Abstract
The excessive use of antibiotics to treat bacterial infectious diseases in all living beings has caused a global epidemic of bacterial resistance to antibiotics, leading to the emergence of multidrug-resistant and pandrug-resistant strains. In 2019, the World Health Organization (WHO) reported that antimicrobial resistance causes at least 700,000 deaths per year worldwide. Therefore, in this global war against microorganisms, a therapeutic alternative is necessary to help us win this battle. A key in this race against the clock could be lactoferrin (Lf), a cationic glycoprotein of the mammalian innate immune system that is highly conserved among mammals. Lf is a multifunctional glycoprotein with immunomodulatory, anticarcinogenic, wound-healing, antioxidant, antimicrobial, and bone regeneration properties, in addition to improving the gut microbiota. Lf limits the growth of microorganisms through the sequestration of iron but can also interact directly with some components of the outer membrane of Gram-negative bacteria or bind to teichoic acids in Gram-positive bacteria, destabilizing the membrane and resulting in lysis. Moreover, cleavage of the Lf molecule could promote the production of lactoferricins (Lfcins) and lactoferrampin (Lfampin) from the N-terminal end, which are known to often have stronger antimicrobial effects than the native molecule, as well as analogous peptides, such as HLopt2, which have also shown enhanced antimicrobial activity. Bovine Lf (bLf) has been approved by the US Food and Drug Administration (FDA), and the European Food Safety Authority for its use as a dietary supplement in food products. Because of its effectiveness, accessibility, low cost, and nontoxicity, Lf could be a promising alternative for preventing or treating infections in animals and humans.
Keywords
Lactoferrin, lactoferricin, bacteria, iron, multidrug-resistantIntroduction
We could currently be experiencing the next world war, a war against microbes because of an increase in antimicrobial resistance. This problem causes approximately 700,000 deaths per year, and the projections for the following years are discouraging, suggesting 10 million deaths per year, exceeding the current 8.2 million annual deaths from cancer [1]. Bacterial pathogens with greater resistance to one or more antimicrobials represent a severe global health problem.
Bacteria can show resistance to one or more classes of antimicrobials and, on this basis, can be classified as follows: multidrug-resistant bacteria (i.e., those resistant to three or more classes of antimicrobials), extensively drug-resistant bacteria (i.e., those resistant to all but one or two classes) or pandrug-resistant bacteria (i.e., those resistant to all available classes) [2]. Some bacteria acquire or develop genes that encode different biochemical mechanisms that protect them from the lethal or growth-inhibitory actions triggered by antibiotics, causing genetically determined resistance to one or more antimicrobial agents. These antimicrobial resistance genes are passed on to subsequent generations and can eventually be horizontally transferred to other bacteria in their immediate environment [3]. Because of this problem, different alternatives have been sought to continue this fight from the trenches, and a new plan is needed. Among what is available, a secret weapon could be lactoferrin (Lf). This review focuses on the different reported antimicrobial activities of Lf as a weapon to defeat bacteria. Importantly, to date, no resistance to Lf has been reported for any microorganism. The commercially available bovine Lf (bLf) has been approved by the US FDA and the European Food Safety Authority as a dietary supplement in food products.
Lactoferrin
Lf is a nontoxic cationic glycoprotein that is a component of the milk whey of mammals, with the exception of dogs and rats. Lf levels depend on the lactation phase. Compared with bovine mature milk (0.03–0.49 g/L), bovine colostrum contains high amounts of Lf (0.8 g/L), whereas, human Lf (hLf) levels are 5 g/L in colostrum and 2–3 g/L in mature milk [4, 5]. As an essential component of the innate immune system, Lf is found in secretions that cover mucosal surfaces and organs such as the lungs, kidneys, pancreas, intestine, gall bladder, liver, and prostate, as well as in secretions or fluids such as saliva, tears, sperm, cerebrospinal fluid, urine, bronchial secretions, vaginal discharge, synovial fluid, umbilical cord blood, and blood plasma. Lf is also produced by cells of the immune system, mainly neutrophils [6–8].
