Abstract
A very new and highly specialized category of radiotracers that is still growing is radiolabeled peptides. Radiolabeled peptides, or radiopeptides, are powerful elements for diagnostic imaging and radionuclide therapy. These laboratory-manufactured peptides have gained attention due to their unique properties. The tiny structure of these peptides compared to proteins and antibodies makes them favorable regarding their availability through simple synthesis from amino acids, easy uptake by receptors on cancer cells, and high specificity and affinity for high-quality and accurate radio imaging. This study highlighted the potential of technetium-99m-labeled peptides in advancing diagnostic capabilities in directed research in Latin America.
Keywords
Technetium-99m, peptide, solid phase synthesis, imaging, radio imagingIntroduction
In the early 2010s, Bolzati et al. [1, 2] reviewed the development of technetium-99m (99mTc)-labeled radiopharmaceuticals, especially radiopeptides, in terms of their usage in radiotherapy and tumor imaging. The metastable nuclear isomer of Tc, 99mTc, has a half-life (T½) of 6 h and emits gamma rays with 140 keV photon energy. The energy of 99mTc rays is sufficient to penetrate the biological tissues, however, the extremely short T½ of 99mTc makes it impossible to store or transport it. Meanwhile, producing this radionuclide through bombardment of 100Mo in cyclotrons is not preferred [3], because it yields the undesired 99gTc. Indeed, the development of 99mTc generators has been crucial for widespread clinical use, which uses the parent 99Mo nuclide (T½ of 66 h) obtained from highly enriched uranium [4, 5]. Correspondingly, the nuclear features of 99mTc make it perfect for use in diagnostic nuclear medicine [6]. Due to its artificial and metallic nature, a pharmacophore should be attached to chelating groups to construct 99mTc-labeled radiopharmaceuticals. The pharmacophore group plays a critical role in targeting specific tissues during imaging. Furthermore, the selected chelating group must be highly reactive toward 99mTc to construct an extremely stable complex [7]. In the published minireview in 2019, Miranda et al. [8] explored the radiochemical quality control of 99mTc-radiopharmaceuticals. They focused on optimizing chromatographic systems for assessing the radiochemical purity (RCP) of 99mTc-eluate and radiopharmaceuticals. Recently, Duatti [6] listed the development of 99mTc-based radio imaging using single photon emission tomography (SPECT). The focus of this literature study is on the synthesis, complexation, purification, and application of various 99mTc-radiopharmaceuticals [6].
In the emerging arena of nuclear imaging, diagnosis, and therapy, peptides are now essential motifs for in vivo targeting, radio imaging, monitoring, and visualization of infected tissues for the diagnosis and treatment of diseases [9–12]. Furthermore, radiolabeled peptides can identify the overexpressed peptide-binding receptors in many malignant cells and tumors [13, 14]. Besides, there are several benefits to employing peptides as bioactive molecules in radiolabeled detectors, including low adverse effects due to the low toxicity of peptides in comparison with the other pharmacophore compounds, great affinity for the target receptors, and the ability to incorporate hydrophilic functional groups into their structure to increase excretion and decrease lipophilicity [15–17]. Decreasing lipophilicity in a radiopharmaceutical leads to rapid renal excretion rather than intestinal clearance. Moreover, high tumor-to-background ratios are obtained by radiolabeled peptides, which is a crucial factor in radio imaging to achieve effective cancer-targeting and high-quality images [18, 19]. In 2020, Mohtavinejad et al. [20] discussed essential aspects of various radiolabeled peptides, including neurotensin (NT), somatostatin (SST), arginylglycylaspartic acid (RGD), exendin, vasoactive intestinal peptide, gastrin, and bombesin (BBN), in tumor imaging and pre-clinical and clinical phases of therapy. Another study collected recently published radiolabeled peptides, which were tested on imaging animals’ organs [21].
Continuing our research on the synthesis of peptides, investigating their bioactivities, and their applicability in radio imaging [22–29], in this review we wanted to summarize the conducted studies in Latin American synthesis of special bioactive peptides, their complexation with 99mTc via special linkers and co-ligands, and their applicability in radio imaging and diagnosis of several diseases. This article covered various peptides, including BBN and its analogs, RGD peptides, NT, LyeTx I, and peptidoglycan aptamer.
99mTc-labeled peptides and their applications in radio imaging
The method of labeling peptides with 99mTc
99mTc is accessible in the 7+ oxidation state by reducing 99mTcO4– to a lower oxidation state using the stannous chloride. Ascorbic acid, as an antioxidant, is usually added to the solution of 99mTc complex to keep it stable. The pH at which the complex is stable is 7.0 because it must be used in biological tissues. Then, Tc can tightly bind to a single specific atom or a small part of the chelating group. For instance, 2-hydrazinonicotinic acid (2-HYNIC), a bifunctional chelator suitable for Tc, easily attaches to bioactive molecules through an amidification reaction [30]. Since the coordination of HYNIC is possible from the nitrogen atom of its hydrazine moiety, it cannot saturate the coordination capacity of 99mTc, thus, the use of co-ligands is essential to complete the coordination sphere. Tricine, ethylenediaminediacetic acid (EDDA), nicotinic acid, pyridine dicarboxylic acid (PDA), glucamine, mannitol, and glucoheptonic acid are commonly used co-ligands to construct 99mTc-HYNIC complexes [31]. Figure 1 illustrates the process of binding HYNIC to a bioactive molecule and subsequently forming complexes with 99mTc using co-ligands. Studies showed that tricine and EDDA give the most stable radiochemical complex even up to one day post-incubation [32]. Therefore, HYNIC has been recently considered to bind to antibodies, fatty acids, proteins, and peptides via the formation of amide bonds, followed by coordinating with 99mTc to be used for radio imaging, diagnosing, and monitoring various targets and diseases, including activated T lymphocytes, pancreatic neuroendocrine neoplasms, cancer, and carcinoma cells [33–40].
