Abstract
Bladder cancer (BC) is a complex disease with multiple clinical manifestations and treatment challenges, and current standard-of-care therapies remain limited and unfavorable. Theranostics, the integration of diagnostic and therapeutic technologies, has emerged as a promising strategy to address these challenges. The rapid development of nanomedicine has been a source of hope for the improvement of BC therapies and diagnostics by reducing side effects, enhancing tumor suppression, and overcoming drug resistance. Metal nanoparticles (NPs), inorganic NPs, polymer NPs, etc. have their respective advantages and show encouraging potential in the therapy of BC. In this review, we provide an overview on the state of the art in nanotechnology-based theranostics for BC, offering insights into the design and discovery of novel NPs for future BC management.
Keywords
Bladder cancer, theranostics, nanoparticles, diagnosis, therapyIntroduction
Bladder cancer (BC) is the 10th most common cancer in the world, with an estimated 573,000 new cases and 212,000 deaths in 2022 [1]. It is more common in males and the elderly, with the highest incidence rates in Europe and North America [2]. Tobacco smoking is the most important modifiable risk factor, accounting for about half of all cases of BC. Other risk factors include occupational exposure to certain chemicals, chronic bladder infections, and genetic susceptibility [3]. Early diagnosis and treatments are crucial for improving BC outcomes. The mainstay of BC treatment includes transurethral resection of bladder tumor (TURBT), intravesical therapy, radical cystectomy, and systemic chemotherapy [4, 5]. TURBT is the standard treatment for non-muscle invasive BC, while radical cystectomy is for muscle invasive BC. Intravesical therapy, such as chemotherapy and immunotherapy, is used to reduce the risk of recurrence after TURBT [6]. Systemic chemotherapy is the preferred treatment for advanced or metastatic BC.
In addition to surgery, chemotherapy, and radiation therapy are commonly adjuvant approach to treat BC [7, 8]. Chemotherapy is used as neoadjuvant therapy before cystectomy or as adjuvant therapy after surgery to improve overall survival. It can also be used as systemic therapy for advanced or metastatic BC. Radiation therapy is used for bladder preservation or as adjuvant therapy after surgery in certain cases. Moreover, immunotherapy has emerged as a promising approach for the treatment of BC by harnessing the patient’s immune system to target cancer cells [9]. Bacillus Calmette-Guérin (BCG) immunotherapy, such as atezolizumab, pembrolizumab, and nivolumab, is generally accepted in the intravesical therapy of non-muscle invasive BC, which has been approved for advanced and metastatic BC therapy [10–12].
Surveillance is a crucial aspect of BC management involving close monitoring to detect any recurrence or new tumors [13]. This includes cystoscopy, a minimally invasive procedure that provides a direct visualization of bladder, as well as imaging studies such as computed tomography (CT) scans and magnetic resonance imaging (MRI) [14–16]. Surveillance schedules are individualized based on the patient’s tumor characteristics, stage, and treatment history. In summary, BC treatment requires a multidisciplinary approach that incorporates surgery, chemotherapy, radiation therapy, immunotherapy, and surveillance. The selection of treatment modality is depended on several factors such as the stage and histologic subtype of the cancer, as well as the patient’s overall health and preferences. The primary aim of BC treatment is eliminating the cancer and preventing recurrence while maintaining bladder function.
Nanomedicine is a rapidly evolving field with the potential to be a game-changer in the treatment of BC [17]. Using nanoscale carriers, such as NPs to deliver therapeutic agents directly to cancer cells, offers several advantages over conventional chemotherapy [18–20]. Nanomedicine represents a promising avenue for improving the treatment of BC by reducing side effects, enhancing tumor suppression, overcoming drug resistance, and allowing non-invasive imaging of therapeutic agent delivery and distribution [21]. Using NPs as carriers for therapeutic agents enables targeted and controlled release, leading to increased efficacy and decreased toxicity. Additionally, NPs allow for improving drug properties, delivery, and bioavailability. Surface modifications with targeted peptides or antibodies can further enhance recognition and specificity towards tumor cells, making NPs an exciting tool in the field of cancer therapy [22–24]. In addition, NPs can increase therapeutic residence time in the bladder, resulting in improved antitumor efficacy [25]. As we go deep into the study of mechanisms and nanotechnology, there is great potential that through the integration of NPs with other novel technologies, more efficient and reliable systems for the diagnosis and treatment of BC will come out.
Theranostics refers to the integration of diagnostics and therapy into one system. It allows personalized medicine, since the diagnosis and treatment can be tailored for individual based on their diagnosis results [26]. Nano theranostics is a field that combines nanotechnology and therapeutics with diagnostic imaging to enable targeted cancer treatment and monitoring of therapeutic response. In BC, different types of NPs have been developed possessing both therapeutic and diagnostic capabilities [27, 28], which include gold NPs (GNPs), magnetic NPs, quantum dots (QDs), polymeric NPs, and lipid-based NPs. Theranostics applications in BC include targeted delivery of chemotherapy, imaging of cancer cells, and hyperthermia therapy. By using theranostics, treatment can be customized to each patient’s specific needs, improving patient outcomes and reducing side effects from treatment.
Nanomaterials for BC theranostics
Metal NPs
GNPs
GNPs are known for their remarkable features such as high surface area-to-volume ratio, surface plasmon resonance (SPR), surface chemistry, multifunctionality, ease of synthesis, and stability [29, 30]. These features make them potential tools for diagnosing various cancers and drug delivery applications [31]. Moreover, their non-toxic and non-immunogenic nature, as well as their high permeability and retention effect, offer additional benefits by facilitating drug penetration and accumulation at tumor sites. The unique optical and SPR characteristics of GNPs have enabled their application in highly sensitive detection and image-based therapeutic technologies, particularly for the treatment of fatal diseases such as cancer [29, 32].
The properties of GNPs are influenced by various factors, including particle size, shape, and other variables related to their chemical structure. Different types of GNPs were designed for the diagnosis and imaging of BC. Feng et al. [33] developed a novel method based on surface-enhanced Raman scattering (SERS) and colorimetric method for controllable assembly and aggregation statement of telomerase activity assay (Figure 1). Li et al. [34] designed a label-free electrochemical immunosensor as a signal amplification matrix, to efficient detect nuclear matrix protein 22 (NMP22) with high sensitivity. Nossier et al. [35] prepared cationic GNPs to detect urinary HAase activity sensitively and rapidly. The development of a colorimetric Au NPs assay provides direct detection hyaluronidase in urine.
