-induced AKI by inhibiting mothers against decapentaplegic homolog 3 (Smad3) signalling and Smad3-mediated cell death [47]. A single-center, open-label, and randomized controlled cohort study explored the efficacy and safety of quercetin, vitamin C, and bromelain (QCB) supplementation in patients (429 in total) with at least one chronic disease and moderate-to-severe respiratory symptoms. Standard treatment plus QCB significantly reduced acute-phase reactants (APRs) such as CRP and ferritin levels when compared to standard treatment only. However this QCB supplementation group had more severe lung involvement compared to standard treatment as revealed by CT images. More studies are needed to make conclusions regarding such combination therapy. Quercetin is being evaluated in combination with other substances in different clinical trials. The efficacy and safety of NASAFYTOL® containing quercetin along with curcumin and vitamin D supplementation with standard care is under investigation in hospitalized patients with early COVID-19 symptoms. Another comparative randomized controlled trial (RCT) is evaluating the efficacy and safety of the combined effects of quercetin, zinc, vitamin C, and bromelain on hospitalized COVID-19 patients [48].
Baicalein
Baicalein (5,6,7-trihydroxyflavone) belongs to flavone mainly extracted from the roots of Scutellaria lateriflora and Scutellaria baicalensis. Baicalein treatment has been shown to inhibit inflammatory cytokines in in vitro and in vivo studies with the H5N1 influenza and in LPS-induced acute lung injury models. Baicalein could inhibit the secretion of IL‐6 and TNF‐α significantly in LPS‐induced human umbilical vein endothelial cells (HUVECs) and in A549 cells infected with the H5N1 virus [49]. It also significantly decreased the level of IL‐6, IL‐1β, and TNF‐α in Sprague‐Dawley rats via the suppression of NF‐κβ mediated inflammatory response and stimulation of Nrf2/heme oxygenase (HO)‐1 pathway in the LPS-induced acute lung injury model [50].
Baicalein has been evaluated in different in vitro studies to assess its inhibitory capacity against SARS-CoV-2 and its interactions with two major targets for viral replication machinery, RdRp, and 3CLpro. Zandi et al. [51] reported that baicalein and baicalin exhibited a dose-dependent anti-SARS-CoV-2 activity in Vero cells with EC50 of 4.5 µmol/L and 9.0 µmol/L respectively. Their study also showed baicalein to be much more potent in inhibiting RdRp activity compared to baicalin. Anti-SARS-CoV-2 activity of baicalein may also be attributed to its ability to inhibit viral protease 3CLpro in cells at the viral post-entry stage as demonstrated by different in vitro studies [52, 53]. Four naturally occurring active baicalein analogue compounds (scutellarein, myricetin, dihydromyricetin, and quercetagetin) strongly inhibited the activity of SARS-CoV-2 3CLpro in vitro with IC50 values of 5.80, 2.86, 1.20, and 1.24 mmol/L respectively [52].
Baicalein has the ability to relieve SARS-CoV-2-induced Vero E6 cell injury and also ameliorate LPS-induced acute lung injury in hACE2 transgenic mice infected with SARS-CoV-2 by suppressing the inflammatory cell infiltration and downregulating the expression of IL-1β and TNF-α in serum [54]. Baicalein exhibited marked inhibition against SARS-CoV-2 3CLpro by non-covalent bonding and also diminished levels of cytokines such as IL-1α, TNF-α, IL-4, and IL-10 in mouse sepsis models. It indicates a potential role of baicalein in preventing cytokine storm during COVID-19 [55].
Epigallocatechin-3-gallate
Epigallocatechin-3-gallate (EGCG), a compound from the class of flavan‐3‐ol, widely present in tea possesses high antioxidant activity. Several reports have indicated EGCG’s ability to interact with different targets of coronaviruses. EGCG in human embryonic kidney (HEK) 293/ACE2 cells blocked attachment of SARS-CoV-2 RBD to ACE2 with IC50 of 1.73 µg/mL and inhibited Middle East respiratory syndrome (MERS)- and SARS-CoV pseudotype lentiviral vectors with IC50 of 2.47 µg/mL [56] suggesting it can prevent viral entry. Similar findings have been corroborated in Vero 76 and Caco-2 cells, where EGCG efficiently attenuates SARS-CoV-2 infection with an EC50 value of 0.27 µg/mL (0.59 µmol/L) and EC90 value of 28 µg/mL (61 µmol/L) respectively [57]. The attenuation of in vitro replication of different coronaviruses can be attributed to EGCG inhibiting viral NSP15 endoribonuclease and 3CLpro activity [42, 58].
The property of EGCG to abrogate hyperinflammatory conditions in addition to preventing entry and replication of virus makes it an interesting candidate for drug development. This has been shown in symptomatic and asymptomatic COVID-19 patients where EGCG could resolve inflammatory responses indicated by serum biomarkers like IL-1β, IL-1, IL-6, MCP-1, and inhibit viral replication [59]. EGCG promoted the downregulation of ACE2 receptor and inflammatory factors, IL-1β, IL-6, TNF-α, IFN-α, -β, and -γ expression levels via inhibition of the activity of ROS/hypoxia-inducible factor (HIF)-1α/glycolysis axis in SARS-CoV-2-infected aged patients [60]. It also upregulated HO-1 expression level via stimulation of hepatic Nrf2 activity and decreased ROS generation and IL-6 production via reduction of elevated HIF-1α in COVID-19 infection [61].
In another in vitro study in Madin-Darby canine kidney (MDCK) cells, a combination of EGCG with cyclodextrin (CD-EGCG) showed antiviral effect against human coronavirus (HCoV)-229E at the adsorption step in short contact (EC50 = 2.5 ppm) and long contact conditions (EC50 = 0.5 ppm) suggesting a potential for COVID-19 prevention and treatment [62].
EGCG exhibits strong antiviral activity against the SARS-CoV-2 Omicron variant with greater tropism towards the upper respiratory tract, via activating Nrf2, which upregulates HO-1, and via increasing expression of IFN-β and retinoic acid-inducible gene I (RIG-I) [63]. Evidence of antiviral effects against Wuhan type of SARS-CoV-2, β-CoV, and δ-CoV types following treatment with sorbitol/lecithin-based throat spray containing concentrated green tea extract rich in EGCG content has been shown in the Vero E6 cell culture model. It suggests EGCG could be useful in the formulation of mouthwash for COVID-19-infected patients for decreasing infectivity in oral mucosa [64].
EGCG inhibits neutrophil extracellular traps (NETs) release or activity or reduces the levels of D-dimers suggesting a potential therapeutic strategy in the treatment of thrombotic and thromboembolic complications in severe COVID-19 infection.
EGCG has been found to downregulate the expression of TGF-1β and type 1 collagen accumulation, while also reducing NF-κβ activity. Additionally, it has been observed to decrease the expression levels of TNF-α and IL-1β, and restored glutathione S-transferase (GST), and nicotinamide adenine dinucleotide (phosphate) reduced:quinone oxidoreductase (NQO1) activity in lung tissues [65, 66]. These findings strongly suggest that EGCG is a highly effective preventative measure against lung fibrosis in COVID-19 infection.
Luteolin
Luteolin (3,4,5,7-tetrahydroxy flavone) is a natural flavonoid that exerts diverse pharmacological activities such as anti-cancer, anti-inflammatory, and neuroprotective activities. This molecule has been evaluated for finding ways to mitigate different clinical manifestations of COVID-19, such as AKI, neuroinflammation, long COVID-related complications, etc.
A study revealed that treatment with luteolin formulation in olive pomace oil (NeuroProtek®) can benefit patients with long-COVID syndrome suffering from brain fog and chemofog. This phytosomal formulation could mitigate neuroinflammation by downregulating the expression of pro-inflammatory mediators such as histamine, tryptase, chemokines [chemokine (C-C motif) ligand (CCL) 2, chemokine (C-X-C motif) ligand (CXCL) 8], and cytokines (IL-6, IL-1β, TNF) released by activated mast cells and microglia in the hypothalamus [67]. Patients suffering from cognitive difficulties and fatigue after mild COVID-19 show impaired cortical gamma-aminobutyric acid type B receptor (GABAB)-ergic activity. In RCT with 39 COVID-19 patients co-ultramicronized palmitoylethanolamide/luteolin (PEA-LUT) oral administration for 8 weeks enhanced GABAB-ergic transmission in the treatment group compared to the placebo [68]. Different formulations containing PEA-LUT have been tested in several clinical studies assessing their therapeutic potential in COVID-19-associated cognitive and olfactory dysfunction. These formulations have markedly improved and ameliorated olfactory recovery and mental clouding in patients, respectively [69–71].
Luteolin was identified as a renoprotective agent against COVID-19-associated AKI as indicated by a decrease in blood urea nitrogen (BUN) and serum creatinine levels, inflammatory mediators such as IL-1β, IL-6, and TNF-α, oxidative stress, and morphological damages [72]. Perilla frutescens seed meal (PFEA) containing rosmarinic acid (388.70 ± 11.12 mg/g extract) and luteolin (248.82 ± 12.34 mg/g extract) has been reported to reduce lung cell inflammation by inhibiting S protein S1-induced inflammatory response via the suppression of the JAK1/STAT3-inflammasome-dependent inflammatory pathway through downregulation of IL-6, IL-1β, IL-18, and NLRP3 genes. The result suggests that luteolin can be developed as a potential therapeutic candidate for the prevention of inflammation-related post-acute sequelae of long COVID-19 [73].
Myricetin
Myricetin is a polyphenolic compound widely reported to exert anti-inflammation, anti-cancer, and anti-oxidation effects. Myricetin’s immunomodulatory functions have been examined in the context of COVID-19. Myricetin is reported to inhibit SARS-CoV-2 replication by targeting viral 3CLpro and also can reduce the expression levels of inflammatory factors such as IL-6, TNF-α, IFN-γ, and IL-1α in bleomycin-induced lung injury mice model [74]. It can interfere with SARS-CoV-2 replication in Vero E6 cells with IC50 of 0.63 μmol/L by inhibiting the enzymatic activity of SARS-CoV-2 3CLpro [75].
Myricetin may attenuate TNF-α-stimulated inflammatory mediator generation in keratinocytes by mitigating the activation of the Akt, the mammalian target of rapamycin (mTOR), and NF-κβ pathways [76].
