Abstract
Traditional medicine systems worldwide utilize natural products (NPs), including plant-derived compounds, minerals, and organisms, harnessing their healing potential. NPs offer a rich source of potential drug candidates, driving innovation in drug discovery. Recent breakthroughs have reignited interest in harnessing the therapeutic benefits of natural compounds. Clinical applications of NP-based immunotherapies, such as curcumin and resveratrol in cancer treatment, highlight their diverse pharmacological properties. However, despite these advancements, challenges persist in the clinical implementation of NPs. Issues such as standardization, regulatory approval, and supply sustainability remain significant hurdles. Overcoming these limitations requires a concerted effort to address the complexities of NP drug development. Nevertheless, ongoing research efforts and interdisciplinary collaboration hold promise for advancing NP-based therapeutics, paving the way for the development of innovative treatments for various diseases. In the world of precision medicine, a new chapter unfolds as NPs join the therapeutic journey. The exploration of NPs as sources of bioactive compounds has revealed promising prospects for precision therapeutics in medicine. This article explores the therapeutic potential of NPs within the context of precision medicine. It examines the intricate pathways through which bioactive compounds derived from nature offer tailored therapeutic prospects, emphasizing their role in precision medicine interventions. Exploring the synergy between NPs and precision therapeutics at a molecular level, this article delineates the exciting prospect of customized treatments, signifying a transformative impact on modern medical care. The review article further highlights their potential in tailoring treatments based on individual genetic makeup and disease characteristics. Additionally, it discusses challenges and prospects, addressing issues of sourcing, standardization, scalability, and regulatory considerations to realize the full therapeutic potential of NPs.
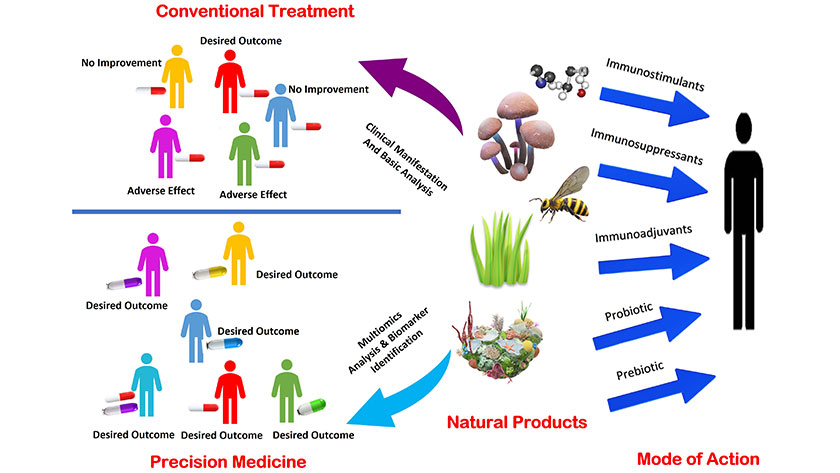
Revolutionizing drug development: conventional treatment vs. precision medicine utilizing natural sources
Keywords
Precision medicine, natural products (NPs), bioactive compounds, tailored therapies, immunomodulationIntroduction
Natural products (NPs) have been used in healthcare systems since ancient times, drawing from the rich biodiversity and traditional knowledge of different cultures. NPs, derived from diverse biological sources including plants, fungi, and marine organisms, have long served as reservoirs of bioactive compounds with profound effects on immune function. Historically, drug discovery has been closely linked to NPs. Traditional medicines are effective treatments for various health conditions, such as malaria, pain, and cancer [1]. Today, there is a renewed interest in exploring the bioactive compounds found in nature. Over the past decades, research efforts have revealed many bioactive compounds from natural sources that possess immunomodulatory properties. These compounds exhibit a spectrum of effects, ranging from potent immunostimulation to immunosuppression, thereby offering versatile tools for the treatment of various immune-related disorders. Notably, the advent of advanced analytical techniques has facilitated the identification and characterization of key bioactive constituents, enabling a deeper understanding of their mechanisms of action and therapeutic potential. The applications of modern techniques, such as multi-omics, network pharmacology, and DNA barcoding, help to validate and document the pharmacological and phytochemical aspects of traditional medicine [2].