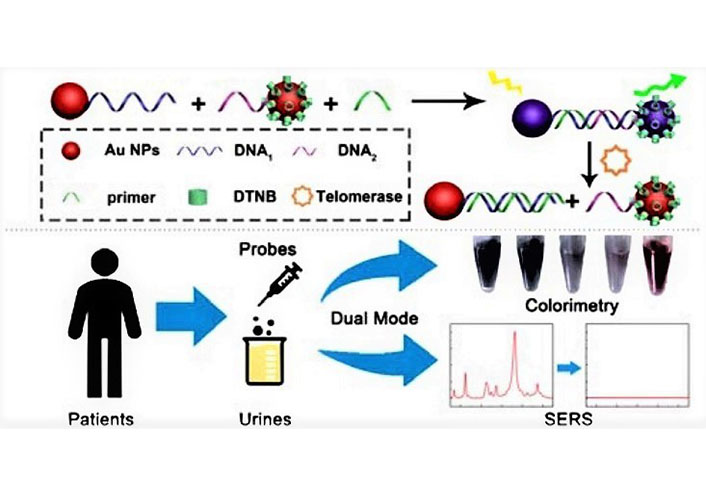
Schematic illustration of a novel approach for analyzing telomerase activity in cell extracts using an Au dimer-based system. This dual-mode Au nanoprobe integrates both SERS and colorimetry, enabling sensitive detection of telomerase activity not only in cell extracts but also in urine samples from patients. DTNB: 5,5’-dithio-bis-nitrobenzoic acid [33]
Note. Reprinted from “Dual-mode Au nanoprobe based on surface enhancement Raman scattering and colorimetry for sensitive determination of telomerase activity both in cell extracts and in the urine of patients,” by Feng E, Zheng T, Tian Y. ACS Sens. 2019;4:211–7 (https://pubs.acs.org/doi/10.1021/acssensors.8b01244). © 2019 American Chemical Society.
Gessner et al. [36] developed magnetic GNPs with an idealized coating. GNPs exhibited a significantly lower rate of serum protein adsorption, enabling them to detect specific BC biomarkers at low concentrations and in the presence of other proteins. Hati et al. [37] proposed a novel solid-state design approach using photoinduced spiropyran-cyanine self-assembled monolayers covalently linked to gold triangular nanoprisms via alkylthioesters. The large localized SPR response was generated due to the reversible conformational change of nanoprisms. Zhang et al. [38] developed a high-performance fluorescent sensor using plasmonic gold nanorods (Au NRs) to enhance the near-infrared (NIR) fluorescence (NIRF) of silver sulfide QDs. The fluorescent sensor is a highly sensitive, dependable, and straightforward technique that holds great potential for clinical applications, including cancer screening, tumor grade classification, and postoperative monitoring. Zhang et al. [39] reported a one-pot synthesis of orange-yellow luminescent core-shell nanoclusters. The synthesized gold nanoclusters (Au NCs) have been used as photoluminescent probes for imaging human BC cells successfully. Liang et al. [40] developed a multiple ratiometric gold nanoprobe based on SERS for precise molecular detection and imaging of BC.
Photothermal therapy (PTT) can aid in overcoming the bladder mucosal barrier and improve drug delivery. Gold nanomaterials with strong photothermal performance and good drug loading capacity are potential carriers for drug delivery. Sun et al. [41] proposed a nanocarrier for drug delivery that is expected to optimize bladder mucosal permeability and controlled drug burst release. They developed a 1,064 nm laser-triggered gas-driven doxorubicin (DOX) burst-release nanosystem, which is suitable for combined chemo-photodynamic therapy (CDT) of BC in situ (Figure 2). Wang et al. [42] developed a hyaluronic acid (HA)-modified NP consisting of Au@SiO2@Au for PTT of genitourinary system tumors, which specifically targets the HA of cancer cells overexpressing CD44 receptors to improve the accumulation of NPs in tumors. The treatment with NPs resulted in the disappearance of bladder and prostate tumors 15 and 18 days after treatment, respectively, with no recurrence observed. Hsu et al. [43] developed a folic acid (FA)-conjugated Au@TNA NP loaded with methylene blue to enhance photodynamic therapy (PDT) in BC cells. Experimental results showed that this safe photomedicine has great potential in achieving localized depletion of cancer sites without side effects.
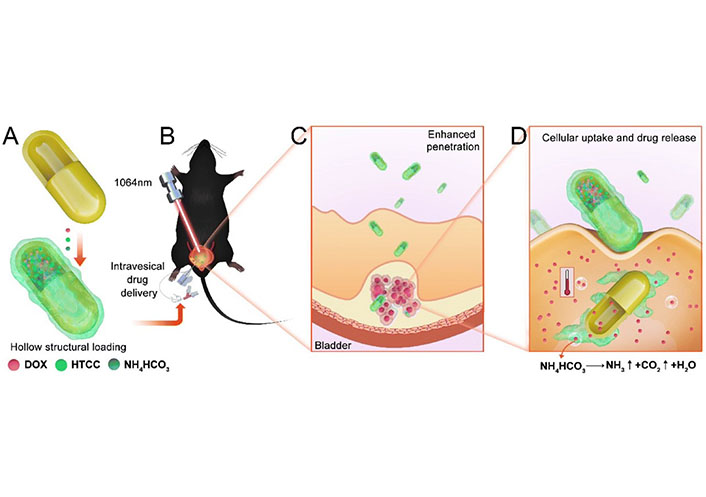
The synthesis illustration of nanosystem and in vivo treatment. (A) The hollow structure loading of DOX, N-(2-hydroxyl)-propyl-3-trimethyl ammonium chitosan chloride (HTCC), and NH4HCO3; (B) the intravesical drug delivery and CDT in orthotopic BC model; (C) enhanced bladder mucosal barrier penetration; (D) enhanced endocytosis and drug burst release driven by gas [41]
Note. Reprinted from “NIR-II-triggered doxorubicin release for orthotopic bladder cancer chemo-photothermal therapy,” by Sun Z, Zhang W, Ye Z, Yuan L, Fu M, Liu X, et al. Nanoscale. 2022;14:17929–39 (https://pubs.rsc.org/en/content/articlelanding/2022/NR/D2NR04200B). © 2022 The Royal Society of Chemistry.
In a recent study, Liao et al. [44] synthesized Au@Chl/Fe nanorods with high stability using natural photosensitizer iron chlorophyllin (Chl/Fe) (Figure 3). They demonstrated that preliminary PDT could restore Fenton-like response-mediated chemokinetic ferroptosis in T24 cells. Orthotopic tumor-bearing mice were locally administered with Au@Chl/Fe combined with PDT in restricted areas, resulting in the successful cure of malignant BC without recurrence. Systemic toxicity was minimized by the local therapy, intravesical instillation of the nanorods, which prevented the material from entering the systemic circulation. After activation of NIR PDT-triggered chemokinetic therapy, ultrasound imaging revealed almost complete tumor remission. The study showed that green photosensitizers (PSs) can achieve antitumor efficacy and survival benefits. Ghoshal et al. [45] developed composite NPs by embedding chitosan and alginate in Au NCs, which effectively bind bacterially expressed human recombinant secreted frizzled-related protein 1 (sFRP1). The sustained release of sFRP1 outside the cell is enabled by these NPs. Additionally, the remarkable luminescent properties of Au NCs are utilized for binding, imaging, and tracking studies. The therapy entails the synergistic use of sFRP1-loaded NPs and the drug cisplatin, which targets two distinct pathways in order to induce apoptosis through a combination of their individual effects.