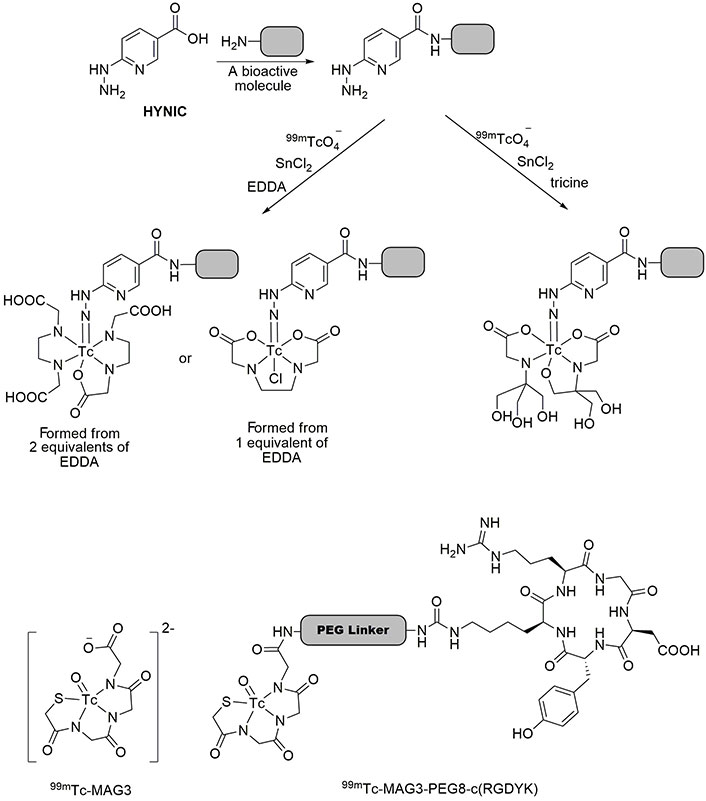
Synthesis of 99mTc-HYNIC complexes. The mentioned bioactive molecule can be any biologically active compound that has an amino group. 99mTc: technetium-99m; EDDA: ethylenediaminediacetic acid; HYNIC: hydrazinonicotinic acid; MAG3: mercaptoacetyltriglycine
99mTc-labeled BBN in detection of tumor cells
BBN is a 14-amino-acid peptide (pGlu-Gly-Arg-Leu-Gly-Thr-Gln-Trp-Ala-Val-Gly-His-Leu-Met-NH2) that binds to G protein-coupled receptors (GPRs). The latter is overexpressed in different kinds of human cancer cells, especially breast, lung, and prostate cancers (PCs) [41]. Faintuch et al. [42] prepared a series of 99mTc-HYNIC-linker-BBN to compare their biodistribution and scintigraphy imaging in mice bearing PC-3 tumor cells. They found that 99mTc-HYNIC-βAla-BBN is rapidly produced during the radiolabeling step with no need for purification, bears high radiochemical efficiency with more with internalization (12% within 30 min) and tumor uptake [32, 42–44]. Inspired by the mentioned research, de Barros et al. [45] prepared a pH-sensitive liposome encapsulating 99mTc-HYNIC-βAla-BBN(7–14) and used it to detect human breast cancer. The prepared nano-liposome with an approximately 165 nm diameter displayed a strong signal in the tumor tissue, showing a good tumor-to-muscle of 9.31% injected dose (ID)/g. It was also enclosed that 99mTc-HYNIC-βAla-BBN(7–14) can recognize Capan-1 pancreatic adenocarcinoma at its early stage with an uptake of 0.47% ID/g [46], and LNCaP prostate tumor [47]. Another research revealed the applicability of 99mTc-HYNIC-BBN or imaging women’s breasts with malignant tumors [48]. Later, Faintuch et al. [49] could design a 99mTc-mercaptoacetyltriglycine (MAG3) complex coupled to BBN via a 6-aminohexanoic acid (6-Ahx) linker (99mTc-MAG3-Ahx-BBN). The RCP of this complex was approximately 96% at neutral pH, exhibiting high internalization (75% within 30 min) and great affinity for BBN receptors [49]. Following their research, Faintuch et al. [50] compared the ability of 99mTc-MAG3-Ahx-BBN and 99mTc-MAG3-Ahx-DUP1 to diagnose prostate carcinoma. DUP1 is a synthetic peptide (Phe-Arg-Pro-Asn-Arg-Ala-Gln-Asp-Tyr-Asn-Thr-Asn) with high affinity for DU-145 prostate and PC-3 cells [51]. The mentioned study demonstrated that the DUP1 tracer was more hydrophilic than the BBN one, with greater kidney uptake. However, due to its higher specificity to receptors of gastrin-releasing peptide, the BBN tracer displayed superior internalization (78%), while tumor uptake for both tracers was comparable [50]. Many other BBN-based 99mTc-radiotracers have been developed through similar protocols to improve stability and high uptake in target tissues [52–59]. Moreover, the focus of some research is on the preparation of multifunctional systems containing BBN-based 99mTc-radiotracers conjugated to the surface modified nanoparticles (NPs) with target-specific molecular recognition [60–64].