Many NPs have contributed to the development of modern drugs, such as aspirin, artemisinin, and vincristine. However, the scientific validation and standardization of NPs are still challenging and require interdisciplinary approaches and evidence-based methods. Precision medicine, characterized by tailored therapeutic interventions based on individual genetic, molecular, and clinical profiles, has emerged as a paradigm shift in healthcare delivery. The complex interplay between the immune system and human health has prompted significant interest in harnessing the immunomodulatory properties of NPs for precision medicine applications. This growing field represents a convergence of traditional knowledge and modern scientific approaches, aimed at elucidating the therapeutic potential of NPs in modulating immune responses with precision and efficacy. By leveraging the diversity and complexity of NP chemistry, clinicians can envision a future where personalized immunotherapy strategies are tailored to the unique immunological landscape of each patient.
This review aims to provide a comprehensive overview of the current state-of-the-art in harnessing the immunomodulatory potential of NPs within the context of precision medicine. Furthermore, we will discuss emerging strategies for integrating NP-based immunotherapies into clinical practice, with a focus on personalized treatment approaches and future directions for research and development. In summary, this review underscores the pivotal role of NPs in shaping the future of precision immunotherapy and tries to unlock new avenues for therapeutic innovation and ultimately enhance the management of immune-related disorders.
Biological diversity of natural sources
NPs are organic compounds derived from living organisms, such as plants, fungi, and marine organisms. They exhibit remarkable structural and functional diversity and have been a rich source of bioactive molecules for drug discovery and development. NPs have played a vital role in the treatment of various diseases, such as cancer, infectious diseases, inflammation, and neurodegenerative disorders.
Plants are a rich and diverse source of NPs, which have been utilized for healing purposes in various traditional medicine systems, such as Ayurveda, Chinese medicine, and Unani medicine for a long time. Plants synthesize a variety of secondary metabolites, such as alkaloids, flavonoids, terpenoids, phenolics, and glycosides, that exhibit different biological activities and therapeutic potentials (Table 1). Some well-known drugs derived from plants include aspirin, morphine, quinine, vincristine, and artemisinin. Plant NPs can influence the immune system by modulating innate and adaptive immunity, cytokine secretion, inflammation, and cell signaling [2–4].
Plant-derived natural products (NPs) with immunomodulatory effect
Major source | NP | Mode of action | References |
---|---|---|---|
Aloe vera | Acemannan |
| [5–9] |
Withania somnifera | Withanolides |
| [10–12] |
Astragalus membranaceus | Astragalosides |
| [13–15] |
Berberis vulgaris | Berberine |
| [16–18] |
Betula pubescens and Bacopa monnieri | Betulinic acid |
| [19–22] |
Boswellia serrata | Boswellic acids |
| [23–25] |
Boswellia sacra | Frankincense |
| [26–28] |
Curcuma longa | Curcumin |
| [29–31] |
Camellia sinensis | Epigallocatechin gallate |
| [32, 33] |
Tanacetum parthenium | Parthenolide |
| [34, 35] |
Allium sativum | Allicin, S-allylcysteine |
| [36, 37] |
Zingiber officinale | Gingerol, shogaol |
| [38, 39] |
Vitis vinifera | Proanthocyanidins |
| [40, 41] |
Camellia sinensis | Catechins |
| [42, 43] |
Ocimum sanctum | Eugenol, ursolic acid |
| [44–46] |
Glycyrrhiza glabra | Glycyrrhizin |
| [47, 48] |
Olea europaea | Oleuropein |
| [49–51] |
Citrus fruits, apples, onions, parsley, sage, tea, red wine, olive oil, grapes, dark cherries, and dark berries such as blueberries, blackberries, and bilberries | Quercetin |
| [52, 53] |
Polygonum cuspidatum and Vitis vinifera | Resveratrol |
| [54, 55] |
Rhodiola rosea | Salidroside, rosavin |
| [56–58] |
Silybum marianum | Silybin |
| [59, 60] |
Zanthoxylum alatum and Ruta graveolens | Skimmianine |
| [61–63] |
Petroselinum crispum, Melissa officinalis, Origanum vulgare, and Justicia gendarussa | Apigenin |
| [64–66] |
Panax ginseng | Ginsenosides |
| [67–70] |
Plant-derived NPs encompass a diverse range of bioactive compounds that exert immunomodulatory effects, examples include curcumin, known for its anti-inflammatory and immunostimulatory properties [71], and quercetin found in fruits and vegetables, which has shown efficacy in autoimmune disease management [72]. Additionally, apigenin, present in parsley and chamomile, etc., exhibits anti-inflammatory and immunosuppressive effects [73], while skimmianine, derived from various plant sources, has been studied for its immunomodulatory potential [63].