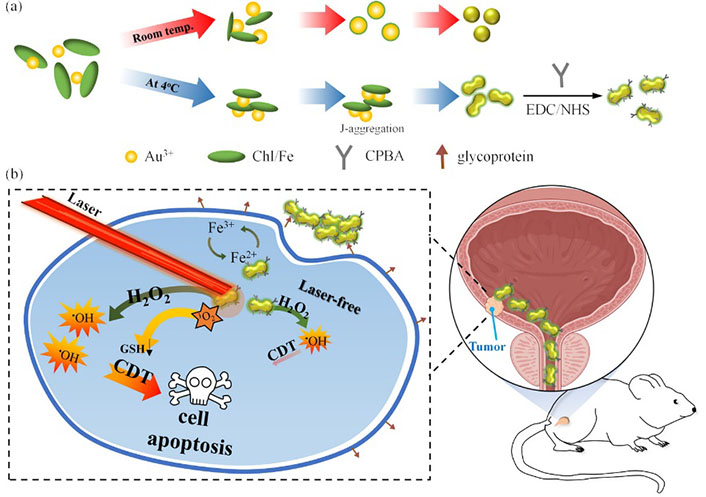
Synthetic illustration of the synthesis of Au@Chl/Fe nanorods and their ability to enhance reactive oxygen species (ROS) generation through NIR-mediated PDT, leading to depletion of glutathione and enabling combined PDT and CDT for effective BC therapy. CPBA: carboxyphenylboronic acid); EDC: 1-Ethyl-3-(3’-dimethylaminopropyl)carbodiimide; GSH: glutathione; NHS: N-hydroxysuccinimide [44]
Note. Adapted from “Surfactant-free green synthesis of Au@Chlorophyll nanorods for NIR PDT-elicited CDT in bladder cancer therapy,” by Liao MY, Huang TC, Chin YC, Cheng TY, Lin GM. ACS Appl Bio Mater. 2022;5:2819–33 (https://pubs.acs.org/doi/10.1021/acsabm.2c00228). © 2022 American Chemical Society.
Iron oxide NPs
Iron oxide NPs (IONPs) exhibit superparamagnetic properties, which enable them to reduce the relaxation time of nearby protons, rendering them valuable as contrast agents for MRI [46–48]. The advent of multimodal imaging techniques has generated tremendous interest as a powerful approach for cutting-edge biomedical research, as well as for the diagnosis and treatment of cancer. Key et al. [49] developed a multimodal NP system for enhanced BC diagnosis and surgery using both MRI and NIRF imaging. The system employed 350 nm ethylene glycol chitosan as a carrier for MRI contrast agents and NIRF probes. Further, to improve cancer cell internalization, BC-targeting peptides and cyanine 5.5 were conjugated to ethylene glycol chitosan NPs. This study has shown the potential of the system for accurate tumor diagnosis and real-time image-guided tumor surgery by utilizing MRI and NIRF imaging. Chou et al. [50] synthesized water-soluble FePt NPs with varying diameters of 3 nm, 6 nm, and 12 nm and have demonstrated their potential as dual-modality contrast agents for molecular imaging using both CT and MRI techniques (Figure 4).
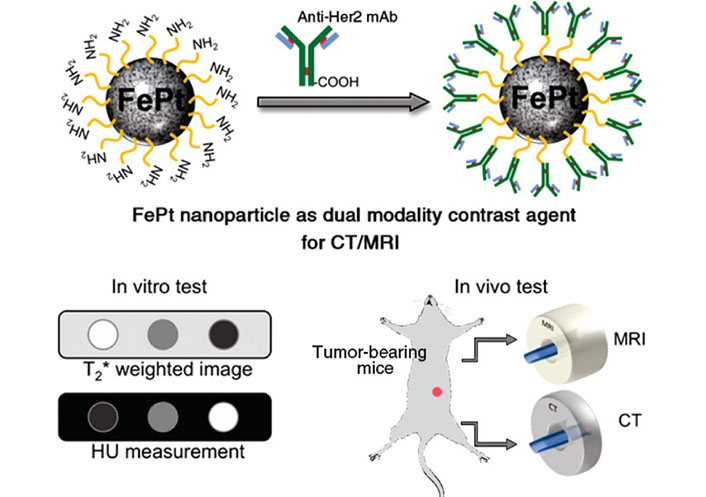
Schematic illustration showed that a cysteamine-capped FePt NP was conjugated with anti-Her2 antibody to serve as a dual contrast agent for in vitro and in vivo MRI and CT evaluation, as depicted in the schematic illustration. HU: Hounsfield unit [50]
Note. Adapted from “In vitro and in vivo studies of FePt NPs for dual modal CT/MRI molecular imaging,” by Chou SW, Shau YH, Wu PC, Yang YS, Shieh DB, Chen CC. J Am Chem Soc. 2010;132:13270–8 (https://pubs.acs.org/doi/10.1021/ja1035013). © 2010 American Chemical Society.
IONPs that are loaded with chemotherapeutic drugs have been successfully employed in magnetic drug targeting approaches to increase drug accumulation and efficacy in BC treatment. Various magnetic drug carriers have been designed to deliver different types of chemotherapeutic agents. Zhang et al. [51] designed a magnetic thermosensitive hydrogel that serves as an innovative delivery system for intravesical BCG, providing a prolonged residence time in the bladder through magnetic field guidance. In a separate study, Suo et al. [52] developed a safe magnetic adhesion system, which effectively extended the duration of epirubicin (EPI) instillation in the bladder under external magnetic influence. While Huang et al. [53] designed polymer NPs that encapsulate superparamagnetic iron oxide and are conjugated with cisplatin, demonstrating their potential as a therapy for BC.
Zakaria et al. [54] prepared a novel type of nanoporous IONPs with controllable release and magnetic permeability properties using a thermal conversion method. These NPs have shown potential as drug delivery systems (DDS) for targeting BC cells. Wang et al. [55] designed a novel trifunctional Fe3O4/CaP/alginate core-shell corona NP with magnetic guidance, pH-responsiveness, and targeted chemotherapy for BC. The study demonstrated that successful magnetic guidance, pH-responsive release, and improved cellular uptake of the NPs led to enhanced chemotherapeutic effects on BC cells. Sun et al. [56] designed a magnetic thermosensitive hydrogel, which transformed into a gel and adhered to the bladder wall upon perfusion into the bladder. Due to its sustained release and targeting properties, the hydrogel demonstrated better anti-tumor effects in BC.