Lf has a molecular mass of 78–80 kDa, depending on its origin, and consists of a bilobal structure (N- and C-terminal lobes). Each lobe can be reversibly bound to a Fe3+ ion [9]. The molecule without iron remains in an open conformation called apo-Lf; when it binds one or two iron atoms, the molecule adopts closed states called monoferric Lf or holo-Lf, respectively. The N-terminal region functions as a serine-protease domain [10, 11], and this region also has antimicrobial properties [12]. Upon protease digestion, Lf provides a series of antimicrobial peptides (AMPs) named lactoferricins (Lfcins). Almost 300 AMPs have been isolated from the Lf sequence [13]. Some examples from bLf are Lfcin 1–11, which includes the first eleven amino acid residues APRKNVRWCTI, Lfcin B-short sequence (FKCRRWQWRMKKLG), Lfcin B-long sequence (FKCRRWQWRMKKLGAPSITCVRRAF) and lactoferrampin (Lfampin) (DLIWKLLSKAQEKFGKNKSR) from the N-terminus, which are known for having a stronger antimicrobial effect than the parental molecule [14–16]. In addition, an analogous peptide from hLf has been synthesized, named HLopt2 (CFQWKRAMRKVR), with Gln24 and Asn26 substituted for Lys and Ala, respectively; this peptide has also shown antimicrobial activity, causing a loss of membrane potential, followed by irreversible damage to bacterial cells. Furthermore, the conjugation of a drug with peptides improves antimicrobial activity, highlighting the important role of peptide-drug conjugates in drug delivery [13]. Lf is a multifunctional molecule with various effects, including antiinflammatory, immunomodulatory, antioxidant, antibacterial, antiviral, antiparasitic, antifungal, anticarcinogenic, neuroprotective, bone-repairing, and wound-healing properties, in addition to maintaining the intestinal microbiota and protecting against lifestyle diseases, such as obesity, high blood pressure, hyperlipidemia, diabetes, and stress-related emotional disorders [17–21].
Lf at the head of the battle against bacteria
The antimicrobial activity of Lf was first related to its ability to chelate iron and, thus, inhibit bacterial growth by scavenging this essential nutrient, resulting in a bacteriostatic effect. However, Lf also expresses antimicrobial activity via iron-independent pathways through direct interaction with membrane components or bacterial virulence factors. Lf and its derived peptides not only have a large repertoire of antibacterial effects against Gram-positive and Gram-negative bacteria but can also potentially be used as natural therapeutic alternatives in human and veterinary medicine. In Gram-negative bacteria, these molecules act on lipopolysaccharides (LPS), whereas, in Gram-positive bacteria, they act on lipoteichoic and teichoic acids, triggering a bactericidal effect. In addition, studies have indicated that Lf and its derived peptides lead to depolarization of the cell membrane without causing lysis of the cells, exerting their initial bactericidal effect by acting on the bacterial cell surface and subsequently on the cytoplasmic content [22–24]. Importantly, Lf has the ability to synergize with antibiotics and other drugs, allowing us to obtain a product with high efficiency in the treatment of patients. Lf could be a perfect adjuvant that helps minimize the toxic doses of antibiotics while simultaneously exerting its anti-inflammatory effects [25–27].
Lf against bacterial virulence factors
Bacterial virulence factors contribute to bacterial pathogenicity; they include capsules, toxins, enzymes, exopolysaccharides, LPS, adhesins, and other molecules that help bacteria evade host immune responses, colonize, persist, and cause disease [28]. A major component of the outer membrane of Gram-negative bacteria that confers pathogenicity is LPS (an endotoxin), whose structure consists of lipid A, a core oligosaccharide, and an O-antigen/O-polysaccharide. LPS provides structural integrity and permeability to the membrane barrier, protecting bacterial cells against antibacterial molecules [29]. Although LPS is a potent endotoxin, Lf can affect this virulence factor; for example, the direct binding of Lf to lipid A of LPS in clinically relevant bacterial species has been shown [30]. Additionally, human apo-Lf alters the structure of the outer membrane and causes LPS release in Escherichia coli and Salmonella enterica serovar Typhimurium strains through its iron-chelating activity, as, when iron is added, the effect is reversed [31]. This effect has also been shown through the binding of bLf [32] or hLf peptides to LPS in E. coli [33].