Other NPs like fungi, insects, marine organisms, etc. stand out as a prolific source of NPs renowned for their diverse therapeutic potentials, encompassing antimicrobial, anticancer, antiviral, and immunosuppressive properties (Table 2). Within these categories lies a spectrum of bioactive compounds, with well-established drugs such as penicillin, lovastatin, and cyclosporine. Penicillin, derived from Penicillium sp., remains one of the most widely used antibiotics worldwide, while lovastatin, sourced from Aspergillus sp., boasts cholesterol-lowering capabilities alongside its anticancer effects. Cyclosporine, extracted from Tolypocladium sp., serves not only as an immunosuppressive agent but also exhibits antiviral properties. Other examples of antimicrobial fungal NPs include griseofulvin, amphotericin B, echinocandin, and caspofungin [74, 75].
Other natural products (NPs) with immunomodulatory effects
Source | Taxonomic species | NP | References |
---|---|---|---|
Fungi | Cordyceps sinensis | Cordycepin | [76, 77] |
Insects | Apis mellifera | Propolis | [78, 79] |
Various species | Lactobacillus, Bifidobacterium | Lactobacillus, Bifidobacterium | [80, 81] |
Algae | Spirulina platensis | Phycocyanin | [82, 83] |
Marine organisms | Haliotis diversicolor | Abalone Haliotis peptide | [84, 85] |
Sargassum fusiform | Fucoidans | [86, 87] | |
Algae, yeast, salmon, trout, krill, shrimp, and crayfish | Astaxanthin | [88, 89] | |
Cartilage | Shark and bovine cartilage | Chondroitin sulfate | [90, 91] |
Fish | Fish oil | Eicosapentaenoic acid (EPA), docosahexaenoic acid (DHA) | [92, 93] |
The antiviral properties and immune-boosting effects of polysaccharides derived from bacteria, fungi, and algae have been reported [94]. The mechanisms of action and potential applications of polysaccharides against various viruses, especially severe acute respiratory syndrome coronavirus 2 (SARS-CoV-2), the causative agent of COVID-19 have been reported and suggest that microbial and algal polysaccharides can be used as adjuvants, nutrient supplements, and drug delivery systems to prevent and/or inhibit viral infections [95].
Fucoxanthin, derived from brown seaweeds, emerges as a promising natural anticancer compound, targeting key pathways involved in cancer development and progression, such as cell cycle regulation, apoptosis induction, angiogenesis inhibition, and autophagy modulation [96]. Small molecule-based approaches are highlighted as effective means to intervene in critical signaling pathways dysregulated in hepatocellular carcinoma (HCC), offering the potential to lessen key aspects of HCC behavior including proliferation, migration, invasion, metastasis, and recurrence [97]. The apoptotic activity of plant and fungal extracts on cancer cells, isolation of active compounds, their mechanisms of action, and clinical trial status, standardization, and bioavailability, emphasize the need for further research to fully unlock their therapeutic potential in cancer treatment [98].
The anti-inflammatory properties of Betulinic acid, Apigenin and Skimmianine (BASk), a novel drug formulation comprising betulinic acid (B), apigenin (A), and skimmianine (Sk) have been studied and it reveals the molecular mechanism underlying the therapeutic effects of BASk, particularly its involvement in CD36-mediated activation of the Toll-like receptor 2 (TLR2)-NOD-like receptor protein 3 (NLRP3) signaling pathway [99]. Some of the recent studies on NPs are listed in Table 3.