Pretargeting involves the use of high affinity and specificity biomarkers to obtain a high contrast of target to the background, improving the tumor-to-nontumor ratio [65–67]. Morpholino oligomers (MORFs) contain DNA bases in their scaffold attached to morpholine rings through phosphonodiamidite groups. These synthetic oligomers are widely employed for nuclear-pre-targeted imaging [68–72]. Since binding MORF to a carrier through a covalent bond is difficult, the utilization of streptavidin (SA) as a linker makes it easy to attach a biotinylated carrier to biotinylated MORF via simple mixing. Consequently, Faintuch et al. [73] prepared 99mTc-MAG3-cMORF as well as Biotin-βAla-BBN and Biotin-MORF and mixed them in the presence of SA to have a 99mTc-labeled nano-peptide to image lymph nodes bearing tumor cells.
99mTc-labeled analogs of SST
SST is a kind of cyclic peptide hormone, containing 14 or 28 amino acids, which plays its role in different ways, including interaction with G protein-coupled SST receptors to control cell proliferation, regulation of cellular functions, and inhibiting the release of other hormones, such as insulin and glucagon secretion [74–76]. The peptidases distributed in plasma and tissues rapidly degrade the natural SST, therefore, its highly short life (1–3 min) makes it useless in clinical cases [77]. Consequently, many analogs of SST have been developed regarding the clinical approaches. Lanreotide or somatuline is an SST-analogue octapeptide, containing 8 amino acids (D-Nal-Cys-Tyr-D-Trp-Lys-Val-Cys-Thr-NH2) cyclized through a disulfide bond between two cysteine moieties. Lanreotide can manage the symptoms resulting from neuroendocrine-active tumors [78]. In the early 2000s, its 99mTc complex was considered to be used in radio imaging of neuroendocrine tumors. Consequently, 99mTc-lanreotide was prepared in a tartrate-phthalate buffer solution, containing maltose, glycine, and SnCl2 solution, followed by the addition of 99mTc. This complex was stable for 6 h, mainly distributed in the gastrointestinal tract, showing its applicability in radiodiagnosis [79]. However, the study of this complex was of interest only in those years and forgotten later.
Octreotide (OCT) and octreotate (TATE) are more attractive than lanreotide in radio imaging studies for the detection of unrespectable neuroendocrine tumors. To aim for this, Melo et al. [80] developed the synthesis of 99mTc-HYNIC-Tyr3-OCT and 99mTc-HYNIC-Tyr3-TATE (Figure 2) and investigated their biodistribution. They found fast blood clearance and high biodistribution of OCT and TATE in the pancreas, intestine, stomach, lung, and blood. The great uptake of these radio-drugs in the kidney and pancreas is due to the high density of SST receptors. Moreover, low uptake was observed in bones, liver, spleen, heart, brain, and thyroid [80, 81]. In another research, OCT was loaded on Au NPs coated with Lys3-BBN or mannose (Figure 3). The prepared radio-agents were useful to detect sentinel lymph nodes [82, 83]. The time-consuming preparation of such loaded radio drugs is one of the drawbacks of this method, which limits its clinical application.