In addition to magnetic drug targeting, IONPs have also been used to induce ferroptosis. Qi et al. [57] designed a mucoadhesive hydrogel for intravesical delivery, aiming to induce ferroptosis in chemotherapy-resistant BC through a three-layer strategy using IONPs (Figure 5). Compared to systemic administration, this three-layer strategy allowed for stepwise delivery of IONPs from the anatomical to the cellular level, resulting in an up to 50-fold increase in iron content. Jasim et al. [58] developed ultrastable, biofunctionalized, and encapsulated IONPs with conjugated polymers for ferroptosis-assisted chemokinetic therapy. Tumor cell death is achieved through ferroptosis, a ROS mechanism that is independent of external activation. Chin et al. [59] developed iron oxide@chlorophyll cluster NPs for the elimination of BC through PDT-induced ferroptosis and immune stimulation. The study successfully demonstrated that the NPs have a combined CDT-enhanced PDT function by regulating redox balance and reprogramming the tumor microenvironment to effectively treat BC.
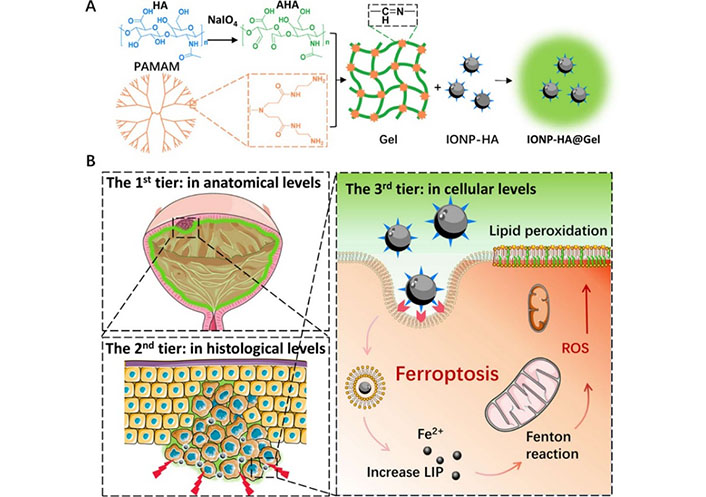
Schematic illustration of the three-tier delivery strategy using intravesical administration of IONP-HA@Gel. (A) IONP-HA@Gel preparation; (B) the three-tier delivery strategy. The first-tier delivery to achieve high nanomedicine concentration on the tumor surface, second-tier delivery to reduce tumor stiffness, and third-tier delivery through CD44-mediated phagocytosis to induce ferroptosis of chemoresistant BC [57]
Note. Adapted from “Intravesical mucoadhesive hydrogel induces chemoresistant bladder cancer ferroptosis through delivering iron oxide nanoparticles in a three-tier strategy,” by Qi A, Wang C, Ni S, Meng Y, Wang T, Yue Z, et al. ACS Appl Mater Interfaces. 2021;13:52374–84 (https://pubs.acs.org/doi/10.1021/acsami.1c14944). © 2021 American Chemical Society.
IONPs have unique properties that make them particularly well-suited for theranostic applications [46, 47]. One example of such an approach, in which diagnostics and therapeutics are closely integrated, is image-guided drug delivery for BC using IONPs. Tao et al. [60] successfully fabricated superparticles composed of polydopamine (PDA)-coated Fe3O4 that were loaded with vincristine and modified with folate for use in the theranostics of BC. The superparticles possess MRI and chemotherapy functions due to the presence of superparamagnetic Fe3O4 and vincristine. In combination with NIR laser, the synergistic effect of MRI, PTT, and chemotherapy resulted in successful multifunctional diagnosis and treatment of BC. Guo et al. [61] developed a gel system combining photothermal, ironphoto, and immunotherapy targeted by intravesical instillation. In this system, PTT is performed by Au NRs under imaging-guided NIR radiation, while high local concentrations of IONPs are taken up by cancer cells to induce ferroptosis. Lin et al. [62] developed a nanoscale oxygen generator (PLZ4@SeD) that was encapsulated with superparamagnetic IONs (SPIONs) and organic selenium, and targeted BC using PLZ4 peptide (cQDGRMGFc) as the targeting moiety (Figure 6). This approach allowed for intravesical instillation to overcome hypoxia-induced resistance and enabled real-time MRI of patient-derived bladders to guide treatment of degenerated tumors.
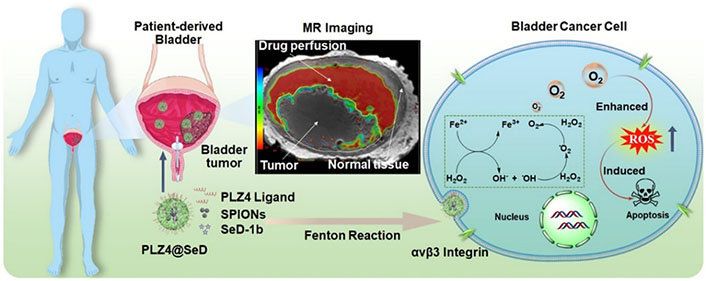
Schematic illustration of the nanoscale oxygen generator PLZ4@SeD, which enables real-time MRI-guided intravesical instillation theranosis of patient-derived BC The nanogenerator targets bladder tumors and relieves hypoxia by consuming excess endogenous H2O2, inducing apoptosis [62]
Note. Reprinted from “Pre-clinical MRI-guided intravesical instillation theranosis of bladder cancer by tumor-selective oxygen nanogenerator,” by Lin W, Liu H, Chen L, Chen J, Zhang D, Cheng Q, et al. Nano Today. 2021;38:101124 (https://www.sciencedirect.com/science/article/pii/S1748013221000499?via%3Dihub). © 2021 Elsevier Ltd.
Metal-organic frameworks
Metal-organic frameworks (MOFs) are a class of porous crystalline materials featuring periodic frameworks made of organic ligands and metal oxide clusters or metals. With their tunable pore size, large surface area, and pore volume, as well as the ease of surface modification, MOFs represent a novel and versatile porous material that has gained considerable attention in the biomedical field, particularly for drug delivery applications [63–66].
Wang et al. [67] investigated and developed a novel hydrophilic Mg-MOFs-coated functionalized magnetic graphene composite with controllable thickness for selective glycopeptide enrichment from human urine. Remarkably, the composite facilitated the identification of 185 N-glycosylated peptides corresponding to 406 glycoproteins, including potential BC biomarkers, in the urine of BC patients. This hydrophilic porous MOFs functionalized composite holds great promise for high-throughput analysis of low-abundance biomolecules in urine, thereby offering a new avenue for rapid and convenient BC diagnosis. Qi et al. [68] developed a zinc oxalate MOFs-based electrochemiluminescence nanoprobe by capturing a luminophore for Apo-A1 detection. The novel probe was effectively employed to quantify Apo-A1 levels in actual serum and urine samples, demonstrating a remarkable recovery rate ranging from 98.0% to 115.0% with a relative standard deviation of less than 5.2%. This can be beneficial for clinical diagnosis of BC.