Some of the recent studies in natural products (NPs)
Sl No | Scientific title | Year | Source register | Web address | Countries |
---|---|---|---|---|---|
1 | Evaluate and compare effect of Platelet Rich Plasma(PRP) and Nutraceuticals in pain management of Post Herpetic Neuralgia | 2024 | CTRI | https://ctri.nic.in/Clinicaltrials/pmaindet2.php?EncHid=MTAxNjA4&Enc=&userName=Evaluate%20and%20compare%20effect%20of%20Platelet%20Rich | India |
2 | Evaluation of the safety and the efficacy of a novel polyherbal mouthwash containing Emblica officinalis,Cinnamomum cassia,Silicate of magnesia,Nigella sativa and Eucalyptus globulus oil in patients with gingivitis:A randomized controlled trial | 2024 | CTRI | https://ctri.nic.in/Clinicaltrials/pmaindet2.php?EncHid=OTM1NDg=&Enc=&userName=CTRI/2024/01/061413 | India |
3 | Beneficial Effects of Specific Natural Products on Management of Xerostomia: A Randomized Controlled Clinical Trial | 2024 | ClinicalTrials.gov | https://clinicaltrials.gov/ct2/show/NCT06217614 | Egypt |
4 | Effect of taking foods containing natural products on mental and physical health in adult women | 2023 | JPRN | https://center6.umin.ac.jp/cgi-open-bin/ctr_e/ctr_view.cgi?recptno=R000055834 | Japan |
5 | The impact of dietary fibre supplementation on gum disease; A randomised control trial in healthy volunteers | 2023 | ANZCTR | https://anzctr.org.au/ACTRN12623001067662.aspx | Australia |
6 | Safety, Pharmacokinetics, and Preliminary Efficacy of Herbal Products for the Treatment of Acute Respiratory Viral Infections Including Severe Acute Respiratory Syndrome Coronavirus 2 (SARS-CoV-2) in Uganda; Phase 2A Open Label Clinical Trial | 2023 | ClinicalTrials.gov | https://clinicaltrials.gov/show/NCT05897203 | Uganda |
7 | A dietary supplement for the management of patients with lumbar osteochondrosis | 2021 | ISRCTN | https://www.isrctn.com/ISRCTN17230715 | Austria |
8 | Evaluation of the Effects of a Nutraceutical Composition Containing Derivatives From Natural Products on the Modulation of the Endocrine Neuroimmune Axis - Translational Study. | 2021 | ClinicalTrials.gov | https://clinicaltrials.gov/ct2/show/NCT04810572 | Brazil |
9 | A dietary supplement for semen parameters quality improvement in low sperm count and azoospermia patients | 2020 | ISRCTN | https://www.isrctn.com/ISRCTN15796121 | Egypt |
10 | Pilot study on the effectiveness of selected natural products from traditional Iranian medicine sources (Bore armani and Raphanus niger Mill. combination/mixture) on the treatment of kidney stones | 2020 | IRCT | https://irct.behdasht.gov.ir/trial/49630 | Iran |
CTRI: Clinical Trials Registry – India; JPRN: Japan Primary Registries Network; ANZCTR: Australian New Zealand Clinical Trials Registry; ISRCTN: International Standard Randomized Controlled Trial Number; IRCT: Iranian Registry of Clinical Trials
Immunomodulatory effect of NPs
Immunomodulation is the process of altering the immune system by enhancing or suppressing its activity. Immunomodulation can be achieved by various agents, such as cytokines, antibodies, vaccines, and drugs. However, these agents may have adverse effects, such as toxicity, resistance, and immunosuppression. Many NPs have shown significant immunomodulatory and overall health-benefiting effects to humans, with no or minimal toxicity. These NPs offer promising alternatives for immunomodulation, as they can modulate the immune system and participate in various processes of innate and adaptive immunity [100]. The relevance of NPs in immunomodulation ideates from their multifaceted effects on immune cells, cytokines, and signaling pathways. These compounds can exert their immunomodulatory effects through various mechanisms, including:
Direct interaction with immune cells: NPs may directly bind to immune cell receptors or enzymes, thereby influencing their activation, proliferation, or differentiation. For example, certain plant-derived compounds can interact with TLRs or major histocompatibility complex (MHC) molecules, modulating antigen presentation and immune cell activation [101–104].
Regulation of cytokine production: Many NPs possess anti-inflammatory or pro-inflammatory properties by modulating the production and release of cytokines, which are key mediators of immune responses. NPs like Echinacea extract, curcumin, genistein, eugenol, 6-gingerol, thymoquinone, allicin, quercetin, betulinic acid, emodin, and parthenolide have shown pronounced effects on cytokine production and secretion in several physiological conditions [105–116].
Induction of regulatory immune responses: Some NPs have been shown to promote the generation of regulatory immune cells, such as regulatory T cells (Tregs) or M2 macrophages, which play critical roles in maintaining immune homeostasis and tolerance [117–119]. By fostering a tolerogenic immune environment, these compounds may be beneficial for managing autoimmune diseases or preventing transplant rejection.