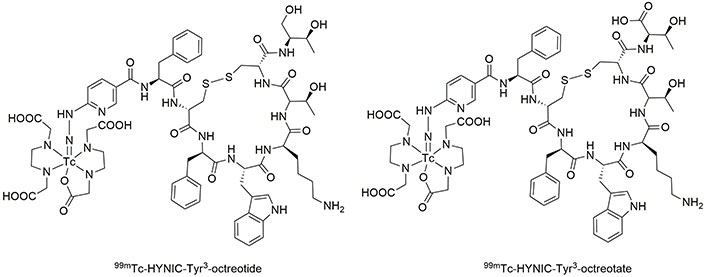
The structure of TATE and OCT. 99mTc: technetium-99m; HYNIC: hydrazinonicotinic acid; OCT: octreotide; TATE: octreotate
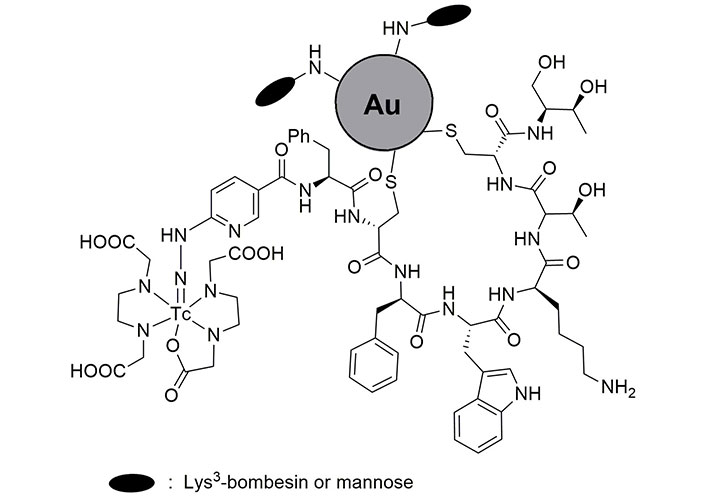
The loaded OCT on Au nanoparticles coated with Lys3-bombesin or mannose. OCT: octreotide
99mTc-labeled exendin-4 fragment
Exendin-4 is an analog of glucagon-like peptide 1 (GLP-1), which can attach to GLP-1 receptors, expressed in patients who suffer from insulinomas, a kind of small pancreatic endocrine tumor [84–86]. Currently, exendin-4 is consumed for the treatment of type 2 diabetes [87, 88]. Because radiolabeled exendin(9–39) has the potential to detect GLP-1 receptors and also OCT can identify SST receptors, a combination of HYNIC-exendin(9–39) and OCT (Figure 4) can detect malignant insulinomas pancreatic tumors, which express high and low densities of GLP-1 and SST receptors, respectively. Biodistribution studies of 99mTc-HYNIC-exendin(9–39)-OCT showed suitable uptake in target tumor cells (2.71% ID/g), blood (1.5% ID/g), and kidney (95.0% ID/g) and it was rapidly eliminated from renal after 2 h [89–92].
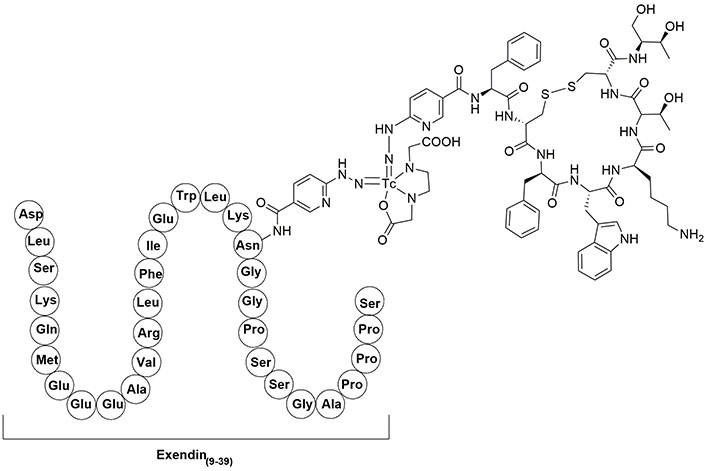
The structure of 99mTc-HYNIC-exendin(9–39)-OCT. 99mTc: technetium-99m; HYNIC: hydrazinonicotinic acid; OCT: octreotide
99mTc-labeled RGD or RGD analogues
RGD is a tripeptide comprising Arg-Gly-Asp residue, which is responsible for cell adhesion to the extracellular matrix [93]. RGD peptides have the potential to be used in tissue engineering, therapy, and imaging [94, 95]. It has been validated that some kinds of cyclic peptides containing RGD residue can bind integrin αvβ3, which is linked to the progress of various diseases [96]. It must be noted that a cyclic RGD-pentapeptide, comprising of Arg-Gly-Asp-Tyr-Lys [c(RGDyK)], is a derivative of RGD with a high affinity for αvβ3 integrin, which is expressed on the cell membrane of many cancerous cells [97, 98]. Decristoforo et al. [99] synthesized c(RGDyK) to make 99mTc-HYNIC-c(RGDyK), as depicted in Figure 5. High uptake of the prepared radiotracer was observed in αvβ3-integrin-receptor-positive M21 melanoma cells up to 2.73% ID/g, while tumor-to-organ ratios were equivalent to that observed for [18F]Galacto-RGD.