Different structures of DNA are widely used in the field of biomedicine [69–72]. Li et al. [73] developed a portable photoelectrochemical (PEC) sensing system for detecting telomerase activity, utilizing dual biomineralized zeolitic imidazolate framework-8 (ZIF-8) NPs for chemical energy conversion to electrical energy and the elongation of Y-bonded DNA structures catalyzed by terminal deoxynucleoside transferase (TdT) (Figure 7). The study demonstrated the efficacy of utilizing MOF NPs for the development of a highly effective diagnostic tool to assess telomerase activity in resected bladder tumor tissues. This innovative approach not only provides a promising diagnostic platform for detecting telomerase-related diseases, but also establishes a novel method for creating PEC assays using MOF NPs.
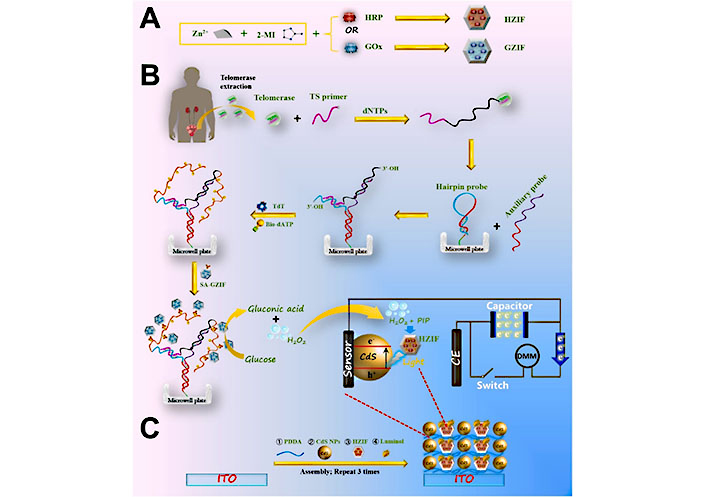
Schematic illustration of the synthesis of glucose oxidase (GOx)-encapsulated ZIF-8 (GZIF) and horseradish peroxidase (HRP)-encapsulated ZIF-8 (HZIF), followed by PEC detection of telomerase activity based on TdT-catalyzed elongation of Y-junction DNA structure and fabrication of luminol/HZIF/CdS-based photoelectrode. 2-MI: 2-methyl imidazole; Bio-dATP: bio-deoxyadenosine triphosphate; CE: carbon electrode; DMM: digital multimeter; dNTPs: deoxy-ribonucleoside triphosphate; ITO: indium tin oxide; PDDA: poly(diallyldimethylammonium chloride); PIP: p-iodo-phenol; SA-GZIF: streptavidin-modified GZIF; TS: telomerase substrate [73]
Note. Reprinted from “Dual biomineralized metal––organic frameworks-mediated conversion of chemical energy to electricity enabling portable PEC sensing of telomerase activity in bladder cancer tissues,” by Li F, Xu M, Zhuang J. Biosens Bioelectron. 2022;204:114070 (https://www.sciencedirect.com/science/article/pii/S0956566322001105?via%3Dihub). © 2022 Elsevier B.V.
MOFs have been utilized in novel DDS due to their ability to tune pore size, possess large surface area and pore volume, and allow for easy surface modification [63]. Li et al. [74] developed a novel bacterial microswimmer system with a cytoprotective MOFs exoskeleton, demonstrating both self-protection and active drug delivery capabilities. By exploiting the chemotaxis of microswimmers and their chemoattractant navigation propulsion, targeted drug delivery was achieved, leading to enhanced anticancer efficacy. The intrinsic motility of these bacteria-driven microswimmers also promoted their binding to the mouse bladder wall and prolonged residence time in the bladder region, thus presenting new opportunities for the advancement of therapeutic platforms for bladder diseases. Chen et al. [75] developed a nanocarrier, named HAFeR, which encapsulates PSs and RSL3 [inhibitor of glutathione peroxidase 4 (GPX4)] within MOFs, to achieve synergistic ferroptosis and PDT. This combination of apoptosis and ferroptosis was shown to exhibit strong toxicity to tumor cells, resulting in the effective eradication of tumors in tumor-bearing nude mice under laser light (Figure 8).
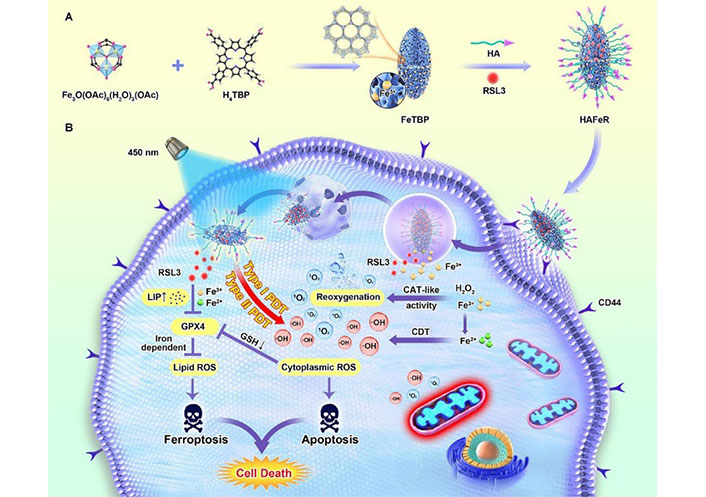
Schematic illustration of the synthesis process of hypoxia-tolerant HAFeR MOFs and their proposed mechanism of action in tumor cells. With the aid of the HA decoration, HAFeR effectively targets tumor cells via HA-CD44-mediated endocytosis. Upon lysosomal decomposition, HAFeR releases O2 and Fe2+ to enhance the cytotoxicity of PDT, ultimately leading to synergistic ferroptosis and PDT for hypoxic tumors. H4TBP: 5,10,15,20-tetra(p-benzoato)porphyrin; LIP: labile iron pool [75]
Note. Reprinted from “Photoactivatable metal organic framework for synergistic ferroptosis and photodynamic therapy using 450 nm laser,” by Chen Y, Gu L, Ma B, Li X, Mei Y, Zhou J, et al. Chem Eng J. 2023;454:140438 (https://www.sciencedirect.com/science/article/pii/S1385894722059186?via%3Dihub). © 2022 Elsevier B.V.