Hesperidin
Hesperidin is the most common citrus flavonoid belonging to the class of flavanones and is widely found in lemons, sweet oranges, and in few other fruits and vegetables. It has a wide range of antioxidant, anti-inflammatory, cardioprotective, antiatherogenic, and anti-hyperlipidemic activities. Hesperidin and its metabolite hesperetin are known to inhibit the release of inflammatory factors TNF-α, IL-1β, IL-6, and IL-12 and promote the secretion of anti-inflammatory factors like IL-4 and IL-10 by modulating c-Jun N-terminal kinase (JNK), NF-κβ, MAPK, and activator protein-1 (AP-1) signalling pathways [77, 78].
In vitro studies in Vero E6 cells have demonstrated that hesperetin and hesperidin hinder the interaction between the viral S protein and cellular receptor ACE2 and can even downregulate host ACE2 and TMPRSS2 expression. This hinders virus entry into the cells [79]. In a mouse model of inflammatory lung disease, nasal delivery of 10 mg/kg of the chitosan nanoparticles (NPs) loaded with hesperidin was found to significantly inhibit inflammatory cytokine levels such as IL-1β, IL-6, nitric oxide (NO), and reduced endothelial permeability compared with free hesperidin [80].
A randomized, double-blinded, placebo-controlled study concluded that the use of hesperidin (1,000 mg once daily) for 14 days in the treatment of 216 symptomatic non-vaccinated patients infected with SARS-CoV-2 showed a reduction of a composite of selected COVID-19 symptoms including fever, cough, shortness of breath, and anosmia [81].
Kaempferol
Kaempferol (3,4’,5,7-tetrahydroxyflavone) is a flavonoid that widely exists in several fruits and vegetables such as tea, broccoli, cabbage, kale, beans, endive, leek, tomato, strawberries, and grapes. It has a wide range of activities such as anti-inflammatory, antiviral, anti-cancer, and anti-bacterial properties. Moreover, kaempferol downregulates pro-inflammatory cytokines which are responsible for the onset of cytokine storm.
In vitro assays from different laboratories have indicated the anti-SARS-CoV-2 potential of kaempferol via the inhibition of viral 3CLpro protein and RdRp of its replication machinery. It was also reported that it can block the internalization of the virus into the host cell by binding to the ACE2-S complex [82]. In vitro analysis of kaempferol by pseudovirion assay showed a marked decrease in the lentiviral particles in transfected HEK293T-hACE2 cells [83].
Both in vivo (BALB/c mice) and in vitro (MH-S cells) studies have proved that kaempferol can mitigate H9N2 influenza virus-induced inflammation and acute lung injury via the suppression of toll-like receptor (TLR) 4/myeloid differentiation primary-response protein 88 (MyD88)-mediated NF-κβ and MAPK signalling pathways [84]. Kaempferol has been characterized in pulmonary fibrosis (PF) models to prevent the extent of lung injury by targeting IL-17, TNF, HIF-1, epidermal growth factor receptor (EGFR), phosphoinositide 3-kinase (PI3K)/Akt, TLR, and protease-activated receptor-1 signalling pathways. Since COVID-19 and PF share common targeting pathways, kaempferol is hypothesized to be effective against COVID-19/PF co-occurrence by modulating these targets [85, 86].
Kaempferol’s ability to inhibit NLRP1/NLRP3 inflammasome formation, reduce oxidative stress-induced apoptosis, and regulate autophagy makes it an interesting target to utilize against COVID-19 and its ophthalmic manifestation of the retinal degenerative disease [87].
However, in order to confirm the antiviral effect of this component, it is necessary to conduct more in-depth studies since kaempferol has a low bioavailability.
Non-flavonoid
Curcumin
Curcumin is the principal curcuminoid extracted from turmeric (Curcuma longa) exhibiting a wide range of pharmacological activities including anti-inflammatory, anti-cancer, antioxidant, wound healing, and antimicrobial effects.
Initial in silico studies have pointed out that curcumin can bind with several SARS-CoV-2 targets such as 3CLpro active sites, RBD domain of S protein, and RdRp-RNA complex active site. All of these can potentially inhibit viral entry or/and replication [88–90].
Curcumin-treated peripheral blood mononuclear cells (PBMCs) stimulated with SARS-CoV-2 have shown a reduction in the production and release of pro-inflammatory cytokines, including IL-1β, IL-6, MCP-1, and IL-8. An in vitro screening of curcumin against SARS-CoV-2 infected Vero E6 cells demonstrated strong inhibition against the D614G strain of SARS-CoV-2 through different treatment strategies like pre-post-infection treatment, pre-infection treatment, post-infection treatment, and co-treatment with EC50 values of 4.06, 5.02, 6.03, and 3.57 µg/mL respectively. The antiviral effect of a non-cytotoxic concentration of curcumin (10 µg/mL) was found to be effective against the SARS-CoV-2 Delta variant using pre-post-infection treatment and co-treatment with EC50 values of 1.14 and 1.66 µg/mL respectively [91]. Aqueous turmeric root extract, dissolved turmeric supplement capsules, as well as pure curcumin could effectively inhibit SARS-CoV-2 infection in Vero E6 and human Calu-3 lung cells at low subtoxic concentrations [92]. Curcumin-based carbon quantum dots (Cur-CQDs) show promise in enhancing the antiviral effects of curcumin against viruses like enterovirus 71 (EV71) and could be explored for future studies against HCoV [93].
In a clinical case study, the compassionate use of two capsules of curcumin-piperine for 14 days in 46 outpatients with COVID-19 disease could significantly reduce weakness. More systematic and controlled clinical studies with a larger sample size and higher dose and duration will be needed in the future to find out the use of curcumin as an adjunct therapy in COVID-19 patients [94]. Use of oral nano-curcumin capsules (at 160 mg of nano-curcumin in four 40 mg capsules daily for 14 days) by 20 patients with severe COVID-19, was found to be able to reduce the mRNA expression level of pro-inflammatory cytokines, including IL-1β and IL-6 significantly [95].
Some clinical trials assessing the efficacy of using curcumin combined with other substances in clinical improvement are under investigation. The therapeutic efficacy and safety of a nanoparticular combination comprising artemisinin, curcumin, Boswellia, and vitamin C are under exploration in patients diagnosed with moderate COVID-19 in a Phase III, double-blind, controlled clinical study [96]. A clinical study has investigated the therapeutic effect of curcumin/Boswellia serrata/ascorbic acid mixture supplementation in patients with post-acute COVID-19 syndrome [97].
Resveratrol
Resveratrol is a plant stilbenoid found abundantly in grapes, red wine, mulberry, and peanuts, possessing antioxidant, antitumor, antiviral, and free radical scavenging properties. Being both an antiviral and anti-inflammatory agent, resveratrol could be a novel therapeutic drug for the treatment of COVID-19 patients.
Resveratrol demonstrated the ability to effectively suppress SARS-CoV-2 replication in vitro with an EC50 value of 4.6 µmol/L, CC50 value of 210 µmol/L, and a selectivity index of 45.65 in medical research council cell strain 5 (MRC5) [98]. In a study, both resveratrol and its metabolically more stable structural analogue, pterostilbene, showed significant antiviral effects by inhibiting SARS-CoV-2 replication for up to 48 h post-infection in the air-liquid interface cultured human primary bronchial epithelial cells, isolated from healthy volunteer [99].
Resveratrol as an adjunctive therapy can mitigate age-related dysregulation of immune response to SARS-CoV-2 infection in elderly patients by the possible mechanism of enhancing Nrf2 signalling; suppressing IL-18, IL-1β, IL-6, and TNF-α expression and attenuating the activity of caspase-3/9 [100]. According to a study, treating peripheral blood samples from COVID-19 patients ex vivo with resveratrol resulted in reduced levels of matrix metalloproteinase (MMP)-9 and IL-8 secretion and NET generation by neutrophils [101].
Resveratrol is being evaluated in combination with other substances for adjunct therapy in various clinical trials. A randomized phase 2 double-blind placebo-controlled proof-of-concept involving treatment with resveratrol and adjunct vitamin D3 in 105 outpatients with mild COVID-19 for 6 months mitigated the effects of COVID-19 by lowering the incidence of pneumonia, hospitalization, and COVID-related ER visits [102]. A retrospective observational study has reported a shortening of recovery time and early resolution of COVID-19-associated symptoms including fever, dry cough, dyspnoea, headache, diarrhoea, and weakness disease in COVID-19-positive patients treated with adjuvant Imuno TF® with a combination of nutraceuticals including resveratrol and other anti-inflammatory natural compounds [103].
In a study, the use of resveratrol-zinc NPs as adjuvant therapy is under investigation among patients with mild COVID-19 to prevent the progression from moderate to severe stage [104].
The safety and efficacy of resveratrol capsule with placebo as an anti-fibrotic therapeutic approach is under investigation in discharged patients recovering from COVID-19 [105].
Pterostilbene
Pterostilbene (trans-3,5-dimethoxy-4-hydroxystilbene) is a stilbenoid, natural dimethylated analogue of resveratrol, widely found in almonds, blueberries, grape leaves and vines, and Pterocarpus marsupium heartwood.
Pterostilbene was found to directly interfere with viral replication and markedly inhibit SARS-CoV-2 in Vero E6 cells and in human primary bronchial epithelial cells [99]. In LPS-induced lung inflammation and melanoma metastasis models, pterostilbene was reported to suppress the expression of TNF-α and IL-6 levels by inhibiting the activity of neutrophil elastase (NE) and expression of thrombospondin-1 (TSP-1) [106]. Pterostilbene significantly attenuated fumonisin B1 (FB1)-induced cytotoxicity via downregulation of JAK2 and STAT3 genes of the JAK/STAT signalling pathway and proved to be a promising candidate with good anti-inflammatory, antioxidant, and cell regeneration effects [107]. It may be further evaluated to assess its potential to ameliorate COVID-19-related excessive inflammation.
Phillyrin
Phillyrin is an effective lignan glycoside extracted from traditional Chinese medicine (TCM) Forsythia suspensa (Thunb.) exhibiting anti-inflammatory, antioxidant, and antiviral activities.
Molecular docking studies revealed that phillyrin can be used as a potential inhibitors of COVID-19 by blocking the interaction between S protein and ACE2 [108].