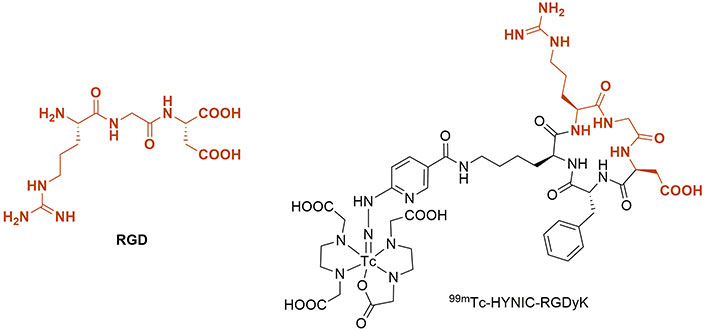
The structures of RGD tripeptide and 99mTc-HYNIC-RGDyK. 99mTc: technetium-99m; HYNIC: hydrazinonicotinic acid; RGD: arginylglycylaspartic acid
99mTc-MAG3 is frequently employed for renal and kidney function imaging [100, 101]. The carboxyl group of MAG3 can bind to a linker molecule, carrying a biomolecule. As shown in Figure 6, 99mTc-MAG3 is attached to c(RGDyK) via a polyethyleneglycol linker, forming 99mTc-MAG3-PEG8-c(RGDyK). It was revealed that 99mTc-MAG3-PEG8-c(RGDyK) traces malignant melanoma cells, SK-MEL-28, after 30 min of incubation with an internalization of 96.13%. Due to its specificity for human SK-MEL-28 cells, this radiotracer is efficient for the early diagnosis of melanoma [102, 103]. Moreover, this tracer was employed for imaging diverse tumor models, including blood, lungs, kidneys, spleen, stomach, pancreas, liver, intestine, muscle, and bone carcinoma. The results revealed its high biodistribution in lung cancer cells, presenting the applicability of 99mTc-MAG3-PEG8-c(RGDyK) tracer to detect lung tumors in addition to melanoma cells [104]. It must be noted that polyethyleneglycol protects peptides from enzymatic degradation and thus decreases proteolysis, also increases overall hydrophilicity of the compound, leading to the increased peptide T½ and stability [105]. Schiper et al. [106] prepared HYNIC-E-[c(RGDfK)]2 (Figure 7) and made its complex with 99mTc to test its biodistribution on Swiss mice infected with osteonecrosis. This radiotracer showed the highest bone uptake after 15 days and could detect bone infarction efficiently [106]. In another research, c(RGDfK) was grafted on the surface of gold NPs modified with 99mTc-HYNIC-GGC, where GGC is Gly-Gly-Cys residue. This NP showed high specificity for detection of αvβ3-integrin-receptor-positive M21 melanoma cells [107].
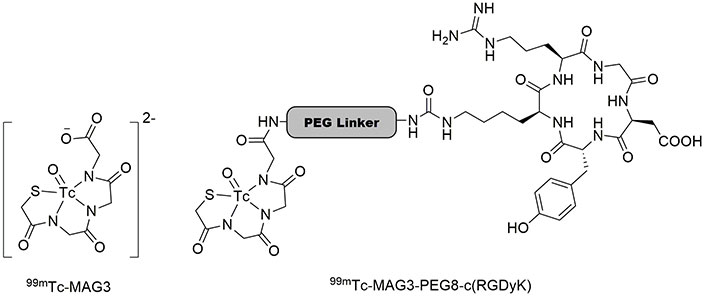
The structures of 99mTc-MAG3 and 99mTc-MAG3-PEG8-c(RGDyK). 99mTc: technetium-99m; MAG3: mercaptoacetyltriglycine
Based on the previously published method [108, 109], Caporale et al. [110] synthesized a small cyclic pentapeptide of c(RGDfV), containing cyclized Arg-Gly-Asp-D-Phe-Val, which is an analogue of c(RGDyK). Then, two complexes of 99mTc-c(RGDfV) were prepared using nitrido nitrogen atoms (Figure 8). These complexes were stable after incubation for 4 h at 37°C in biological serum [110].
GX1 (Cys-Gly-Asn-Ser-Asn-Pro-Lys-Ser-Cys) is one of the high-affinity peptides with angiogenesis that can inhibit it. Accordingly, GX1 has been widely used for targeting and imaging cancer cells because of its binding specificity to integrin α3β1 receptors [111–113]. Correspondingly, binding the RGD peptide to GX1 may improve tumor cell affinity in radio imaging. In this regard, cyclized GX1 was introduced in two complexes with 99mTc, i.e. 99mTc-HYNIC-PEG4-c(GX1) and 99mTc-HYNIC-E-[c(RGDyK)-c(GX1)] (Figure 9). Both prepared tracers showed considerable stability after 4 h remaining in human serum under physiological conditions. They had great hydrophilic features with great renal excretion, however, the clearance of 99mTc-HYNIC-PEG4-c(GX1) from the blood was faster. After 5 min of incubation (0.41%), about 51% of the latter was internalized, while 99mTc-HYNIC-E-[c(RGDyK)-c(GX1)] showed the highest binding value after 2 h of incubation (0.35%). Comparable biodistribution was observed for both tracers in most organs [114, 115]. Later, their ability in radio imaging was established, revealing better visualization, favoring tumor uptake (2.96% at 1 h), and highest binding (1.14%) at 2 h for 99mTc-HYNIC-E-[c(RGDyK)-c(GX1)] in glioma U87MG cells [116]. Moreover, magnetic resonance imaging (MRI) confirmed the specific binding of these tracers to human U87 glioblastoma in the brain [117]. Another research signified that a remarkable uptake can be obtained by injecting 99mTc-HYNIC-PEG4-c(GX1) into mice bearing B16F10 and SK-MEL-28 melanoma cells (1.41% and 2.42%, respectively) at 1 h [115].