Bacteria have developed diverse ways to transport proteins from the cytoplasm throughout bacterial compartments and/or outside the cell, as well as to export small molecules such as antibiotics and toxins, known as protein secretion systems [34]. However, it has been demonstrated that hLf causes the loss and degradation of the type III secretion system in Shigella and E. coli [35] or the type IV secretion system in Helicobacter pylori [36]. The secretion of enzymes is a virulence mechanism, for example, bacterial proteases help with cell survival, replication, and physiology; secreted proteases can destroy host tissues by cleaving host proteins [37]. Lf has been shown to target bacterial proteases with effects such as the cleavage of the IgA protease precursor of Haemophilus influenzae [38], the cleavage of a cysteine proteinase from Porphyromonas gingivalis [39], the elimination of the Lf proteolytic activity of Actinobacillus pleuropneumoniae [22], and the inhibition of a 100 kDa protease of Mannheimia haemolytica [40]. However, if bacteria evade host immune responses, they can reach host cells and attach to them.
Once bacteria adhere to host cells, they can colonize and cause disease; the elimination of bacterial adhesion is essential; importantly, Lf has a role in inhibiting the adhesion of many bacteria to host cells, such as enteropathogenic E. coli (EPEC) to HeLa cells [41], Streptococcus mutans to saliva-coated hydroxyapatite beads (using bLf and bLf polypeptides) [42], and Streptococcus gordonii single cells and coaggregates to glass slides [43], and titanium surfaces [44]. The importance of avoiding bacterial adherence is not only related to inhibiting bacterial colonization, because bacteria that accomplish adhesion to host cells are able to persist in a 3D arrangement known as a biofilm, representing another virulence mechanism. Biofilms are bacterial aggregates embedded in a self-produced matrix of exopolysaccharides that confer protection against harsh environments, such as extreme temperatures and pH, high pressure, a lack of nutrients, and the presence of antibodies, bacteriophages, and many antibiotics [45]. Although biofilms are difficult architectures to abolish and penetrate for many antibiotics, they are not an obstacle for Lf, as there is much evidence that Lf decreases biofilm formation by inhibiting the initial attachment of bacteria such as Bacteroides fragilis and Bacteroides thethaiotaomicron on microtiter plates [46], Streptococcus agalactiae to human gestational membrane biopsies [47, 48], Streptococcus sanguinis on titanium surfaces [49], Pseudomonas aeruginosa on polyvinylchloride microtiter plates [50] and glass coverslips [51], P. gingivalis and Prevotella intermedia on polyvinylchloride microtiter plates [39, 52], and Acinetobacter baumanii, and enteroaggregative E. coli (EAEC) on microtiter plates [53, 54]. Furthermore, Lf also disaggregates mature biofilms such as in Streptococcus pneumoniae through its DNase activity [55]. Figure 1 and Table 1 summarize the effects of Lf on different bacterial virulence factors and mechanisms.