Modulation of immune cell trafficking: NPs can influence the migration and homing of immune cells to specific tissues or sites of inflammation through chemotactic effects or modulation of adhesion molecule expression [120, 121]. This can impact the intensity and duration of immune responses, shaping the overall immune landscape in various disease contexts.
Activation of immune cells
NPs can exert immunomodulatory effects by different mechanisms.
Immunostimulatory NPs can activate various immune cells, such as macrophages, dendritic cells, natural killer cells (NKs), T cells, and B cells, and increase the production and secretion of various cytokines, chemokines, and antibodies. It can also enhance the antigen presentation, phagocytosis, cytotoxicity, and proliferation of immune cells. They can be used for the prevention and treatment of infectious diseases, chronic inflammatory diseases, and immunodeficiency [122–125].
Immunosuppressive NPs can inhibit various immune cells, such as T cells, B cells, and mast cells, and decrease the production and secretion of various cytokines, chemokines, and antibodies. They can also inhibit the antigen presentation, activation, differentiation, and migration of immune cells. Such NPs can be used for the treatment of autoimmune diseases, such as rheumatoid arthritis, multiple sclerosis, type 1 diabetes, inflammatory bowel disease, and allergic diseases, such as asthma, eczema, and anaphylaxis [126–129].
Dual-action compounds are NPs with both stimulatory and suppressive effects on immunity. They can modulate the immune system in a balanced and context-dependent manner, depending on the type, dose, and duration of exposure to the NP, and the status, phenotype, and function of the immune cells. It can regulate the immune system by affecting the expression and activity of various receptors, enzymes, transcription factors, and signaling molecules involved in immune regulation. Dual-action NPs can be used for the treatment of chronic inflammatory diseases, such as atherosclerosis, obesity, and neurodegeneration, and the enhancement of vaccine efficacy [105, 130]. Some of the NPs and their mode of action are described in Tables 1 and 2.
Synergistic effects of combining NPs with traditional anti-cancer treatments
NPs have demonstrated significant potential in enhancing the efficacy of traditional anti-cancer treatment strategies. Combining NPs with conventional therapies can result in synergistic effects, leading to improved treatment outcomes and reduced side effects. Several studies have highlighted the synergistic effects of NPs with chemotherapy agents. For example, the combination of curcumin, a bioactive compound derived from turmeric, with doxorubicin has been shown to enhance the cytotoxic effects on cancer cells and reduce drug resistance [131]. Similarly, resveratrol, found in grapes and red wine, has been reported to enhance the anti-cancer effects of paclitaxel in breast cancer cells [132].
NPs can also potentiate the effects of radiation therapy. For instance, epigallocatechin gallate (EGCG), a polyphenol found in green tea, has been shown to sensitize cancer cells to radiation, leading to increased apoptosis and reduced tumor growth. Co-treatment with EGCG and tumor necrosis factor (TNF)-related apoptosis-inducing ligand (TRAIL), of highly aggressive colon cancer cells, synergistically increased cytotoxicity, by upregulation of death receptor 5 (DR5) and activation of caspase 8 demonstrating that EGCG can be a potent TRAIL sensitizer [133].
Synergistic effects of EGCG and L-theanine, which are key functional components of green tea, on nerve repair and regeneration can inhibit inflammation, promote metabolism, and nourish nerve cells, leading to potential benefits in the repair and regeneration of nerve cells. The combination of these compounds promotes nerve cell health and slows down the progression of neurodegenerative diseases like Alzheimer’s [134].
The synergistic effects observed when combining NPs with traditional anti-cancer treatments can be attributed to several mechanisms, including: enhanced cancer cell death: NPs can induce apoptosis and autophagy in cancer cells, thereby enhancing the cytotoxic effects of chemotherapy and radiation therapy and reducing the development of drug resistance to chemotherapy agents [135–137].
Understanding and harnessing the synergistic effects of combining NPs with traditional anti-cancer treatment strategies can lead to improved treatment outcomes and reduced side effects. Further research is needed to explore the optimal combinations, doses, and treatment schedules to maximize the synergistic effects and advance personalized cancer therapy.