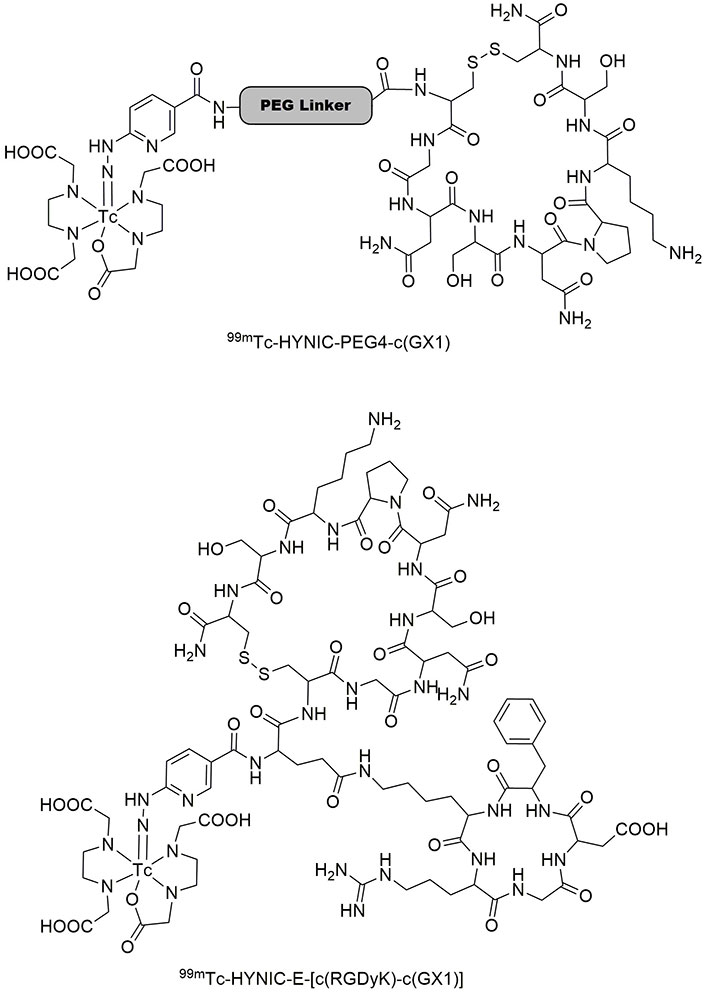
Two complexes of 99mTc-HYNIC-PEG4-c(GX1) and 99mTc-HYNIC-E-[c(RGDyK)-c(GX1)]. 99mTc: technetium-99m; GX1: Cys-Gly-Asn-Ser-Asn-Pro-Lys-Ser-Cys; HYNIC: hydrazinonicotinic acid
In another study, a hexapeptide of Gly-Arg-Gly-Asp-His-Val (GRGDHV) was synthesized as an RGD analog, and labeled with a tricarbonyl complex of 99mTc, as illustrated in Figure 10. However, the sites of complexation of peptide with 99mTc have not been determined. This complex was stable for 24 h in human serum under biological conditions. Moreover, after 1 h of incubation, 99mTc(CO)3-GRGDHV showed distinct binding in C6 tumorigenic cells with bound and internalized fractions of 22% and 34%, respectively. The accumulation of this radiopeptide was observed in the brains of glioblastoma allograft tumor-bearing rats and normal rats, showing biodistribution up to 1.57 and 0.6, respectively, at 4 h. The results showed promising application of this radiolabeled peptide in the clinical diagnosis of glioblastoma [118].
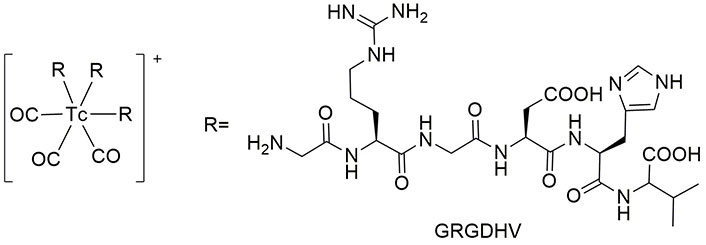
The structure of 99mTc(CO)3-GRGDHV. 99mTc: technetium-99m; GRGDHV: Gly-Arg-Gly-Asp-His-Val
99mTc-labeled NT
NT is a neuropeptide, containing 13 amino acids of pyroGlu-Leu-Tyr-Glu-Asn-Lys-Pro-Arg-Arg-Pro-Tyr-Ile-Leu, playing its role in the central nervous system through interaction with dopamine receptors, smoothing muscle contraction, and regulation of luteinizing hormone and prolactin release [119–121]. Regarding the published results for the application of NT analogues in tumor imaging [122], Teodoro et al. [123] investigated the bioactivities of NT(8–13) analogue (Arg-NMe-Arg-Pro-Tyr-Ile-Leu) using its complex 99mTc-HYNIC-βAla-NT(8–13). Considerably high uptake of this tracer was observed for the lung, blood, and kidneys, while it was rapidly eliminated from the blood. Moreover, low uptake of this tracer was detected in the liver and intestine, showing its potential to be used in tumor imaging due to its high uptake and fast clearance from the blood [123].