An in vitro study demonstrated that phillyrin inhibited replication of SARS-CoV-2 in Vero E6 cells with IC50 of 63.90 μg/mL by reducing the expression level of pro-inflammatory cytokines (TNF-α, IL-6, IL-1β, MCP-1, and IP-10) [109]. Phillyrin exhibited significant downregulation of pro-inflammatory cytokines such as IL-6, TNF-α, IL-1β, IP-10, and MCP-1 in a dose-dependent manner in human hepatocellular carcinoma cell lines (Huh-7) cells infected with SARS-CoV-2 and HCoV-229E. This study also showed that phillyrin markedly inhibited the NF-κβ signalling pathway via inhibition of NF-κβ p65, p-NF-κβ p65, p-inhibitory kappa βα (Iκβα), and upregulation of Iκβα protein expression levels [110]. Another investigation found phillyrin to considerably reduce inflammation in LPS-stimulated RAW264.7 macrophages via inhibition of JAK/STAT and p38 MAPK signal pathways and to reduce ROS levels [111]. Phillyrin treatment markedly reduced the level of TNF-α, IL-1β, IL-6, and myeloperoxidase in an acute lung-injury mice model, thereby reducing lung inflammation, alveolar haemorrhage, and neutrophil infiltration [112].
A study revealed that phillyrin may mainly act on the HIF-1 signalling pathway, PI3K/Akt signalling pathway, and rat sarcoma (RAS) signalling pathway to alleviate hypoxia-cytokine storm mediated by chronic inflammatory diseases such as COVID-19 and influenza. It may be a potential therapeutic candidate to treat COVID-19 and influenza co-infection [113].
Tannins
Tannins are a class of polyphenolic compounds having antiviral, anticarcinogenic, and antimutagenic potentials that are found in many plant foods.
Docking-based assays reported that hydrolysable tannins, including ellagitannins (punicalagin and ellagic acid) and gallotannins (tannic acid, pentagalloyl glucose, ginnalin A, and gallic acid), and their gut microbial metabolites, urolithins, and pyrogallol, respectively showed potent inhibition against SARS-CoV-2 3CLpro protein (by 6.6–100.0% at 50 μmol/L) [114]. Studies based on enzymatic assay revealed that tannic acid effectively inhibited TMPRSS2 (IC50 = 50 μmol/L) and 3CLpro (IC50 = 1 μmol/L), potentially blocking the SARS-CoV-2 activity [115].
Corilagin was found to effectively inhibit the binding between S RBD peptide and ACE2 receptor with IC50 of 24.9 μmol/L and also reduced RBD-pseudotyped lentivirus infection in hACE2 overexpressing HEK293 cells, which mimicked the entry of SARS-CoV-2 virus in human host cell [116]. Geraniin extracted from Elaeocarpus sylvestris was reported to prevent the interaction between S protein RBD and hACE2 receptor in competitive enzyme-linked immunosorbent assay thereby suggesting it might inhibit the entry of SARS-CoV-2 [117]. Punicalagin inhibits the 3CLpro in a dose-dependent manner with an IC50 of 6.192 μg/mL. Punicalagin in combination with zinc sulfate monohydrate (punicalagin/Zn-II) decreases the action of 3CLpro 4.4-fold in contrast to only punicalagin [118].
Persimmon (Diospyros kaki)-derived tannin dissolved in carboxymethyl cellulose inhibits the replicative ability of the Alpha variant of SARS-CoV-2 effectively in vitro in a dose-dependent manner. Oral treatment of persimmon-derived tannins to uninfected hamsters lowered the severity of pneumonia by suppressing pro-inflammatory cytokines such as IL-6, TNF-α, and IFN-γ as well as transmission of the SARS-CoV-2 virus [119]. Oral intake of tannins (extracted from quebracho and chestnut) in addition to vitamin B12 and standard treatment reduced MIP-1α and TNF-α levels in hospitalized COVID-19 patients with diarrhoea and pneumonia complications [120].
The randomized placebo-controlled trial was conducted to investigate the efficacy of oral administration of the dietary supplement ARBOX (combination of quebracho and chestnut tannins extract and vitamin B12), 2 capsules per day of tannin for the 14 days on the restoration of gut microbiota function in the treatment of hospitalized COVID-19 patients. These tannin-based dietary supplementations showed a significant reduction of MIP-1α level i.e., correlated with microbiota composition modulation, although no considerable clinical improvement or significant gut microbiota shifts after 14 days were noticed [121].
Alkaloids
Alkaloids in plants are derived from nitrogen-containing bases. Traditionally used alkaloids possess several pharmacological effects like anti-bacterial, anti-malarial, anti-inflammatory, anti-cancer, anti-viral, etc. Classification is not uniform for alkaloids. Mostly alkaloids are derived from higher plants like Berberis sp., Stephania sp., Colchicum sp., etc. Alkaloids which are mentioned in this review have their anti-SARS-CoV-2 properties evaluated in experimental studies and belong to different subclasses like isoquinolines, benzylisoquinoline, proto-alkaloid, cytotoxic alkaloid, etc. Notably, all these alkaloids are FDA-approved.
Berbamine
Berbamine, an isoquinoline, and a bisbenzylisoquinoline (BBIQ) alkaloid, is a TCM which can be derived from different parts of barberry plants (Berberis poiretii, Berberis integerrima, etc.) of Berberidaceae family. Berbamine exhibits antiviral [JEV, bovine viral diarrhoea viruses (BVDV), African swine fever virus (ASFV), SARS-CoV-2, MERS-CoV, etc.] [122], immunomodulatory, anti-inflammatory effects, and cardiovascular effects [123].
Several in vitro and in vivo studies have demonstrated the efficacy of berbamine in controlling SARS-CoV-2 entry and infection. In hACE2 overexpressed HEK293T cells, treatment with berbamine at a dose of 10 μmol/L suppresses SARS-CoV-2 S pseudotyped particle entry by decreasing cell surface expression of ACE2 or dipeptidyl peptidase-4 (DPP4). The reduced cell surface expression of ACE2 is attributed to berbamine modulating transient receptor potential mucolipin (TRPML) channels activity, which are calcium (Ca2+) permeable channels of lysosomes [124]. Treatment with berbamine hydrochloride can diminish viral N protein expression levels in SARS-CoV-2-infected Vero E6 and Caco2 cells in a dose-dependent manner (EC50 values = 1.732 and 1.887 μmol/L respectively). In a coculture of SARS-CoV-2 S protein expressing baby hamster kidney (BHK) fibroblasts cell (effector cell) and Vero E6 cells (target cells), berbamine hydrochloride treatment could prevent S protein-mediated cell-cell fusion [125].
Berberine
Berberine is a quaternary ammonia compound that belongs to the benzylisoquinoline alkaloids group and is extracted from root, bark, shoots, and rhizomes of different plants such as barberry (Berberis vulgaris), Oregon grape (Mahonia aquifolium), goldenseal (Hydrastis mcanadensis), and yellowroot (Xanthorhiza simplicissima).
Berberine was reported to block SARS-CoV-2 replication and suppress ACE2 and TMPRSS2 gene expression in human epithelial cells (Calu-3). A newly developed orally available immunotherapeutic-berberine nanomedicine, named NIT-X, could mitigate COVID-19 by suppressing hyperactive mast cells and exerting immunomodulatory effects to suppress pro-inflammatory cytokines [126]. The treatment of berberine on human nasal epithelial cells infected with SARS-CoV-2 (BavPat1 isolate) inhibited viral replication in cells with an EC50 value of 10.7 µmol/L [127].
As cytokine storm is a great concern in COVID-19, there are reports of the efficacy of berberine in the suppression of hyper-cytokinemia and inhibition of hyper-inflammation in different disease models. For example, an in vitro study by Li et al. [128] on LPS-treated RAW264.7 cell found berberine at a dose of 1.25 μmol/L to remarkably lower the pro-inflammatory cytokine and markers [TNF-α, IL-1β, IL-6, prostaglandin E2 (PGE2), NO], inhibit the mRNA expression of COX-2 and iNOS and inhibit NF-κβ p65 and Iκβα phosphorylation. Additionally, in phorbol 12-myristate 13-acetate (PMA)-induced macrophages, berberine was shown to attenuate NLRP3 inflammasome expression by inhibiting the activation of the TLR4/Myd88/NF-κβ signalling pathway in PMA-induced macrophages [129].
In vivo studies in dextran sodium sulfate (DSS)-induces colitis model of C57BL/6 mice, berberine administered in 50 mg/kg dose lowered pro-inflammatory cytokines and chemokines (TNF-α, IFN-γ, IL-1β, IL-5, IL-22, IL-17A, IL-13, IL-23, CCL2, CCL3, CCL4, CCL17, CCL20, CXCL9, CXCL10, CXCL11) by modulating and inhibiting JAK1/2, STAT1/3/4/5/6, ERK/MAPK, Akt pathways [130]. Oral treatment with berberine for 2 weeks on male C57BLKS/J mice downregulated the expression level of the thioredoxin-interacting protein (TXNIP), NLRP3, and TNF-α [131]. It remains to be seen if berberine can have a beneficial role in protecting COVID-19 patients from cytokine storm.
A randomized controlled clinical trial was carried out to assess the efficacy of berberine on alleviating gastrointestinal symptoms including changes in diarrhoea frequency and secondarily modifying serum concentrations of the inflammatory biomarkers, and organ function in severe patients with SARS-CoV-2 infection [132]. Another ongoing clinical trial is looking into the efficacy and safety of COVIDEX (herbal formulation containing berberine) in patients infected with SARS-CoV-2. The aim of this study is to examine whether this treatment regimen in patients decreases SARS-CoV-2 viral load in the nasopharynx, and shortens clinical recovery and disease severity [133].
Cepharanthine
Cepharanthine, a BBIQ alkaloid, isolated from Stephania cepharantha Hayata (family Menispermaceae), shows anti-inflammatory, antioxidative, immunomodulating, antiparasitic, and antiviral properties.
Transcriptomic analysis of Vero E6 cells infected with SARS-CoV-2-related coronavirus (SARS-CoV-2r GX_P2V from Guangxi pangolins) noticed that the virus upregulated about 619 genes (e.g., HSPH1, CALR, HSPA8, HSPH1, CALR, DNAJB1, DNAJA3, DNAJA4). Cepharanthine (6.25 μmol/L dose) treatment can remarkably downregulate 374 of these genes. The effect of virus infection on the fat metabolism pathway and autophagy-associated gene expression was also reversed by cepharanthine [134]. In a similar study with the same cell line, cepharanthine (10 μmol/L dose) could lower the viral RNA load up to 15,393-fold in comparison to a nontreated one [135]. Cepharanthine could target the NSP13 enzyme, affecting the Ca2+ channel of the host and increasing the cholesterol level of the cell. In doing so, it blocked the S protein and ACE2-mediated virus-cell fusion and viral entry [136, 137].