The synergistic effects of natural compounds with conventional therapeutics against colorectal cancer progression and metastasis were well established [138]. Various in vitro and in vivo studies have shown that combining NPs with tamoxifen (TAM) results in synergistic anti-cancer effects. This combination enhances the inhibition of tumor cell growth, increases TAM sensitivity, and reduces the side effects or toxicity associated with TAM. However, some NPs, such as Angelica sinensis (Oliv.) Diels [Apiaceae], Paeonia lactiflora Pall., Rehmannia glutinosa (Gaertn.) DC., Astragalus mongholicus Bunge, and Glycyrrhiza glabra L. [Fabaceae], exhibit estrogen-like activity. This estrogenic activity might diminish the anti-cancer efficacy of TAM. On the other hand, certain NPs like morin, silybin, EGCG, myricetin, baicalein, curcumin, kaempferol, and quercetin have been found to enhance the bioavailability of TAM and its metabolites in vivo. Nevertheless, there is a lack of extensive clinical studies investigating the combined use of NPs and TAM [139].
The synergistic effects of the herbal mixture C5E in combination with the chemotherapeutic drug gemcitabine on the pancreatic cancer cell line PANC-1 notably decreased the SP cell percentage from 8.2% (control) to 5.1%, and more effectively induced early apoptosis compared to individual treatments. Additionally, the combination treatment led to a greater downregulation of sonic hedgehog mRNA expression, a protein associated with certain cancer types, compared to individual treatments [140].
The synergistic effects of Clinacanthus nutans Lindau (C. nutans) extracts when combined with gemcitabine, a conventional chemotherapy drug for pancreatic cancer. The research revealed that the non-polar stem extracts of C. nutans (SN extracts) synergistically enhanced the efficacy of gemcitabine, allowing for a dosage reduction of gemcitabine by 2.38 times to 5.28 times while maintaining its therapeutic effects. The combination treatment more effectively induced apoptosis in pancreatic ductal adenocarcinoma cells compared to either treatment alone, as indicated by the upregulation of Bax and downregulation of anti-apoptotic markers like Bcl-2, cIAP-2, and XIAP. The study concludes that although C. nutans extracts are not effective as a standalone cancer treatment, they can enhance the anti-tumor mechanism of gemcitabine [141].
In a study conducted by Kapadia et al. [142] (2013), B. vulgaris was co-administered with doxorubicin to pancreatic, breast, and prostate cancer cells. The combination of B. vulgaris and doxorubicin resulted in synergistic cytotoxic effects on pancreatic and breast cancer cells, but not on prostate cancer cells. This combined treatment approach with B. vulgaris has been reported to allow for a reduction in the dosage of anticancer drugs, thereby reducing adverse effects.
Collectively, these studies suggest that integrating NPs into cancer treatment protocols can enhance efficacy and reduce side effects, warranting further exploration to optimize treatment outcomes.
Role of NPs in managing tumor microenvironment
The potential of NPs to remodel the tumor microenvironment and overcome drug resistance in cancer immunotherapy by targeting and regulating immune cells, such as T cells, macrophages, and mast cells, as well as inflammatory cytokines within the tumor microenvironment has been well established [143].
Natural compounds function as metabolic modulators of the tumor microenvironment by modulating the metabolism of cells within the tumor microenvironment and their metabolic crosstalk as a promising strategy in anticancer therapies [144].
The study by Deng et al. [145] provides an overview of NPs and their derivatives as promising modulators of tumor immunotherapy. It discusses how these compounds can target various components of the immune system, including T cells, macrophages, B cells, NKs, Tregs, and myeloid-derived suppressor cells, and affect key pathways such as nuclear factor kappa-light-chain-enhancer of activated B cells (NF-κB), PI3K/Akt, mitogen-activated protein kinase (MAPK), and Janus kinase (JAK)/signal transducers and activators of transcription (STAT). These studies suggest that NPs can enhance the therapeutic outcome of cancer immunotherapies by remodeling the tumor immunosuppressive microenvironment.
Precision medicine
Precision medicine represents an emerging approach in healthcare, aiming at tailored treatment solutions uniquely suited to each patient’s genetic, molecular, and clinical makeup. This innovative approach holds promise for enhancing the diagnosis, prognosis, prevention, and treatment of a wide array of diseases, with a particular focus on immune-related disorders like diabetes, arthritis, atherosclerosis, cancer, autoimmune conditions, and allergies [146]. These disorders, characterized by complex and diverse manifestations, involve disruptions in the body’s immune system, its crucial defense mechanism against external threats, and abnormal cellular activity [147, 148]. Through precision medicine, the underlying triggers and pathways of immune-related disorders can be differentiated, enabling the customization of treatment strategies to address individual patient needs effectively.