MOFs were demonstrated as a theranostic nanoplatform that combines drug therapy and phototherapy. Shang et al. [76] developed a multifunctional platform consisting of MOFs-drug NPs that enable simultaneous NIR hyperthermia, drug delivery, and MRI imaging under various drug administration modes. Microporous Fe-MOFs are used as MRI reagents for localizing tumors and nanocarriers, thereby improving the upload of chemotherapeutic drugs. Additionally, MOFs have shown remarkable targeting capabilities when administered through different routes, such as intravenous injection and local instillation for BC. It is worth mentioning that MOFs have exhibited high efficiency in PTT and drug release around the tumor site upon exposure to 808 nm laser light. This synergistic approach provides a promising strategy for drug delivery and can be customized for various cancer cell types and molecular targets implicated in disease advancement.
Inorganic NPs
Silica NPs
Silica NPs (SNPs) serve as promising carriers for a variety of imaging and chemotherapy agents, including organic dyes, QDs, DNA, and PSs [77–79]. Furthermore, the use of established surface modification techniques can provide SNPs with solubility, surface charge control, and targeted delivery capabilities [80].
Different surface modification strategies have been applied to SNPs to enhance their efficacy in the treatment of BC [81]. Zhang et al. [82] developed β-cyclodextrin-modified mesoporous SNPs (MSNPs) with hydroxyl, amino, and thiol groups, and compared their mucoadhesive properties and drug delivery potential for BC therapy. Thiol-functionalized NPs showed higher mucus adhesion in the urinary tract, attributed to disulfide bond formation with mucus glycoprotein, and were loaded with DOX for pH-triggered drug release. In another study, Ellina A. et al. [83] found that thiolated SNPs exhibited higher retention on porcine bladder mucosa than non-mucoadhesive dextran, but less than chitosan. PEGylation reduced their mucoadhesion. Wei et al. [84] prepared targeted DOX-loaded MSNPs with PDA coating and CSNRDARRC peptide for enhanced therapeutic effect on BC. The release of DOX is pH-sensitive and controlled by the PDA coating. NPs showed superior efficacy to free DOX and DOX-loaded MSNPs@PDA, with specific recognition of HT-1376 cells as a potential targeted DDS for BC.
Wang, et al. [85] investigated the mucoadhesive ability and controlled drug release properties of polyamidoamine (PAMAM)-modified MSNPs by layer-by-layer grafting. PAMAM-modified MSNPs showed improved mucoadhesion with increased PAMAM amino groups, reaching maximum adhesion after grafting two generations of PAMAM onto MSNPs. The findings suggest that PAMAM-modified MSNPs have potential as a DDS for BC therapy. In addition to enhanced adhesion capabilities, Hortelao et al. [86] developed a unique MSNPs-based nanomotor, featuring an outer surface consisting of polyethylene glycol and anti-fibroblast growth factor receptor 3 (FGFR3) antibody, targeting 3D spheroids BC cells. This nanomotor can be powered by urea, a fuel that is naturally abundant in the bladder, and propels the NPs to move actively (Figure 9).
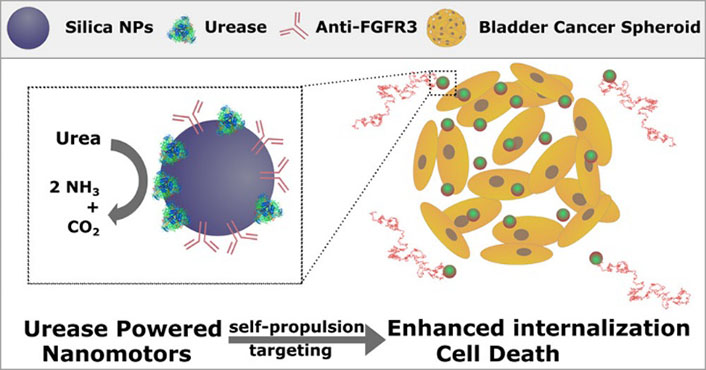
Schematic illustration of MSNPs with polyethylene glycol and anti-FGFR3 antibody on their outer surface were utilized as urease-powered nanomotors to target BC cells in 3D spheroids. The autonomous motion was driven by urea, which is abundant in the bladder [86]
Note. Reprinted from “Targeting 3D bladder cancer spheroids with urease-powered nanomotors,” by Hortelão AC, Carrascosa R, Murillo-Cremaes N, Patiño T, Sánchez S. ACS Nano. 2019;13:429–39 (https://pubs.acs.org/doi/10.1021/acsnano.8b06610). © 2018 American Chemical Society.
MSNPs possess unique properties that make them suitable for delivering nucleic acids, such as a high surface area and pore volume, tunable particle and pore sizes, and variable morphology. Moreover, their ability to create core-shell particles with distinct surface functionalization further enhances their potential as effective anti-cancer nanocarriers. Haddick et al. [87] synthesized a series of core-shell MSNPs with different particle sizes between 60 nm and 160 nm to study the size effect on anti-tumor microRNA (miRNA) delivery. The NPs were comparable in all other properties, including surface area, pore size, and zeta potential. The copolymer was designed to bind to the MSNP shell through charge matching and simultaneously act as an endosome-releasing agent. The peptide ligand GE11 targeting epidermal growth factor receptor was also functionalized. The nanoscale MSN160 nm-454-GE11 vector shows promising potential for future in vivo biomedical applications due to its rapid cellular internalization.
In a recent study, Shahidi et al. [88] presented a promising approach for BC therapy through the use of MSNPs loaded with multicomponent siRNA/miRNA. This nanocarrier was constructed using cyclic (Arginine-Glycine-Aspartic acid-d-Phenylalanine-Lysine) [c(RGDfK)]-poly(lactide-coglycolide) (PLGA)-poly(ethylene glycol) (PEG) modification for co-delivery of miR-34a and programmed cell death-ligand 1 (PD-L1) siRNA in BC cells and mouse models. The study showed that the nanocarrier was biocompatible in the serum environment and protected the therapeutic molecules from serum degradation. Notably, the c(RGDfK)-MSNPs were able to simultaneously downregulate PD-L24 expression and upregulate miR-24a, resulting in enhanced antitumor effects both in vivo and in vitro. These findings highlight the potential of targeted therapeutic strategies with molecular targets to improve BC therapy.
Borzecka et al. [89] proposed a novel silica nanoderivative-based system that holds great promise as an effective vehicle for delivering PSs into tumor tissues during PDT. In their study, the team reported on the preparation, characterization, and in vitro investigation of MSNPs of various shapes, all of which were grafted with S-glycoside porphyrins. Their results highlighted the potential of this PS targeting BC cells for PDT. Interestingly, the MSNPs produced more singlet oxygen and demonstrated higher phototoxicity in cancer cell lines. These findings suggest that this novel spherical nanosystem could be a successful candidate for PDT of BC. Sweeney et al. [90] reported a novel approach using multimodal NP contrast agent technology for preclinical imaging of BC. The innovation involves the engineering of mesoporous silica with specific contrast-enhancing properties for MRI. Real-time and repeated MRI evaluations of the tumors are enhanced by the NP contrast, which has the potential to be translated into human clinical studies for tumor staging, therapeutic monitoring, and drug delivery. This technique offers a promising avenue for non-invasive and accurate imaging of BC, with implications for improving clinical outcomes.