99mTc-labeled antibacterial peptides
The cationic peptide LyeTx I is a natural 25 amino acid peptide, isolated from Lycosa erythrognatha venom, exhibiting antimicrobial activities [124–126]. Fuscaldi et al. [127] designed two chelating agents for 99mTc using C- and N-terminus of LyeTx I and HYNIC to give LyeTx I-K-HYNIC and HYNIC-LyeTx I, respectively. Then, the bioactivities of these agents were determined against Staphylococcus aureus and Escherichia coli, and it was discovered that the latter couldn’t inhibit the growth of bacterium, while the former LyeTx I-K-HYNIC showed antibacterial properties with minimum inhibitory concentration (MIC) values of 5.05 μmol/L and 10.01 μmol/L against the mentioned species, respectively. Accordingly, 99mTc-LyeTx I-K-HYNIC complex was prepared with high purity to study infected tissues [127, 128]. In that study, researchers evaluated the biodistribution of two peptidoglycan aptamers, Antibac1 and Antibac2, which were labeled with 99mTc. These aptamers were specifically designed for bacterial infection diagnosis. The results exhibited that these tracers can easily recognize a bacterial infection focus [129].
Recently, ubiquitin (UBI) peptide is a cationic, synthetic antimicrobial peptide fragment. It has been considered a versatile antibacterial agent to be labeled with 99mTc [130–132], which is useful for distinguishing bacterial infections. The use of radiolabeled peptides for infection imaging in humans has been an area of active research. Here are some key findings from clinical studies involving 99mTc-UBI(29–41) as an infection-imaging agent [133]. In 2005, a 29–41 fragment of UBI with the sequence of Thr-Gly-Arg-Ala-Lys-Arg-Arg-Met-Gln-Tyr-Asn-Arg-Arg was labeled with 99mTc through coordination using its Lys and Arg7 amino acids. The obtained 99mTc-UBI(29–41) was injected into patients with osteomyelitis, diabetes, and fever of unknown origin. Imaging showed infected tissues, which were comparable with the results obtained by biopsy [134–136]. In another research, it was determined that the imaging results of infected tissues obtained by 99mTc-UBI(29–41) were in agreement with those recorded by 67Ga-citrate. Moreover, after 24 h, nearly 85% of 99mTc-UBI(29–41) is eliminated by renal clearance [137]. Vallejo et al. [138] used this radiotracer to detect mediastinitis after cardiac surgery. Furthermore, 99mTc-UBI(29–41) was applicable to diagnose musculoskeletal [139] and postsurgical spinal infections [140].
Discussion
Table 1 shows a summary of the peptide-99mTc complexes discussed in this review article. This table depicts their target cells, uptake amounts by the target, biodistribution of the complex in different organs, current applications, and advantages and usage of the prepared complexes.
The summary of peptide-99mTc complexes in radio imaging
Entry | Peptide-99mTc complexes | Target cells or receptors | Uptake by target (% ID/g) | Biodistribution | Current applications | Advantages | Reference |
---|---|---|---|---|---|---|---|
1 | 99mTc-HYNIC-βAla-BBN(7–14) | BBN-positive tumor cells, Capan-1 pancreatic adenocarcinoma | 0.47–9.31 | Tumors, spleen, the liver, and muscles | Imaging of breast tumors | Rapid clearance by renal excretion, higher uptake by tumors | [45, 46] |
2 | 99mTc-MAG3-Ahx-DUP1 | DU-145 prostate and PC-3 cells | 1.23 | Tumors, pancreas, spleen, lung, liver, and kidney | Diagnosis of prostate carcinoma | Rapid clearance | [50] |
3 | 99mTc-MAG3-cMORF | PC-3 cells | 2.58 | Tumors, kidney, intestines, and liver | Imaging of lymph nodes bearing tumor cells | High tumor uptake | [73] |
4 | 99mTc-lanreotide | Neuroendocrine-active tumors | Not determined | Intestine, kidney, lung, and liver | Imaging of neuroendocrine tumors | - | [79] |
5 | 99mTc-HYNIC-Tyr3-OCT | Somatostatin receptors | 1.65–19.12 | Pancreas, intestine, stomach, lung, and blood | Imaging of sentinel lymph nodes | High somatostatin receptor uptake | [80, 81] |
6 | 99mTc-HYNIC-Tyr3-octreotate | Somatostatin receptors | 1.0–26.0 | Pancreas, intestine, stomach, lung, and blood | Imaging of sentinel lymph nodes | High somatostatin receptor uptake | [80, 81] |
7 | 99mTc-HYNIC-exendin(9–39)-OCT | GLP-1 receptors | 2.71 | Tumors, blood, and kidney | Detection of malignant insulinomas pancreatic tumors | Rapid clearance | [89–92] |
8 | 99mTc-HYNIC-c(RGDyK) | αvβ3-integrin-receptor-positive M21 melanoma cells | 2.73 | Tumors, intestine, and kidney | Imaging of integrin αvβ3 in coronary arterial and peripheral vascular angiogenesis | Low blood retention, low liver, and muscle uptakes | [99] |