NPs and personalized medicine
Personalized therapy is one of the most promising applications of precision medicine in immune-related disorders. NPs can be used as potential sources of personalized immunotherapy, as they can target specific genes, proteins, and pathways involved in immune regulation, and induce specific immune responses against diseases. It plays a significant role in personalized immunotherapy, offering diverse compounds with potential therapeutic benefits in combating immune-related disorders. For example, EGCG from green tea has been found to inhibit certain immune checkpoint molecules, offering potential therapeutic benefits in cancer immunotherapy [149].
NPs can also be used as adjuvants, supplements, or delivery systems to enhance the efficacy and safety of immunotherapy. NPs can enhance the efficacy of immunotherapy when used as adjuvants or in combination with conventional treatments. Compounds like quercetin, found in fruits and vegetables, have been shown to improve the effectiveness of immunotherapy by sensitizing cancer cells to immune-mediated destruction [150, 151].
Many NPs exhibit minimal toxicity compared to synthetic drugs, making them attractive candidates for personalized immunotherapy. For example, compounds like apigenin, found in parsley and chamomile, have shown promising anti-inflammatory and immunomodulatory effects with few adverse reactions [152, 153].
Extensive preclinical and clinical investigations have explored the therapeutic potential of these compounds in personalized immunotherapy, shedding light on their efficacy, safety, and mechanisms of action in immune-related disorders [154]. Precision medicine emphasizes individualized treatment tailored to each patient’s unique genetic, molecular, and clinical profiles. In the context of NPs, personalization can be based on, disease pathophysiology, multiomics-screening of biomarkers, the patient’s age and health status, and interactions with other medications. However, recent advancements in genomic technologies have enabled rapid diagnosis and interpretation of genetic variations influencing therapy response, paving the way for targeted therapies that address specific disease features [155, 156]. Initiatives like the Human Genome and The Cancer Genome Atlas (TCGA) projects strive to elucidate the role of genetics and gene expression in disease progression and therapy response. These developments offer promise in transforming drug discovery by leveraging genomic insights to identify new therapeutic targets and optimize treatment strategies. The “gene to screen” approach, focusing on genes as determinants of cellular phenotype, has emerged as a promising strategy in drug discovery, facilitating the identification of novel drug targets. Additionally, genome-wide association studies (GWAS) provide a cost-effective and unbiased method for identifying genetic determinants of diseases and elucidating underlying disease mechanisms [156, 157]. Through these approaches, the integration of NPs and genomic insights holds significant potential in driving innovative drug discovery and advancing personalized medicine.
Precision medicine and AI: revolutionizing personalized healthcare
Precision medicine tailors treatments to individual variations by identifying unique patient phenotypes. Artificial intelligence (AI) analyzes complex healthcare data to empower clinician decision-making and develop personalized treatment strategies. However, challenges like data integration, security, and bias exist. Successful AI adoption requires robust data governance, advanced analytics, and collaboration among healthcare professionals and data scientists. Despite challenges, AI’s integration with precision medicine holds great promise for revolutionizing personalized healthcare and improving patient outcomes [158].
Clinical applications of NP-based therapy
NPs have gathered significant attention in clinical practice due to their diverse immunomodulatory properties and potential therapeutic benefits. For instance, curcumin has been studied for its ability to modulate immune responses, inhibit tumor growth, and enhance the efficacy of conventional cancer treatments [159–161]. EGCG, found in green tea, has been shown to inhibit immune checkpoint molecules, potentially improving the effectiveness of cancer immunotherapy [162, 163]. Compounds like quercetin, apigenin, and licorice extract have shown promising results in preclinical and clinical studies. Quercetin, abundant in fruits and vegetables, exhibits anti-inflammatory and immunomodulatory effects, making it a potential therapy for autoimmune conditions [72, 164]. Apigenin, found in parsley and chamomile, has demonstrated anti-inflammatory and immunosuppressive properties, suggesting its potential in autoimmune disease management [73, 165]. Licorice extract, containing glycyrrhizin, has been investigated for its immunomodulatory effects and potential therapeutic applications in autoimmune diseases [49, 166].