QDs
QDs are a distinctive type of inorganic NP possessing optical properties that render them highly valuable for biomedical applications, especially as fluorescent labels [91]. By emitting light in response to specific wavelengths of light, these nanocrystals have become instrumental in imaging and PDT for BC. The benefits of QDs, such as their high brightness and stability, make them an attractive choice for use in a variety of research fields, including cell biology and medical imaging. Their unique optical properties, coupled with their small size, make them a valuable tool for studying biological processes with high sensitivity and resolution.
In a study by Fan et al. [92], a new class of magnetofluorescent nanoprobes was developed by grafting cationic polyethyleneimine (PEI)-terminated QDs onto negatively charged magnetite nanorods surface modified with citric acid. The resulting nanoprobes exhibited excellent dual-modal properties, combining high luminescence with magnetic vortex cores, which make them promising for intracellular imaging and therapeutic applications in BC. The potential of graphene QDs (GQDs) doped with heteroatoms in biology and as light-emitting materials has led to significant interest. Deng et al. [93] developed nitrogen-doped GQDs (N-GQDs) using a solvent-free, gram-scale mechanochemical method, resulting in broad excitation-dependent full-color photoluminescence (PL) at 672 nm. The study investigated the inhibitory effect of N-GQDs on BC cells, and demonstrated a significant inhibition of cancer cell proliferation with increasing concentrations of N-GQDs.
The use of QD fluorescent probes shows promise in targeted imaging and diagnosis of BC. Masha et al. [94] synthesized carbon-based QDs (CBQDs) that emit blue and green light, exhibiting excellent PL emission across a wide range of excitation wavelengths. The biocompatibility of CBQDs was demonstrated in concentration-dependent cell viability studies on BC cells. Biagiotti et al. [95] developed a fluorescent glycoside probe using QDs coated with a binary mixture of ligands that conferred specific targeting properties based on the terminal domain structure. The probe was designed with monosaccharide residues for dispersion in biological media and QDs for optical/fluorescent properties. This innovative QD-based probe has potential for accurate imaging and diagnosis of BC.
Yuan et al. [96] developed a QD fluorescent probe coupled to a prostate stem cell antigen (PSCA) monoclonal antibody (QD-PSCA) for specific targeted labeling and imaging of bladder urothelial cancer cells in vitro and in vivo. The QD-PSCA fluorescent probe exhibited excellent optical stability, providing a feasible approach for non-invasive targeted imaging, early diagnosis, and targeted tumor treatment in vivo. Despite the superior optical properties of fluorescent NP such as QDs compared to organic dyes, their in vivo applications may be limited by potential toxicity after systemic administration. Therefore, Pan et al. [97] investigated the biodistribution and toxicity of intravesically instilled free QDs and anti-CD47-QDs in mice, revealing that QDs did not accumulate significantly outside the bladder on average but were detected in some extravesical biodistributions. These findings highlight the importance of developing targeted NP with established biosafety profiles to minimize long-term toxicity in clinical settings and reduce systemic exposure.
QDs have emerged as valuable tools for biological analysis and imaging, particularly when combined with the fluorescent resonance energy transfer (FRET) properties of semiconductor QDs. This combination enables enhanced sensitivity and accuracy in biological analyses. Othman et al. [98] reported the development of a highly sensitive fluorescent immunosensor for detecting NMP22, an early diagnostic biomarker of BC. Xu et al. [99] developed a core-shell-shell bifunctional magnetic fluorescent bead (MFB) microscopic imaging method for the multiplex detection of BC miRNA, which integrates catalytic hairpin assembly (CHA) and terminal deoxynucleotidyl TdT-mediated DNA polymerization (Figure 10). MFBs, assembled from QDs and magnetic NPs, are used as dual-functional carriers for optical encoding and magnetic separation. The MFB-CHA-TdT strategy can detect BC-related miRNAs in clinical serum samples and has good consistency with quantitative real-time polymerase chain reaction.
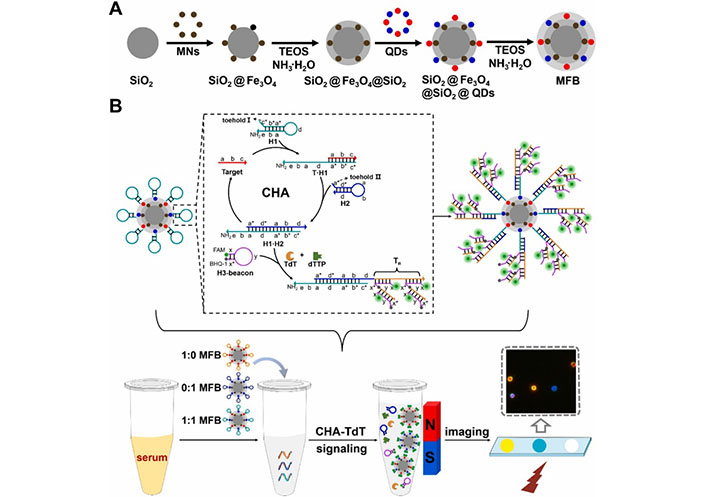
Schematic illustration of constructing MFBs and their application in multiplexed detection of clinical serum specimens. MNs: magnetic iron oxide nanoparticles; TEOS: tetraethyl orthosilicate [99]
Note. Adapted from “Multiplexed detection of bladder cancer microRNAs based on core-shell-shell magnetic quantum dot microbeads and cascade signal amplification,” by Xu J, Wei X, Zhang X, Cai Z, Wei Y, Liu W, et al. Sens Actuators B Chem. 2021;349:130824 (https://www.sciencedirect.com/science/article/pii/S0925400521013927?via%3Dihub). © 2021 Elsevier B.V.
Zhang et al. [100] demonstrated an electrochemical method based on CdTe QDs, QDs modified glassy CEs (GCEs) to detect specific DNA sequences in BC cells. The electrochemical DNA sensor modified with CdTe has the advantages of being easy to operate, low cost, fast in response, selective, and highly sensitive, providing a potential platform for the preparation of other electrochemical biosensors and new strategies for detecting BC DNA. Shan et al. [101] utilized QD immunofluorescence technology to detect the expression of cyclin D1 and cyclin E in urothelial BC tissue chips. The results showed that anomalous expression of cyclin D1 and cyclin E may be associated with the occurrence and development of urothelial BC.