9 | MAG3-PEG8-c(RGDyK) | SK-MEL-28 cells | 7.85 | Tumors, intestine, liver, and kidney | Early diagnosis of malignant melanoma | Stable internalizationuntil 120 min | [102, 103] |
10 | 99mTc-HYNIC-E-[c(RGDfK)]2 | Severely devascularized bone | 4.2 | Bone | Imaging of osteonecrosis | Remarkable renal excretion | [105] |
11 | 99mTc-HYNIC-GGC-Au NPs | αvβ3-integrin-receptor-positive M21 melanoma cells | 8.18 | Tumors, pancreas, liver, and kidney | Imaging of tumor αvβ3 expression | High spatial resolution | [106] |
12 | 99mTc-HYNIC-PEG4-c(GX1) | Glioma U87MG cells | 1.52 | Tumors, blood, liver, kidney, and intestines | Targeting angiogenesis in glioma tumors | - | [113–115] |
13 | 99mTc-HYNIC-E-[c(RGDyK)-c(GX1)] | Glioma U87MG cells | 2.96 | Tumors, blood, liver, kidney, and intestines | Targeting angiogenesis in glioma tumors | Great renal excretion | [113–115] |
14 | 99mTc(CO)3-GRGDHV | C6 tumorigenic cells | 1.57 | Brain, heart, spleen, lung, liver, and kidney | Imaging of tumor αvβ3 expression | Hydrophilic character | [117, 118] |
15 | 99mTc-HYNIC-βAla-NT(8–13) | Tumor cells | > 18.1 | Tumors, lung, blood, and kidneys | Tumor imaging | Low uptake in liver and intestine, high uptake, and fast clearance from the blood | [122, 123] |
16 | 99mTc-LyeTx I-K-HYNIC | Bacterial infection | - | Not determined | Infection imaging | - | [128] |
17 | 99mTc-UBI(29–41) | Infected tissues | - | Not determined | Diagnosis of musculoskeletal and postsurgical spinal infections | - | [136–139] |
-: no data. 99mTc: technetium-99m; Ahx: aminohexanoic acid; BBN: bombesin; GLP-1: glucagon-like peptide 1; GRGDHV: Gly-Arg-Gly-Asp-His-Val; GX1: Cys-Gly-Asn-Ser-Asn-Pro-Lys-Ser-Cys; HYNIC: hydrazinonicotinic acid; ID: injected dose; MAG3: mercaptoacetyltriglycine; MORF: morpholino oligomer; NPs: nanoparticles; NT: neurotensin; OCT: octreotide; PC-3: prostate cancer-3
Conclusions
Radio imaging is an essential technique to monitor and diagnose a wide variety of diseases, including cancer, Alzheimer’s, and infections. The emission of gamma rays with a T½ of 6 h and 140 keV photon energy makes 99mTc a valuable metastable nuclear isomer to be used in SPECT. To this aim, 99mTc is tightly binding to a chelating group, such as HYNIC, and co-ligands. The use of 99mTc-labeled peptides for tumor imaging has gained significant attention in clinical research. These radiolabeled peptides offer advantages such as high specificity, favorable pharmacokinetics, and minimal radiation exposure. The chelating group is attached to a bioactive molecule, which possesses affinity for a specific receptor. In this review, we showed different kinds of bioactive peptides labeled with 99mTc, which are useful in the clinical detection of prostate, pancreas, lung, and stomach tumors. The application of 99mTc-labeled antibacterial peptides holds promise for detecting infected tissues and tracing bacterial function. In summary, 99mTc-labeled peptides hold promise for non-invasive disease imaging, and ongoing research aims to improve their clinical impact.
Abbreviations
2-HYNIC: | 2-hydrazinonicotinic acid |
6-Ahx: | 6‐aminohexanoic acid |
99mTc: | technetium-99m |
BBN: | bombesin |
EDDA: | ethylenediaminediacetic acid |
GLP-1: | glucagon-like peptide 1 |
GRGDHV: | Gly-Arg-Gly-Asp-His-Val |
GX1: | Cys-Gly-Asn-Ser-Asn-Pro-Lys-Ser-Cys |
ID: | injected dose |
MAG3: | mercaptoacetyltriglycine |
MORFs: | morpholino oligomers |
NPs: | nanoparticles |
NT: | neurotensin |
OCT: | octreotide |
PCs: | prostate cancers |
RCP: | radiochemical purity |
RGD: | arginylglycylaspartic acid |
SA: | streptavidin |
SPECT: | single photon emission tomography |
SST: | somatostatin |
T½: | half-life |
TATE: | octreotate |
UBI: | ubiquitin |
Declarations
Acknowledgments
We gratefully acknowledge Iran National Science Foundation (INSF, grant no 99029802) for the financial support.
Author contributions
VFV: Conceptualization, Investigation, Writing—original draft, Writing—review & editing. SB: Conceptualization, Investigation, Writing—original draft, Writing—review & editing, Funding acquisition.
Conflicts of interest
Both authors declare that they have no conflicts of interest.
Ethical approval
Not applicable.
Consent to participate
Not applicable.
Consent to publication
Not applicable.
Availability of data and materials
Not applicable.
Funding
This study was funded by Iran National Science Foundation (INSF) [99029802]. The funder had no role in study design, data collection and analysis, decision to publish, or preparation of the manuscript.
Copyright
© The Author(s) 2024.