Combination therapy with nelfinavir (NFV) and cepharanthine is validated to downregulate SARS-CoV-2 infection by reducing N protein expression in an in vitro assay. This study found that cepharanthine could inhibit the attachment of the SARS-CoV-2 virus to the Vero E6/TMPRSS2 cells and block the entry of the virus. Also, after virus entry into the cell, the co-treatment of NFV and cepharanthine reduced the viral RNA load in infected cells. Mathematical modelling of combination treatment of NFV (oral administration) and cepharanthine (intravenous injection) is predicted to downregulate the viral RNA load 6.15 days faster than the untreated group and 1.23 days earlier than NFV treatment alone [138]. There is an ongoing randomized phase 2, double-blind clinical trial to assess the efficacy and safety of orally administered cepharanthine in non-hospitalized asymptomatic or mild adult participants with COVID-19 to reduce viral clearance time and SARS-CoV-2 viral load [139].
Cepharanthine may be an interesting candidate to evaluate in COVID-19-related lung injury studies as it has been demonstrated to ameliorate PF in animal models and reduce inflammation by blocking the NF-κβ/NLRP3 pathway [140].
Colchicine
Colchicine is a type of proto-alkaloid, derived from the Colchicum autumnale plant (common name autumn corcus). It is an FDA-approved drug mainly used as anti-gout medicine as well as to prevent familial Mediterranean fever and Behcet’s syndrome.
Colchicine possesses anti-inflammatory properties by downregulating the level of pro-inflammatory substances like IL-6, IL-1, the nucleotide-binding and oligomerization domain (NOD), granulocyte-macrophage colony-stimulating factor (GM-CSF) [141]. Colchicine also inhibits IL-1β /IL-18 response by suppressing mitochondrial Ca2+ uniporter (MCU)-induced NLRP3 inflammasome and reduces oxidative stress by lowering Ca2+ influx inside neutrophils [142].
Clinical studies with colchicine have revealed many benefits against COVID-19. Colchicine administration in moderate to severe COVID-19 patients showed less mortality and a higher discharge rate in comparison to the control [143]. A pilot RCT based on 40 adults with obesity and metabolic syndrome reported that a dosage of 0.6 mg colchicine twice daily for 3 months reduced pro-inflammatory molecules including CRP, IL-6, resistin, along with vascular-related proteins (such as oxidized low-density lipoprotein receptor, phosphodiesterase 5A) [144]. Another clinical trial assessed combination oral therapy of prednisone (60 mg per day for 3 days) and colchicine (1–1.5 mg per day for 3 days and then 0.5 mg per day for 11 days) on 25 patients and 29 patients were chosen as control. This therapy enhanced the survival rate in elderly COVID-19 patients who required oxygen therapy over a 28-day period. Patients in this treatment required lower glucocorticoid which decreased the hospitalization rate and also downregulated ferritin and D-dimer [145]. A randomized clinical trial based on colchicine administration with standard care for as long as 3 weeks in patients hospitalized with COVID-19 had shown significant improvement in time to clinical deterioration, although no significant differences in high-sensitivity cardiac troponin or CRP levels were noticed [146]. During a therapeutic study on moderate to severe COVID-19 patients equally divided into both colchicine and placebo groups, colchicine showed a beneficial effect of the treatment. Colchicine reduced the median time required for oxygen supplementation (colchicine: 4.0 days; placebo: 6.5 days) and hospitalization (colchicine: 7.0 days; placebo: 9.0 days). On the second day, 67% of patients in the colchicine group and 86% of patients in the placebo group required oxygen support. By day 7, the need for oxygen support decreased to 9% in the colchicine group and 42% in the placebo group. However, this study did not confirm whether colchicine decreases mortality or not. As a side effect, colchicine-treated patients had higher frequency of diarrhoea [147].
An RCT was carried out among COVID-19 patients with mild, moderate, or severe pneumonia, orally treated with colchicine plus herbal phenolic monoterpene fractions along with standard treatment. It found changes in mortality rate, length of hospitalization, lymphocyte count, and serum LDH level among experimentally treated group [148]. Importantly, there are several clinical trials being carried out on colchicine—NCT05246072 (involving combined treatment of ivermectin and colchicine along with standard care in COVID-19 patients), NCT04756128 [involving colchicine and low-dose naltrexone (LDN)-relative to standard of care in patients hospitalized with moderate COVID-19] [149, 150]. Data from different clinical trials may help us to better understand the scope of colchicine treatment for deploying during coronavirus disease outbreaks in the future.
Emetine
Emetine is a pyridoisoquinoline and an isoquinoline alkaloid, derived from Uragoga ipecacuanha root. Emetine is an FDA-approved anti-protozoal drug and is used for amoebiasis treatment.
Emetine has known anti-viral properties against several viruses like ZIKV, Ebola virus, bovine herpesvirus 1, herpes simplex virus (HSV)-2, MERS-CoV, SARS-CoV, HCoV-OC43, etc. Emetine was reported to inhibit SARS-CoV-2 virus replication at 0.5 μmol/L dose. An in vitro study demonstrated that a combination therapy of remdesivir and emetine could inhibit 64.9% of viral yield [151]. Emetine was found to possess the lowest EC50 value among 294 screened agents with EC50 0.054 μmol/L and 0.014 μmol/L for SARS-CoV and MERS-CoV respectively which was 500–1,900 times lower than IC50 of Entamoeba histolytica (IC50 = 26.8 μmol/L). The data showed that emetine was more effective as an anti-coronavirus agent in comparison to amoebiasis. Due to the highly potent drug, intramuscularly 0.1–0.2 mg/kg (max 6–12 mg/day) of emetine is enough dose for coronavirus treatment [152]. In Vero cells, emetine could block viral protein translation by stopping the binding of SARS-CoV-2 mRNA with eukaryotic initiation factor 4E (eIF4E). Thus, reducing viral load in low nanomolar doses (EC50 = 0.147 nmol/L) [153].
In another in vitro study on SARS-CoV-2 virus-infected Vero E6 cells, emetine inhibited viral replication in a very low dose with EC50 of 0.007 μmol/L which is 30-fold more efficient in comparison to remdesivir treatment alone. Emetine (0.01 μmol/L) efficiently downregulated LPS-induced IL-6 expression level in M1-polarized THP-1 macrophages. Pharmacokinetic evidence showed that emetine provided faster drug accumulation in the lung (Tmax: 0.03 h) and kidney (Tmax: 0.5 h) compared to the liver (Tmax: 9 h) when administered by intravascular injection in mice. This phenomenon indicates the suitability of emetine for treating respiratory diseases [154].
Clinical efficacy of emetine against COVID-19 was shown in a therapeutic study employing low-dose emetine administration (for 10 days in 3.6 mg per os dose, 3 times per day) with other routine antiviral therapy on hospitalized patients infected with SARS-CoV-2. The low-dose treatment improved oxygen saturation in the blood and brought relief in breathing difficulties. It was found to decrease the infection level in severe conditions and inhibit the transition of mild to moderate infection into severe cases [155].
Homoharringtonine
Homoharringtonine, extracted from Cephalotaxus harringtonia, is a cytotoxic alkaloid and FDA-approved drug for chronic myeloid leukemia (CML) treatment and has been used in China for about 50 years [156].
Homoharringtonine shows antiviral activities in different doses against viruses like vesicular stomatitis virus (VSV), Newcastle disease virus (NDV), porcine epidemic diarrhoea virus (PEDV), etc. in both cell line and animal models. Homoharringtonine was known to inhibit mouse hepatitis coronavirus (MHV) translation in vitro in 125 nmol/L dose. eIF4E phosphorylation promotes replication and translation of MHV which is downregulated by homoharringtonine [157]. In another study with PEDV in Vero E6 cell line, homoharringtonine at doses of 150 nmol/L and 300 nmol/L reduced viral titer by 3.5-fold and 40-fold, respectively, in the 50% tissue culture infectious dose (TCID50) [158]. Homoharringtonine inhibited translation by binding with 80S ribosomes and stopping the elongation of the peptide chain in the treatment of myelogenous leukemia. This property marked that homoharringtonine could act as an anti-translation agent for various viruses and block viral replication [159].
In in vitro study on Vero cells, homoharringtonine exhibited high efficacy against SARS-CoV-2 with an average EC50/IC50 value of less than 300 nmol/L and IC50 value of 0.16 μmol/L. In MRC5 cells, for HCoV-229E infection, homoharringtonine showed its efficacy with an EC50 value (0.05106 μmol/L) 4 times lower than the CC50 value (0.2046 μmol/L) [160].
In another study, homoharringtonine (HY-14944) showed anti-SARS-CoV-2 activity on the Vero E6 cell line in nanomolar concentration with tolerable cytotoxicity (IC50 = 165.7 nmol/L and CC50 = 1,110 nmol/L). homoharringtonine also induced IFN-β responsive host gene activity in primary normal human bronchial epithelial (NHBE) cells [161].
In vitro studies have provided evidence towards homoharringtonine’s ability in preventing virus entry into the cell by lowering the expression of TMPRSS2 on the cell surface of Vero E6, Caco-2 (intestinal epithelial cell line), and Calu-3 (human lung cancer cell line) cell lines [162]. When nebulization with homoharringtonine is carried out, its concentration in the respiratory tract is noticeable and may make it more convenient for treating respiratory virus attacks [163].
Lycorine
Lycorine is an indolizidine alkaloid which is derived from plants of the Amaryllidaceae family, for example, Crinum asiaticum, Clivia miniata (Bush Lily), Lycoris squamigera (Surprise Lily), etc. Although it may be toxic in certain quantities, lycorine exhibits anti-malarial activity. This Chinese medicinal herb also has anti-leukemia, anti-parasitic, anti-tumor, anti-bacterial, anti-inflammation as well as anti-viral properties with low toxicity and minimal side effects [164].
Lycorine was demonstrated to inhibit SARS-CoV-2 infection in Vero-E6 cells by binding with frameshift stimulation element (FSE) and blocking propagation of SARS-CoV-2. It interfered with the growth site of functional RNA and blocked SARS-CoV-2 transcription to inhibit virion core assembly in a dose-dependent manner [165]. A dose-dependent administration of lycorine on virus-infected Vero E6 cells showed viral inhibition by lycorine with an EC50 of 0.31 μmol/L [166]. An in-cell-protease assay demonstrated inhibition of SARS-CoV-2 viral activity due to lycorine derivative, lycorine hydrochloride targeting 3CLpro of SARS-CoV-2. In infected Huh-7.5 cells, 3CLpro inhibitor lycorine hydrochloride lowered the virus spread by 88% with an EC50 value of 0.01 μmol/L [167].