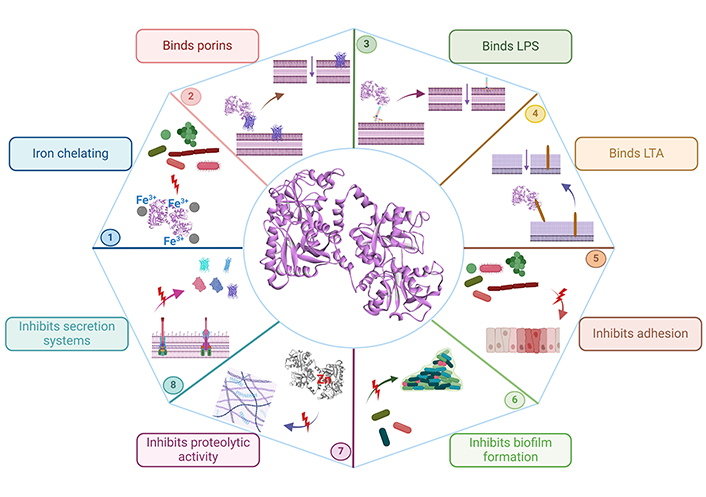
Schematic representation of the effects of Lf on bacterial virulence factors and mechanisms. The iron-chelating activity of Lf inhibits bacterial growth (bacteriostatic effect) (1), The binding of Lf to porins (2), or LPS (3) in Gram-negative bacteria, and lipoteichoic acid (LTA) (4) in Gram-positive bacteria causes membrane cell permeability (bactericidal effect), Lf inhibits the adhesion to host cells (5), biofilm formation (6), proteolytic activity (7), and secretion systems (8) of many bacteria. Lf: lactoferrin; LPS: lipopolysaccharides. Created in BioRender. Ruiz, L. (2024) BioRender.com/s75x068
Effect of Lf on bacterial virulence factors
Virulence factor | Lf | Pathogen | Effect | Ref. |
---|---|---|---|---|
LPS | hLf | E. coliK. pneumoniaeP. aeruginosa | Decreases endotoxicity by binding to LPS. | [30] |
hLfbLfLfcin | E. coliS. typhimurium | Releases LPS and alters outer membrane (OM) permeability. | [31, 32] | |
hLf | E. coli | Destabilizes OM by binding to LPS. | [33] | |
Secretion systems | hLf | ShigellaE. coli EPEC | Degradation of proteins associated to the type III secretion system. | [35] |
rLf | H. pylori | Decreases expression of the oncogenic cag pathogenicity island-encoded type IV secretion system. | [56] | |
Protease secretion | hLf | H. influenzae | Cleavage within the helper region of IgA (IgAβ) domain. | [38] |
bLf | P. gingivalis | Inhibits the activity of the cysteine proteases. | [39] | |
bLf | A. pleuroneumoniae | Inhibits activity of metalloproteases. | [22] | |
bLf | M. haemolytica | Inhibits secretion and activity of cysteine and metalloproteases. | [40, 57] | |
Adhesion | hLf | E. coli EPEC | Inhibits adhesion to HeLa cells. | [41] |
bLfbLf polypeptides | S. mutans | Inhibits adhesion to saliva-coated hydroxyapatite. | [42] | |
bLfrhLf | S. gordonii | Inhibits attachment to glass disks.Inhibits adhesion to titanium surfaces. | [43][44] | |
Adhesion and biofilm formation | rhLfbLfcinB | B. fragilisB. thethaiotaomicron | Inhibits binding to laminin and biofilm formation. | [46] |
hLf | S. agalactiae | Inhibits adherence to human gestational membranes and biofilm formation. | [47, 48] | |
hLf peptide | S. sanguinis | Inhibits adhesion to titanium surfaces and reduces early stages of biofilm formation. | [49] | |
Biofilm formation | bLf | P. aeruginosa | Inhibits biofilm formation and reduces preformed biofilm. | [50] |
bLfcinbLfampinbLfchimera | P. aeruginosa | Decreases biofilm formation. | [51] | |
bLfhLf | P. gingivalisP. intermedia | Abolishes biofilm formation and reduces preformed biofilm. | [39, 52] | |
hLfbLf | A. baumanii | Inhibits biofilm formation. | [53] | |
bLf | E. coli EAEC | Decreases biofilm formation. | [54] | |
bLf | S. pneumoniae | Eradicates form biofilm and disrupts eDNA. | [55] |
Lf: lactoferrin; LPS: lipopolysaccharides; hLf: human Lf; bLf: bovine Lf; Lfcin: lactoferricin; rLf: recombinant Lf; EPEC: enteropathogenic E. coli; rhLf: recombinant human Lf; bLfcinB: bovine Lfcin B; bLfcin: bovine Lfcin; bLfampin: bovine lactoferrampin; bLfchimera: bovine Lf chimera; EAEC: enteroaggregative E. coli; A. baumanii: Acinetobacter baumannii; A. pleuroneumoniae: Actinobacillus pleuropneumoniae; B. fragilis: Bacteroides fragilis; B. thethaiotaomicron: Bacteroides thetaiotaomicron; E. coli: Escherichia coli; H. influenza: Haemophilus influenza; H. pylori: Helicobacter pylori; K. pneumoniae: Klebsiella pneumoniae; M. haemolytica: Mannheimia haemolytica; P. aeruginosa: Pseudomonas aeruginosa; P. gingivalis: Porphyromonas gingivalis; S. agalactiae: Streptococcus agalactiae; S. gordonii: Streptococcus gordonii; S. mutans: Streptococcus mutans; S. pneumoniae: Streptococcus pneumoniae; S. sanguinis: Streptococcus sanguinis; S. typhimurium: Salmonella typhimurium