Challenges and considerations for clinical implementation
The clinical integration of NPs is promising but encounters numerous hurdles and factors to consider. These include grasping the intricate chemistry of herbal products and their formulation for therapeutic purposes in humans, ensuring the translation of fundamental scientific findings into clinical safety and effectiveness, establishing a framework for gathering evidence to validate traditional herbal medicines, and establishing a regulatory system that supports research funding and safeguards intellectual property rights. Additionally, there’s a need to develop standard operating procedures for conducting clinical trials on herbal medicines. The integration of NPs into clinical practice offers promising opportunities for enhancing immune-related disorder management. However, addressing challenges such as standardization, safety concerns, regulatory approval, and patient education is essential for realizing the full potential of NP-based therapies in personalized medicine.
The journey from preliminary findings on the immunomodulatory capabilities of NPs to their formulation into evidence-based targeted therapeutics is fraught with challenges. A significant impediment is the lack of uniform research protocols to assess efficacy, safety, and action mechanisms, leading to inconsistent outcomes. Additionally, the inherent complexity and heterogeneity of NPs hinder the identification of specific bioactive constituents responsible for immunomodulatory actions, obscuring pharmacological profiles and optimal dosing. Further complicating the translation process is the incomplete understanding of the mechanisms of action in immunomodulation, necessitating detailed investigations to lay down a robust scientific groundwork. Moreover, navigating the intricate regulatory framework and ensuring stringent quality control are pivotal in the drug development process to guarantee safety, efficacy, and consistent quality. In summary, while the potential of NPs in immunomodulation is promising, their development into targeted drugs requires meticulous research, standardization, and regulatory compliance for successful integration into evidence-based medicine [167–169].
NPs, continue to play a dominant role in modern drug development. Nobel laureate Youyou Tu’s research on artemisinin [170] sparked renewed interest in traditional medicine and NPs, leading to groundbreaking developments such as the Moringa oleifera tablets for type 2 diabetes [171] and icariin softgels for HCC [172]. However, challenges persist, including issues with solubility, bioavailability, and stability, driving research in nanotechnology for novel dosage forms. Understanding drug targets is crucial for innovative drug discovery, particularly in the context of NPs which pose unique challenges in target identification.
The technological advancements in clinical research hold significant promise for revitalizing NP-based drug discovery across various domains. NPs have historically been used in combating infectious diseases, particularly as antibiotics. Leveraging cutting-edge techniques, such as harnessing the human microbiome for novel NPs, promises to unearth potent antimicrobial compounds. Given the pivotal role of gut microbiota in health, NP-based drug discovery targeting the gut microbiome emerges as a promising frontier [173, 174]. However, this area is still in its nascent stages, with many unanswered questions. Computational tools play a crucial role, in facilitating NP discovery through omics, chemical, and pharmacological analysis, as well as the integration of diverse datasets for NP-based drug discovery and development.
Conclusions
In conclusion, NPs persist as a fertile ground for discovering different formulations with diverse bioactivities, serving as both direct candidates for drug development and starting points for optimization into novel therapeutics for various diseases. Despite the ongoing challenges in drug development, NPs face additional hurdles related to accessibility, sustainable supply, and intellectual property constraints. However, scientific and technological progress lays a strong foundation for NP-based drug discovery to continue making significant steps in enhancing human health and longevity.
Abbreviations
AI: | artificial intelligence |
B. vulgaris: | Berberis vulgaris |
C. nutans: | Clinacanthus nutans |
EGCG: | epigallocatechin gallate |
HCC: | hepatocellular carcinoma |
NPs: | natural products |
TAM: | tamoxifen |
Declarations
Acknowledgments
We gratefully acknowledge the financial support provided by the Department of Health Research (DHR), Government of India, Women Scientist Scheme (File No.R.12013/21/2022-HR dated 30/03/2022), which enabled the completion of this review paper. Additionally, we extend our gratitude to the University of Kerala for its institutional support. We sincerely appreciate the resources and facilities provided by both institutions that contributed to the successful completion of this work.
Author contributions
MGP: Conceptualization, Writing—original draft, Writing—review & editing. HA: Writing—review & editing, Supervision. Both authors read and approved the submitted version.
Conflicts of interest
The authors declare that they have no conflicts of interest.
Ethical approval
Not applicable.
Consent to participate
Not applicable.
Consent to publication
Not applicable.
Availability of data and materials
Not applicable.
Funding
This study was supported by Women Scientist Scheme, Department of Health Research (DHR), Ministry of Health & Family Welfare, Government of India [File No.R.12013/21/2022-HR]. The funders had no role in study design, data collection and analysis, decision to publish, or preparation of the manuscript.
Copyright
© The Author(s) 2024.