By a cell-based reporter assay, an in vitro analysis demonstrated that lycorine could directly inhibit SARS-CoV-2 viral replication by using RdRp as a target and inhibit this enzyme by forming hydrogen bonds with RdRp at Asp623, Asn691, and Ser759 position, with a similar binding pocket like remdesivir. In this study lycorine-treated infected Vero cells had reduced viral activity reporting the IC50 value of 0.878 μmol/L and strain index (SI) value of > 56.95 [168].
Quinine
Quinine is an effective blood schizontocidal antimalarial agent that is a 4-methylquinoline alkaloid and is derived from the bark of Cinchona species. It is the most potent and oldest natural drug against Plasmodium falciparum infection.
Quinine as well as its different chemical derivatives such as chloroquine (CQ), hydroxychloroquine (HCQ), and quinine sulfate (QS) have shown potential anti-SARS-CoV-2 activity. In Vero B4 cells quinine performed as more potent in diminishing SARS-CoV-2 compared to HCQ and QS and with better cytotoxicity profile. The effectiveness of quinine was also confirmed in human Caco-2 colon carcinoma-derived epithelial cells, in human transgenic lung cancer cells, A549 (modified to stably express either the receptor ACE2 or both ACE2 and TMPRSS2), human lung Calu-3 cells (expressing ACE2 and TMPRSS2 endogenously), Vero E6 and Vero B6 cells [169, 170].
At the start of the pandemic after the first report of in vitro inhibition of SARS-CoV-2 by quinine derivative HCQ [171], numerous (approximate 80) clinical trials were set up and fast-tracked to evaluate HCQ and CQ for the treatment and prevention of COVID-19. But therapeutic efficacy of quinine and its derivatives remain inconclusive following the clinical trials.
QS possesses antipyretic, anti-viral, and anti-inflammatory properties. Quinine increases the production of anti-inflammatory substance IFN-α cytokine [172, 173]. Quinine shows an important role in cytokine storm control by reducing the level of pro-inflammatory factors like, IL-1, IL-2, IL-6, IL-18, TNF-α, IFN-γ, chemokines CCL2 and CXCL10, and T helper-17 (Th17)-related cytokines and it also inhibits microRNA activity [174].
In in silico study demonstrated that HCQ blocked ACE2 terminal phosphorylation and upregulated endosomal pH. Quinine, CQ, and HCQ inhibited SARS-CoV-2 entry in the cell by binding with Lys353 amino acid in the peptide region of the ACE2 receptor [175].
Terpenes
Terpenes are the largest plant-derived organic hydrocarbon compound or phytochemical group, which is extremely used in India and China in the form of essential oils and provides aroma in the source plant. Currently, these phytochemicals are widely used in anti-cancer therapy. Different parts of plants (e.g., cinnamon, cloves, ginger, citral, menthol, camphor, salvinorin A, etc.), including flowers, bark, raisins, leaves, etc., are different sources of different terpenes. According to isoprene units (n), terpenes have different classes like hemiterpenes (n = 1), monoterpenes (n = 2), sesquiterpenes (n = 3), diterpenes (n = 4), sesterterpenoids (n = 5), triterpenes (n = 6), tetraterpenes (n = 8), and polyterpenes (n > 8). In this study, 9 terpenes have been selected for the discussion of their potential in SARS-CoV-2 treatment.
Andrographolide
Andrographolide is a natural diterpenoid derived from Andrographis paniculata possessing anti-inflammatory, anticancer, antibacterial, antipyretic, and analgesic [176] as well as antiviral properties.
A. paniculata extract and andrographolide were demonstrated to inhibit the production of infectious virions at the late phase in Calu-3 cells infected with SARS-CoV-2, reporting IC50 values of 0.036 μg/mL and 0.034 μmol/L, respectively, using the plaque reduction assay [177]. Shi et al. [178] also highlighted that andrographolide and its fluorescence derivative, the nitrobenzoxadiazole-conjugated andrographolide (Andro-NBD), could inhibit the activity of 2019-nCoV 3CLpro with IC50 of 15.05 μmol/L and 2.79 μmol/L respectively based on in vitro assay. Andrographolide was reported to bind with active site Cys145 of 2019-nCoV 3CLpro by covalent bond, as determined by mass spectrometry analysis.
Based on in vivo study, andrographolide treatment for LPS-induced acute lung injury was reported to inhibit the NF-κβ signalling pathway at the level of IkappaB kinase (IKK)-β activation [179]. The treatment of andrographolide [10 mg/kg intraperitoneal (i.p.) dose] on the C57BL/6 mice model was reported to improve lung condition, increase survival rate, and decrease cytokine level and viral load by modifying the NF-κβ and JAK/STAT signalling pathway [180]. Based on in vivo study, it had shown that the aqueous extract of A. paniculata downregulated the expression levels of pro-inflammatory molecules such as TNF-α, IL-1β and IL-6, ROS, and thiobarbituric acid reactive substancesthiobarbituric acid reactive substances (TBARS), and upregulated acetylcholinesterase (AchE) and butyrylcholinesterase (BchE) activity as well as reduced oxidative stress in LPS-induced rats [181].
Oral andrographolide administration at doses of 60 mg to adults with mild COVID-19 for 5 days had shown speedy SARS-CoV-2 clearance, less chances of pneumonia, and inflammation resolution [182]. A randomized, double-blind, placebo-controlled trial has been carried out to evaluate the safety and efficacy of A. paniculata extract containing andrographolide in the form of the capsule on adults with mildly symptomatic SARS-CoV-2 infection to check events of symptom severity, duration of illness symptoms, risks of pneumonia, and levels of inflammatory cytokines [183]. Xiyanping injection (main component is andrographolide) was reported to downregulate inflammation and reduce body temperature, cough, and lung infection when administered in COVID-19 treatment [184].
Tanshinone IIA
Tanshinone IIA (Dan Shen ketone or Tanshinone B) is a lipophilic abietane diterpene phytochemical derived from Salvia miltiorrhiza, possessing anti-cancer and anti-inflammatory properties.
In vitro study confirmed the result that tanshinone IIA acted as a potent anti-SARS-CoV-2 compound with IC50 of 4.08 μg/mL and had a virucidal activity of about 94% at 50 μg of concentration [185]. In SARS-CoV-2 treatment, tanshinone IIA sulfonate sodium, a derivative of tanshinone IIA, retained PLpro inhibitor activity with IC50 of less than 10 μmol/L [186].
In in vivo study tanshinone IIA-loaded nanoemulsion formulation was administered intratracheally in 30 µg/kg dose on albino Wistar rats with LPS-treated acute lung injury downregulation of TNF-α and IL-17 levels and upregulation of IL-10 level as well as elevation of lung oxidative stress markers including superoxide dismutase (SOD), glutathione peroxidase (GPx) [187].
Glycyrrhizin
Glycyrrhizin, also called glycyrrhizic acid (GA), is a triterpene glycoside, also known as saponins, extracted mainly from the Glycyrrhiza glabra (Ayurvedic name: Yashtimadhu) roots [188] and also from G. uralensis roots. Multiple studies have reported a wide range of pharmacological effects that glycyrrhizin possesses, including potent anti-inflammatory and antiviral effects, which have led to them being proposed as therapeutic compounds for the COVID-19 treatment.
The plaque reduction assay showed that glycyrrhizin was demonstrated to attenuate SARS-CoV virus replication (EC50 = 100 µg/mL) in Vero cells [189]. The 3-(4,5-dimethylthiazol-2-yl)-2,5-diphenyltetrazolium bromide (MTT) assay and immunocytochemical staining on Vero cells demonstrated potential inhibition of SARS-CoV activity of glycyrrhizin, and its 15 derivatives. Hoever et al. [190] evaluated that the addition of 2-acetamido-β-D-glucopyranosylamine to the glycoside chain of glycyrrhizin resulted in increased inhibition of SARS-CoV activity up to 10-fold when compared to glycyrrhizin. Glycyrrhizin blocked the viral replication by inhibiting the SARS‐CoV‐2 3CLpro proteins as well as targeting the S‐RBD‐ACE2 complex with EC50 of 0.44 μg/mL at 1 mg/mL (post-entry conditions) and 0.5 mg/mL (combined pre- and post-entry conditions) in Vero E6 cells [191]. Noticeably, in Vero cells by MTT cell-proliferative assay, glycyrrhizin exhibited inhibition on the replication of SARS-associated coronaviruses (SARS-CV, two clinical isolates FFM-1 and FFM-2 from SARS patients) with EC50 of 300 mg/L as well as prevented viral adsorption and penetration into the host cells [192].
An in vivo study reported that glycyrrhizin treatment significantly inhibited LPS-induced acute lung injury and suppressed the production of pro-inflammatory factors such as MCP-1, COX-2, and HMGB1 by the mechanism of upregulating ACE2 and inhibiting the caveolin-1/NF-κβ signalling pathway [193]. Zhu et al. [194] reported that glycyrrhizin prevented inflammation and fibrosis from bleomycin-induced pulmonary toxicity in mice by suppressing inflammatory signalling MAPK and fibrotic signalling Smad3 pathway.
Being a good candidate as an antiviral agent, glycyrrhizin has been investigated for clinical trials to cure COVID‐19 patients. A single-centre, randomized, double-blind, placebo-controlled clinical trial is being carried out on hospitalized patients with moderate COVID-19 infection which aims to evaluate the efficacy and safety of licorice extract containing glycyrrhizin along with Boswellia extract. This dietary supplement had a potent effect on lowering mortality rate, shortening recovery time, and enhancing prognosis as well as significantly increasing the serum CRP level and decreasing lymphocyte percentage [195]. Treatment with a mixture of diammonium glycyrrhizinate (glycyrrhizin ammonium and salt) and vitamin C on self‐quarantined patients evaluated significant resolution of patient’s symptoms [196]. A randomized, open, controlled trial upon the implementation of diammonium glycyrrhizinate enteric-coated capsules combined with vitamin C tablets is under investigation to treat novel coronavirus pneumonia (COVID-19) [197].
Saikosaponin
Saikosaponins are triterpene saponin glycosides extracted from different traditional oriental medicine plants, including Bupleurum spp., Heteromorpha spp., and Scrophularia scorodonia, possessing anti-inflammatory and antiviral properties.