Lf (mainly of bovine origin) is used to combat bacterial infections because it is easily available and inexpensive, and has no toxicity; in addition, no bacterial resistance has been reported. This may be related to the mechanism of action of Lf, which differs from that of antibiotics, whereby antibiotics need to penetrate bacterial cells to exert their effects and can be eliminated by efflux transporters, whereas, in addition to its iron-chelating ability, Lf interacts with and alters bacterial cell membranes, as well as block efflux transporters [18]. Lf has been administered as formula in infants, where an improvement of iron absorption in the Lf-fortified formula group was found compared to the control (no Lf administered) [58]. Furthermore, some reports have shown the efficacy of orally administered Lf in patients to combat bacterial infections, such as a randomized controlled trial that evaluated the effectiveness of bLf from iron-fortified formulas on respiratory tract infections [59], a randomized double-blind, placebo-controlled study that revealed the ability of bLf to suppress H. pylori colonization (bacterium associated with the development of chronic gastritis, peptic ulcer diseases, mucosa-associated lymphoid tissue lymphoma, and gastric cancer) [60], and a clinical trial that demonstrated the ability of bLf to decrease group A Streptococcus invasion (a pathogen that causes pharyngitis, impetigo, cellulitis, and deeper infections such as rheumatic fever, scarlet fever, necrotizing fasciitis, and streptococcal toxic shock syndrome) [61]. No side effects were reported when Lf was administered in these clinical trials. Furthermore, it has been designated by the US FDA as a food additive that is generally recognized as safe (GRAS) [62].
Conclusions
We cannot avoid the existence of bacteria and we do not want to; however, the presence of resistant pathogenic bacteria is disturbing. Pathogenic bacteria have developed strategies to avoid host immune responses and persist as pathogens, but we do have weapons to combat these pathogens. There is much evidence that “the miracle protein”, Lf, is one such weapon, as it is able to combat every stage of bacterial colonization. Accordingly, Lf has renewed hope that we can win this battle, and we are very close to achieving this goal.
Abbreviations
AMPS: | antimicrobial peptides |
bLf: | bovine lactoferrin |
FDA: | Federation Drugs Administration |
hLf: | human lactoferrin |
Lf: | lactoferrin |
Lfampin: | lactoferrampin |
Lfcins: | lactoferricins |
LPS: | lipopolysaccharides |
Declarations
Author contributions
GRR and LRM: Conceptualization, Investigation, Project administration, Supervision, Visualization, Writing—original draft, Writing—review & editing. MdlG: Conceptualization, Supervision, Writing—review & editing.
Conflicts of interest
The authors declare that they have no conflicts of interest.
Ethical approval
Not applicable.
Consent to participate
Not applicable.
Consent to publication
Not applicable.
Availability of data and materials
Not applicable.
Funding
Not applicable.
Copyright
© The Author(s) 2024.