There are different in vitro studies suggesting that saikosaponins may play an important role as a potential antiviral compound for the treatment of COVID-19. The 2,3-bis-(2-methoxy-4-nitro-5-sulfophenyl)-2H-tetrazolium-5-carboxanilide (XTT) cell-proliferative assay reported inhibition of saikosaponins A, B2, C, and D on cytopathic effects in MRC5 cells infected with HCoV-229E with reported EC50 values of 8.6, 1.7, 19.9, and 13.2 μmol/L respectively [198]. Another in vitro study revealed that saikosaponin B2 displayed better SARS-CoV inhibitory efficacy with the best SI value of 221.9 compared to other tested saikosaponins. Saikosaponin B2 was shown to prevent the early stage of viral replication by inhibiting the adsorption and penetration of the HCoV‐229E virus into host cells in a dose‐dependent manner [199].
Saikosaponin A was reported to show the inhibition of the expression of inflammatory mediators such as COX-2, ROS, and ILs (IL-6, IL-8, and TNF-α) in a dose‐dependent manner, thus repressing cytokine storm [200]. Saikosaponin D was shown to inhibit expression of pro-inflammatory cytokines including MIP-2, IL-6, and TNF-α and up-regulation of expression of anti-inflammatory mediators, such as TGF-β1 and IL-10. Saikosaponin D may attenuate ventilator-induced lung injury in rats via inhibition of inflammatory responses, oxidative stress, and apoptosis, as well as the infiltration of pulmonary neutrophils [201].
The potent antiviral activity as well as anti-inflammatory properties, highlight saikosaponins as a possible traditional medicine potential candidate for further clinical investigations in COVID-19 treatment.
Escin
Escin is a naturally found combination of saponins with anti-inflammatory, vasoconstrictor, and vaso-protective effects, extracted from horse chestnut seeds (Aesculus hippocastanum).
Wu et al. [202] demonstrated that escin significantly inhibited SARS-CoV (H.K. strain) replication in Vero E6 cell with EC50 of 6 μmol/L.
Escin has been reported to downregulate the levels of pro-inflammatory cytokines (TNF-α, IL-1β, and IL-6), alleviate LPS-induced acute lung injury via upregulating expression of glucocorticoid receptors and stimulating endogenous antioxidant capacity thereby protecting from pulmonary inflammation [203]. Another in vivo study reported that escin attenuated immune cell infiltration and selectively modulated Nrf2/HO-1, TNF-α/JNK, and IL-22/STAT3 signalling pathways in concanavalin A-induced autoimmune hepatitis in adult male BALB/c mice [204].
Currently, there are some clinical trials under investigation with escin or sodium escinate as an anti-inflammatory or antiviral treatment in COVID-19 patients. The potent antiviral activity as well as anti-inflammatory properties, identify escin as an excellent candidate for further clinical investigations in COVID-19 treatment. Among them, a clinical trial [205] investigated the anti-inflammatory efficacy of the injectable sodium escinate in patients suffering from COVID-19 pneumonia. The anti-inflammatory efficacy was monitored by chest imaging (computed tomography) and measuring plasma CRP and IL-6 levels. Further, a comparison with standard treatment will be observed. A randomized, double-blind, parallel-controlled clinical trial is in progress to evaluate the safety and efficacy of escin or sodium escinate as an adjunctive treatment regimen for COVID-19 patients [206].
Zerumbone
Zerumbone (2,6,9,9-tetramethylcycloundeca-2,6,10-trien-1-one) is a sesquiterpenoid and the major active compound of Zingiber zerumbet Smith rhizome, which belongs to the Zingiberaceae family. This compound possesses anti-inflammatory, antipyretic, and antinociceptive properties, which have been proven by several in vivo and in vitro experiments.
LPS-stimulated RAW264.7 cells treated with zerumbone showed the downregulation of pro-inflammatory compounds like iNOS and COX-2 protein. Zerumbone has been reported to decrease COX-2 and MMP-13 expression in IL-1β-induced primary rat chondrocytes at different concentrations [207].
An in vitro study showed that in RAW264.7 mouse macrophages and adult mouse microglia, administration of zerumbone decreases LPS-induced lipocalin-2 expression. Moreover, the study displayed that supplementation of zerumbone significantly downregulated LPS-stimulated phagocytosis and H2O2, ROO•, and HO• production in microglial cells. Also, M1 polarization markers (IL-6, IL-1β, and TNF-α) and LPS-induced increased protein expression (CXCL-10 and CCL-2) in both cell models were decreased by zerumbone. The sesquiterpenoid upregulated adenosine monophosphate-activated protein kinase (AMPK) and Akt/glycogen synthase kinase-3 (GSK3) signalling pathways and mediated endogenous antioxidant [HO-1, glutamate-cysteine ligase modifier subunit (GCLM), glutamate-cysteine ligase catalytic subunit (GCLC), and NQO1] production and anti-inflammatory IL-10 expression in microglial cells, which promoted protective responses in inflammation [208].
An in vivo study on male ICR mice stated that, in LPS-stimulated acute lung injury treatment, zerumbone decreased lung edema in a dose-dependent manner beginning from 1 μmol/kg concentration. Zerumbone significantly downregulated the expression of pro-inflammatory cytokine (TNF-α and IL-6), iNOS, and COX-2, and inhibited NF-κβ activation and Akt phosphorylation [209]. These anti-inflammatory properties make zerumbone efficient for cytokine storm management during COVID-19. Further clinical and therapeutic studies are required to validate the efficacy of zerumbone.
Eugenol
Eugenol, a type of phenolic monoterpenoid, can be extracted primarily from the flower buds of cloves (Eugenia caryophyllata) belonging to the Myrtaceae family. It can also be found in other sources like holy basil or tulsi (Ocimum tenuiflorum), cinnamon, pepper, and nutmeg. It possesses antimicrobial, antioxidant, anti-inflammatory, and analgesic properties.
In vitro, influenza A virus (IAV) infection significantly upregulated malondialdehyde (MDA), NO, and ROS and downregulated glutathione, total SOD (T-SOD), glutathione reductase (GR), and catalase (CAT) levels which were reversed by eugenol (5 µg/mL) administration. In continuation, eugenol also downregulated ERK1/2, JNK1, p38 MAPK, and IKK/NF-κβ signal pathways [210]. In a study, chemiluminescence and in silico assay demonstrated that eugenol has capability to inhibit ACE2 and S1 protein binding of SARS-CoV-2. Eugenol can also inhibit the pseudo-SARS-CoV-2 entry in hACE2-expressing HEK293 cells. In vitro, on the A549 lung cell line, eugenol administration resulted in the suppression of S protein induced NF-κβ and pro-inflammatory cytokine (TNF-α, IL-1β, and IL-6) expression. In vivo experiment on recombinant SARS-CoV-2 S protein S1 intoxicated C57/BL6 mice, eugenol (25 mg/kg body weight/d) administration via gavage upregulated locomotor performance, ameliorated the cardiac condition and LDH level [211]. In another study, an in vitro experiment stated that in the sub-micromolar range [inhibition constant (Ki) = 0.81 μmol/L) eugenol was capable of interfering with the 3CLpro enzymatic activity of SARS-CoV-2 [212].
In the BALB/c mice model of lung injury, eugenol treatment (160 mg/kg body weight) downregulated pulmonary inflammation and remodelling. Eugenol treatment lowered the polymorphonuclear leukocyte (PMN) infiltration within lung parenchyma in comparison to the untreated group. Additionally, the treatment suppressed TNF-α signalling and decreased NF-κβ levels [213].
Truzzi et al. [214] demonstrated that spermidine and eugenol combination treatment on LPS-treated Caco-2 cells and L929 cells increased autophagy which was confirmed by increased level of LC3-II (hallmark molecule of autophagy).
The above experiments clearly show the potentiality of eugenol as an anti-inflammatory and anti-COVID-19 substance. Further clinical study is required to confirm the efficacy.
Thymol
Thymol is an aromatic monoterpenoid compound which is extracted from Thymus vulgaris (common name Ajwain) belonging to the Lamiaceae family. Thymol formulation (trade name: Thymol Mouthwash® by Xepa) alone resulted in a very low reduction in viral load (only 0.5 log10). But in combination with other essential oils, thymol showed antiviral activity against certain enveloped virion HSV type 1 and type 2 (DNA virus), IAV (RNA virus), and rotavirus (RNA virus) [215].
In the case of LPS-induced acute lung injury in mice, thymol (100 mg/kg) suppressed NF-κβ signalling and downregulated TNF-α, IL-6, MDA, and myeloperoxidase levels [216].
In the hyperlipidemic rabbit model, thymol suppressed the activation of pro-inflammation-related genes, like IL-1β, IL-6, TNF-α, and TNF-β, in the aorta. In continuation, high cholesterol (HC) levels induced CRP secretion which may cause cardiovascular disease and also increased IL-6 levels. Thymol was reported to inhibit CRP secretion and downregulate IL-6 levels. Thymol also suppressed mRNA expression of vascular cell adhesion protein-1 and MCP-1 in the HC group of mice [217].
A molecular review states that, caspase-1-mediated IL-1β and IL-18 maturation is promoted by NLRP3 inflammasome components which may be reduced by thymol [218].
In stage 1 COVID-19 cases, gargling with the formula of 1% povidone-iodine (PVP-I) along with essential oils (thymol, eucalyptol, methyl salicylate, and menthol) has the potential to eliminate the SARS-CoV-2 virus [219].
Prospective role of phytochemicals in the management of post-COVID complications
SARS-CoV-2 infections result in significant long-term effects leading to a wide range of post-COVID complications including new-onset diabetes mellitus, neurodegenerative disease, kidney and heart failure, and liver and lung injury.
New-onset diabetes
The risk of COVID-19-induced new-onset diabetes in patients may increase due to cytokine storm during the active phase of infection causing impaired insulin secretion and resistance due to the destruction of pancreas beta cells, hyperglycaemia due to stress and steroid drugs [220].
Anthocyanins, a group of flavonoids are known to mitigate diabetes complications by reducing oxidative stress via lowering the ROS production, downregulating the expression of NADPH oxidase and GPx as well as CYP2E1; resolving inflammation via decreasing levels of CRP, MCP-1, IL-6, and TNF-α; reducing insulin resistance via AMPK and TLR pathways [221]. According to the therapeutic role in the diabetes complications of COVID-19, anthocyanins seem to be a hopeful candidate in combating COVID-19-associated diabetes. The phytochemicals isolated from Momordica charantia (bitter melon) extract such as momordicosides, momordin, and saponins possess anti-diabetic activity by protecting pancreatic β-cells. This extract can reduce diabetes by inhibiting diabetes-related enzymes, such as α-glucosidase and α-amylase, lowering gluconeogenesis, activating peroxisome proliferator-activated receptor (PPAR) δ and PPAR α [222].
An in vivo study was carried out to show that oral treatment with Aloe vera water extract containing alkaloids, flavonoids, tannins, phenols, saponins, carbohydrates, vitamins, and minerals reduced marked blood glucose levels by decreasing insulin resistance [223].
Gymnemic acids, a group of triterpenoid saponins extracted from Gymnema sylvestre leaves, enhanced the regeneration of pancreatic β-cells thereby encouraging insulin production, and stimulating insulin sensitivity and insulin activity [222]. The aforementioned effects of gymnemic acids may prove helpful in treating diabetes after recovering from COVID-19.
Quercetin, quercitrin, and rutin, extracted from Euphorbia hirta, were shown to decrease blood glucose levels by inhibiting α-glucosidase and α-amylase [224]. These extracts have potential therapeutic applications for post-COVID diabetic treatment.
Parkinsonism
Increased oxidative stress and neuroinflammation triggered by SARS-CoV-2 infection led to misfolding and aggregation of the resulting alpha‐synuclein (α‐Syn) in the brain activating microglia which is a pathological hallmark of Parkinsonism [225].
EGCG inhibited neuroinflammation by downregulating microglial secretion of iNOS and TNF-α. Evidence also indicated that it exerted a neuroprotective role by inhibiting the formation of nontoxic α-Syn oligomers aggregation [226], thereby could be a novel drug for the treatment of Parkinson’s disease.
Eugenol exhibited protective effects by inhibiting the expression level of TLR4 markers via suppression of pro-inflammatory mediators in human macrophages [227] and seems to be a hopeful candidate in combating related neuronal manifestations of Parkinson’s disease.
Andrographolide, diterpene lactone, exerted a significant neuroprotective effect by inhibiting the expression level of ROS, TNF-α, PGE2, iNOS, and COX-2; attenuating inflammation-mediated dopaminergic neurodegeneration [228]. This finding indicates that andrographolide seems to be a therapeutic candidate for the treatment of inflammation-related neurodegenerative disorders such as Parkinson’s disease.
An in vitro study with Brassica juncea (Indian mustard) leaf extract containing various polyphenolic antioxidant phytochemicals such as sinapic acid, rutin, ferulic acid, caffeic acid, gallic acid, catechol, etc. showed a reduction of the levels of monoamine oxidase-B (MAO-B) and increased the level of dopamine, SOD, CAT, and glutathione in brain [229]. These findings highlight how phytochemicals could be an effective therapeutic approach to Parkinson’s disease.
Post-COVID‐19 lung infections
Systemic lung parenchymal and microvascular inflammation induced by COVID-19-associated cytokine storm promotes the development of viral pneumonia-induced ARDS with associated hypercoagulable states and microthrombosis [230].
Several reported plant-derived phytomolecules such as flavonoids (luteolin, baicalein, quercetin, rutin, kaempferol, mangiferin, naringin, hersperitin, etc.), alkaloids (berberine, cordycepin, betanin, bergenin, etc.), terpenoids (pogostone, eucalyptol, limonene, thymol, andrographolide, dehydroandrographolide, costunolide, ginsenoside, betulinic acid, platycodin D, saikosaponin A, isoforskolin, etc.), and other polyphenol compounds (zingerone, octyl gallate, resveratrol, EGCG, tannic acid, geraniin, etc.) have potential therapeutic effects in the treatment of acute lung infection through modulation of NF-κβ, MAPK, and Nrf2 signalling pathways owing to their anti-inflammatory and antioxidant activities [231].
Post-COVID‐19 kidney failure
Some patients having problems related to the kidney exhibited AKI after COVID due to the destruction of renal resident cells triggered by exposure of the virus to the kidney through blood circulation. As a result, the targeting of ACE2 protein expressed in kidney cell by SARS-CoV-2 promoted the elevation of plasma pro-inflammatory factors (IL-2, IL-7, GSCF, IP-10, MCP-1, MIP1A, and TNF-α) [232]; hyper-activation of immune cells such as CD68(+) macrophages, CD4(+) T cells, and CD56(+) NK cell [233]. However, chronic kidney disease may be a risk factor for severe SARS-CoV-2 infection [234].
Oxymatrine, an alkaloid, is extracted from the Sophora flavescens exerted renal protective effect by decreasing NAG (N-acetyl-beta-d-glucosaminidase), BUN, and creatine (CRE) serum levels as well as by inhibiting the release of pro-inflammatory cytokines including IL-6, IL-1β, and TNF-α [235]. The polyphenol-rich extract of Ocimum gratissimum leaves alleviated oxidative stress, suppressed uncontrolled surge of pro-inflammatory cytokines and leukocytes infiltration, and stimulated antioxidant enzymatic activity of the renal tissues [236]. Experimental results revealed promising phytochemical candidates for management of post-COVID renal injury.
Post-COVID‐19 heart failure
Most people admitted to the hospital with COVID-19 have been facing heart muscle injury due to systemic cardiac inflammation. The phytochemicals such as flavonoids, triterpenoids, and tannins extracted from various parts of Terminalia arjuna plant were reported for treating heart failure condition [237].
Myricetin extracted from Vitis vinifera exhibited immunostimulating and cardiovascular protective activity by reducing the levels of cardiac markers including LDH, CRE kinase (CK), aspartate aminotransferase (AST), SOD, CAT, as well as modulating vascular reactivity and electrocardiographic (ECG) patterns [238]. Juglone, a phenolic compound from the black walnut tree (Juglans nigra) can be used as a potential cardioprotective therapeutic agent in elevating the activity of SOD, decreasing oxidative stress, activating the MAPK signalling pathway [239]. Fumaria indica extract contains isoquinoline alkaloids and flavonoids showing cardioprotective activity by reduction of the serum level of cardiac markers [troponin I (cTnI), CRE phosphokinase (CPK), LDH, and AST)] [240]. Paeonol from Moutan Cortex possesses cardioprotective activity via inhibiting ERK1/2/JNK pathway [241].
Potential role of phytochemicals in prophylaxis and therapy of COVID-19 by inhibiting platelet-activating factor
Prophylaxis and therapies for COVID-19 have to be designed to inhibit inflammation and thrombosis, especially in the lung. Platelet-activating factor (PAF) is a central player in both inflammation and thrombosis. Clinical findings have confirmed upregulated coagulation markers and microthromboses in lungs and other organs in severe COVID-19 patients. Among such patients, increased platelet activation and aggregation have been reported [242]. PAF is the most potent mediator for platelet activation and aggregation. Its structure as a glyceryl-ether lipid (1-O-alkyl-2-acetyl-sn-glycero-3-phosphocholine) and first synthetic preparation was described by Demopoulos et al. [243] in 1979.
Different triggers can initiate PAF production in several cells, such as mast cells, macrophages, platelets, neutrophils, and lymphocytes. PAF production stimulates these cells further in an autocrine manner. Mast cells are potent producers of PAF and IL-6, the cytokine implicated in the COVID-19 cytokine storm. PAF has been previously shown to promote lung injury in SARS-CoV-1 infections, and human cells infected with coronavirus HCoV-229E also have elevated PAF levels [244]. Interestingly, S protein has been shown to augment PAF production. In the presence of S protein, PAF-induced aggregation in human platelet-rich plasma (hPRP) from non-vaccinated individuals is enhanced [245]. Hence, scientists have highlighted PAF inhibition as a potential target for drug development in COVID-19.
Currently, no clinically available PAF inhibitors exist except the histamine-1 receptor antagonist rupatadine. Phytochemicals with potential anti-PAF activity present a promising avenue for research. Some phytochemicals already described in the previous sections have shown anti-PAF activities. Quercetin, luteolin, curcumin, and resveratrol have anti-PAF activity [244]. They can reduce the chances of platelet aggregation, thrombosis, and coagulation. Luteolin, for example, can inhibit mast cells, have a neuroprotective role, and reduce cognitive impairment and COVID-19-associated brain fog [246].
The anti-PAF activity of phytochemicals has been linked to their potent anti-inflammatory and anti-thrombotic properties. The ability of these phytochemicals to inhibit a central player, such as PAF, further strengthens the logic behind exploring them for the prevention and treatment of COVID-19.
Conclusions
Vast knowledge regarding the usefulness of phytochemicals for application in SARS-CoV-2 infection-related pathologies has accumulated since the isolation of this virus. A number of phytochemicals can be effective therapeutic candidate to combat SARS-CoV-2 infection as demonstrated by in silico, in vitro, in vivo, and clinical studies. Interventions have been shown to block the cellular entry process of the virus and interfere with several processes of the virus replication cycle inside the cell. Treatment with phytochemicals in addition to existing drugs can mitigate the adverse immune reaction elicited by the virus in certain patients leading to cytokine storm and multi-organ involvement.
In this review, 28 phytochemicals belonging to diverse categories have been noted which exhibit potent inhibition of SARS-CoV-2 activity in different cell types in vitro. In fact, different phytochemicals have been demonstrated to hit the same viral targets as shown in Figure 1. Several phytochemicals can inhibit viral entry to the cells by blocking RBD of the S protein with hACE2 receptor and inhibiting TMPRSS2. Different phytochemical can block the viral replication process by attenuating 3CLpro and RdRp as shown in Figure 1.
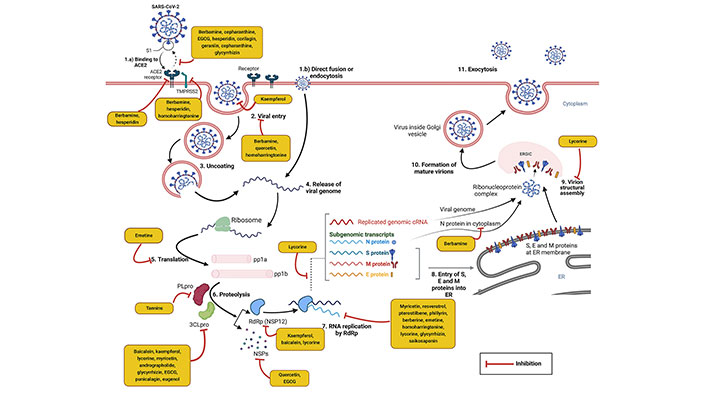
Potential therapeutic targets of different phytochemicals in SARS-CoV-2 infection. The above figure describes the life cycle of SARS-CoV-2 virus and potential therapeutic targets of antiviral phytochemicals. Entry of SARS-CoV-2 virus into the host cell is mediated by S protein and ACE2 binding or by direct fusion or endocytosis (1. a, b); after fusion virus enters the cytosol (2), uncoats (3), and releases the viral genome (4). 5’ end ORF1a and ORF1b undergoes translation and are converted into pp1a and pp1b (5). pp1a and pp1b undergoes proteolysis by PLpro and 3Clpro and cleaved into different NSPs (6). Then the RNA is replicated by RdRp (NSP12) (7). A viral transcription-replication form and translated structural proteins (S, E, and M) enter into the ER (8) which further moves to the ERGIC body. N proteins release into cytosol and form ribonucleoprotein complexes with replicated genomic RNA. Ribonuclease complexes interact with M protein at ERGIC body and perform virion assembly (9). Virions are budded out from ERGIC and mature virions are formed (10) which are released from cell by exocytosis (11). Multiple experimental evidence states the inhibiting efficacy of different phytochemicals in various steps of COVID-19 viral infection which is demonstrated in the above diagram (yellow boxes). Created with BioRender.com
Phytochemicals can act at several levels of the complex immunopathological pathways that start in the lung epithelial cells (Figure 2, panel A & B) but may escalate with the involvement of different organs (Figure 2, panel C). Elevated levels of several clinical markers can be normalized by the effect of phytochemicals (Figure 2, panel D).
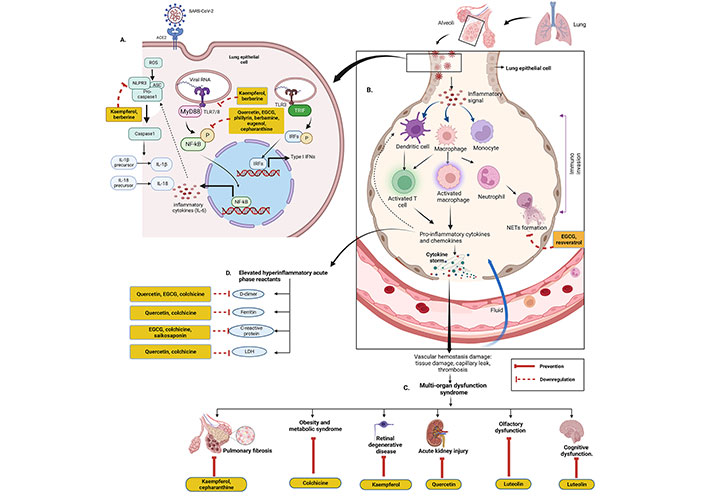
Immune response to SARS-CoV-2 and pathogenesis. (A) Schematic representation of SARS-CoV-2-driven signalling cascade and possible potential phytochemical targets. Upon the entry of SARS-CoV-2 into lung epithelial cells, viral RNA recognized by TLR activates transcription factors, such as NF-κβ and IFN regulatory factors (IRFs) which upregulates the expression of pro-inflammatory cytokines. NLRP3 inflammasome components NLRP3, apoptosis-associated speck-like protein (ASC), and pro-caspase-1 are activated. Activated caspase-1, in turn, triggers the conversion of pro-IL-1β and pro-IL-18 into mature IL-1β and IL-18, respectively, resulting in an inflammatory cytokine storm. (B) Crosstalk between epithelial cells and immune cells leads to cytokine storm. Inflammatory signals induced by SARS-CoV-2 infection lead to macrophage and monocyte activation along with activation and differentiation of T and B cells which in turn triggers the production of huge amounts of pro-inflammatory cytokines. Activated neutrophils release NETs. (C) Clinical manifestations of multi-organ dysfunction syndrome induced by cytokine storm and its prevention by selective phytochemicals. (D) COVID-19 markers and potential phytochemicals targets. TRIF: toll/IL-1R domain-containing adaptor-inducing interferon-β. Created with BioRender.com
Furthermore, the immunomodulatory activity of phytochemicals like flavonoids has been confirmed via the inhibition of various pro-inflammatory cytokines including TNF-α, IL-6, IL-8, IL-1β, IL-17, and IFN-γ and stimulation of different anti-inflammatory cytokines by modulating signalling pathways. Cellular infiltration is coordinated at the inflammation site by a variety of chemokines. The activity of the chemokines can be potentially regulated by a variety of phytochemicals. The several phytochemicals that were experimentally demonstrated to change cytokine and chemokine levels have been summarized in Figure 3.
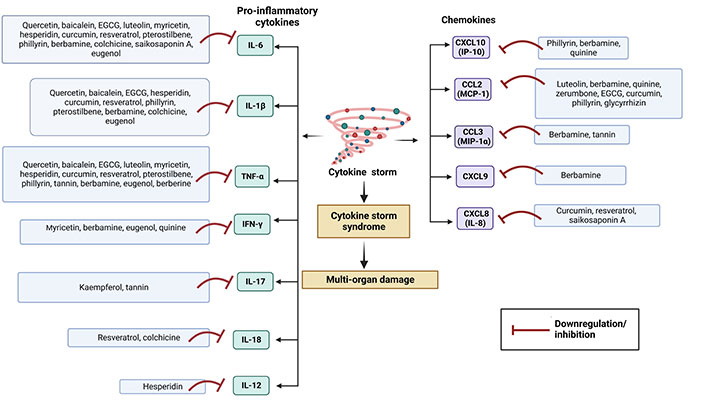
Management of cytokine storm by phytochemicals. Phytochemicals can potentially prevent detrimental cytokine storm in COVID-19 by inhibiting several cytokines and chemokines. Created with BioRender.com
During pandemic, quick patient turnover plays a significant role in vacating hospital beds, especially in critical care units. It was necessary to improve clinical symptoms, promote speedy recovery of patients, and shorten the hospitalization stay times for accommodating new COVID patients. Therapeutic potential of phytochemicals such as hesperidin, resveratrol, berberine, and glycyrrhizin has been reported to shorten recovery time and enhance prognosis. There are various pre-clinical and clinical studies that were carried out to demonstrate traditional phytochemicals as suitable therapeutics regimens for the effective and safe management of the cytokine storm in the COVID-19 condition along with different post-COVID complications.
Even after deploying mass vaccination campaigns and introduction of several antivirals for therapeutic application, newly emerging variants and sub-variants are giving sporadic rise to COVID cases in different countries. Therefore, it is important to evaluate the knowledge acquired from various studies on phytochemicals and determine the most effective ones, whether used singly or in combination. In order to increase the effectiveness of these phytochemicals, it is important to optimize their delivery for improved absorption and bioavailability in future research studies. As scientists warn the public about the prospect of future pandemics with climate change, the development of phytochemicals as a therapeutic tool for the prevention and treatment of viral infection, remains an important component of public health security.
Abbreviations
3CLpro: |
3-chymotrypsin-like protease |
ACE2: |
angiotensin-converting enzyme 2 |
AKI: |
acute kidney injury |
Akt: |
protein kinase B |
Ca2+: |
calcium |
CAT: |
catalase |
CC50: |
half-maximal cytotoxic concentration |
CCL: |
chemokine (C-C motif) ligand |
COVID-19: |
coronavirus disease 2019 |
COX-2: |
cyclooxygenase‐2 |
CQ: |
chloroquine |
CRE: |
creatine |
CRP: |
C-reactive protein |
CXCL: |
chemokine (C-X-C motif) ligand |
E: |
envelope |
EC50: |
half-effective concentration |
EGCG: |
epigallocatechin-3-gallate |
ERGIC: |
endoplasmic reticulum-Golgi intermediate compartment |
ERK: |
extracellular signal-regulated kinase |
FDA: |
United States Food and Drug Administration |
hACE2: |
human angiotensin-converting enzyme 2 |
HCoV: |
human coronavirus |
HCQ: |
hydroxychloroquine |
HEK: |
human embryonic kidney |
HIF: |
hypoxia-inducible factor |
HO: |
heme oxygenase |
IC50: |
half-maximal inhibitory concentration |
IFN: |
interferon |
Iκβα: |
inhibitory kappa βα |
IL: |
interleukin |
iNOS: |
inducible nitric oxide synthase |
IP-10: |
interferon-γ inducible protein-10 |
JAK: |
Janus kinase |
JNK: |
c-Jun N-terminal kinase |
LDH: |
lactate dehydrogenase |
LPS: |
lipopolysaccharide |
M: |
membrane |
MAPK: |
mitogen-activated protein kinases |
MCP-1: |
monocyte chemoattractant protein-1 |
MERS: |
Middle East respiratory syndrome |
MIP: |
macrophage inflammatory protein |
MRC5: |
medical research council cell strain 5 |
N: |
nucleocapsid |
NFV: |
nelfinavir |
NF-κβ: |
nuclear factor kappa β |
NK: |
natural killer |
NLRP: |
pyrin domain-containing protein |
NO: |
nitric oxide |
Nrf2: |
nuclear factor erythroid-derived 2-like 2 |
NSPs: |
non-structural proteins |
ORF: |
open reading frame |
PAF: |
platelet-activating factor |
PF: |
pulmonary fibrosis |
PLpro: |
papain-like protease |
pp1a: |
polyprotein 1a |
pp1b: |
polyprotein 1b |
QCB: |
quercetin, vitamin C, and bromelain |
QS: |
quinine sulfate |
RBD: |
receptor binding domain |
RCT: |
randomized controlled trial |
RdRp: |
RNA-dependent RNA polymerase |
ROS: |
reactive oxygen species |
S: |
spike |
SARS-CoV-2: |
severe acute respiratory syndrome coronavirus 2 |
Smad3: |
mothers against decapentaplegic homolog 3 |
SOD: |
superoxide dismutase |
STAT: |
signal transducer and activator of transcription |
TGF: |
transforming growth factor |
TLR: |
toll-like receptor |
TMPRSS2: |
transmembrane protease serine 2 |
TNF: |
tumor necrosis factor |
Declarations
Author contributions
AD and SK: Writing—original draft, Visualization. SR: Conceptualization, Writing—review & editing. SD: Conceptualization, Writing—original draft, Writing—review & editing, Supervision. All authors read and approved the submitted version.
Conflicts of interest
The authors declare that they have no conflicts of interest.
Ethical approval
Not applicable.
Consent to participate
Not applicable.
Consent to publication
Not applicable.
Availability of data and materials
Not applicable.
Funding
Shantanabha Das is supported by start-up research grant from
Copyright
© The Author(s) 2023.