Manan et al. [102] reported the development of a nanocarrier system composed of Mn:ZnS QDs conjugated with chitosan and encapsulated with mitomycin C for the efficient delivery of chemotherapeutic agents (Figure 11). The developed system demonstrated enhanced drug loading, targeted delivery, and prolonged retention at the cancer site, making it a promising approach for the treatment of non-muscle invasive BC to combat cancer recurrence and progression. Isaac et al. [103] utilized single-walled carbon nanohorns as a diagnostic vehicle for imaging and delivering cisplatin to tumor cells, along with QDs for real-time imaging. The dual functionality of nanohorns as imaging beacons and chemotherapy delivery agents was demonstrated, with cisplatin-loaded nanohorns effectively inhibiting cancer cell proliferation. This study highlights the development of the first theranostic carbon nanohorn, which combines QDs for fluorescence imaging and cisplatin as a chemotherapeutic payload.
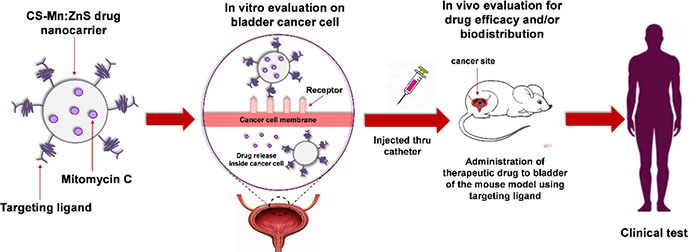
Schematic illustration of the synthesis, characterization, and evaluation of a chitosan-based nanocarrier system that encapsulates mitomycin C, with Mn:ZnS QDs conjugated to its surface [102]
Note. Reprinted from “Drug release profiles of mitomycin C encapsulated quantum dots-chitosan nanocarrier system for the possible treatment of non-muscle invasive bladder cancer,” by Abd Manan FA, Yusof NA, Abdullah J, Mohammad F, Nurdin A, Yazan LS, et al. Pharmaceutics. 2021;13,1379 (https://www.mdpi.com/1999-4923/13/9/1379). CC BY.
Polymer
Polymeric micelles
Polymeric micelles represent a rapidly evolving field in the area of nanotechnology for drug delivery [104–106]. Polymeric micelles are composed of amphiphilic copolymers, which self-assemble into a core-shell structure with hydrophobic and hydrophilic segments. The resulting micelles have a size range of 10 nm to 100 nm and exhibit exceptional stability in biological environments. The hydrophobic core of these micelles can solubilize poorly soluble drugs, while the hydrophilic shell can provide protection from degradation and enhance the pharmacokinetics of the drug. Polymeric micelles are capable of sustained drug release and have been shown to exhibit longer circulation times in vivo than free drugs, leading to improved therapeutic efficacy. In summary, polymeric micelles hold great promise for drug delivery and have shown great potential for targeted cancer therapy, making them an exciting area of research in nanomedicine.
Zhou et al. [107] developed a targeted DDS for intravesical instilled chemotherapy of superficial BC, using c(RGDfK)-modified micelles assembled from poly(ε-caprolactone) (PCL)-b-poly(ethylene oxide) (PCL-b-PEO) diblock copolymers. The micelles exhibited strong affinity to T24 cells and inhibitory effects on cell proliferation when loaded with DOX, indicating their potential as a nano-scaled DDS for BC treatment. In another similar study, Zhou et al. [108] synthesized an amphiphilic diblock copolymer with amine group, PCL-b-PEO-NH2, and conjugated it with FA and fluorescein isothiocyanate to assemble micelles with targeted moieties and fluorescence labeling. Drug-loaded micelles with DOX demonstrated a higher targeting efficiency to T24 cells with FA without any targeting effect on normal human embryonic kidney-293 cells, indicating the potential of these micelles as a drug carrier for intravesical instilled chemotherapy of BC.
Yu et al. [109] synthesized a PEG and poly(N-(2-hydroxypropyl)methacrylamide) block copolymer (PEG-b-PHPMA) with quantitatively introduced FA and DOX. They investigated the effects of FA content and pH on FA receptor-mediated cell endocytosis and pH-responsive DOX release. The study found that FA plays a significant role in targeting and internalization of the conjugates against BC cells, leading to higher cytotoxicity than non-targetable ones and free DOX. In another study, Zhu et al. [110] developed a dual-sensitive NP delivery system for delivering DOX and IR780. The system is self-assembled from PEG-PCL-SS micelles, which can be internal cross-linked under disulfide catalysis conditions. This NPs system has long circulation, low toxicity, and can generate heat under NIR laser irradiation, achieving the combined therapeutic effects of PTT and chemotherapy for the treatment of BC.
As a typical example of nano micelles in the theranostics of BC, Lin et al. [111] designed a multifunctional nanoporphyrin platform (PNPs) coated with a BC-specific ligand named PLZ4 for targeted diagnosis and therapy of BC. The PNPs integrated photodynamic diagnosis, image-guided PDT, PTT, and targeted chemotherapy in a single procedure. DOX loaded PNPs had a slow drug release and long systemic circulation time. The fluorescence signal of PNPs increased efficiently and selectively in BC cells in vitro and in vivo. PDT with PNPs significantly eliminated orthotopic patient derived BC xenograft (PDX) after intravesical treatment. Image-guided PDT and PTT synergized with targeted chemotherapy and significantly prolonged overall survival of mice carrying PDXs. The platform selectively targeted tumor cells for photodynamic diagnosis and served as effective triple-modality therapeutic agents against BCs.
Polymeric NPs
Polymeric NPs have emerged as a promising DDS owing to their unique physicochemical properties [112–114]. These NPs can be prepared from a variety of natural or synthetic polymers such as poly(lactic acid), PLGA, PCL, PEI, PDA, HA, and chitosan. The advantages of polymeric NPs include their ability to control drug release, lower cost, and protection of drugs from environmental degradation [115]. In the treatment of BC, chemotherapy is considered a standard approach after tumor resection, whose high rate of drug excretion poses a challenge to the effectiveness of chemotherapy. Interestingly, the use of mucoadhesive polymers has shown potential in improving the effectiveness and penetrability of chemotherapeutic drugs. This approach can increase the retention time of drug delivery and enhance the overall therapeutic efficacy in the post-surgery treatment of BC patients. However, there are some limitations on polymeric NPs, for example, some polymers such as poly(lactic acid) have poor toughness and slow degradation rates, while chitosan exhibits low water solubility and poor strength. Simultaneously, the toxicity associated with polymeric NPs is a major concern, making the improvement of the biocompatibility of polymeric NPs essential for safe biomedical applications.
Some progresses have been made in chitosan NPs for delivery. Xu et al. [116] developed a reduction-sensitive chitosan-based DDS for targeted treatment of BC. The system delivers a ROS activated prodrug of gambogic acid, selectively releasing the therapeutic agent in response to glutathione and ROS levels within cancer cells. The positively charged chitosan enhances adhesion and permeability, resulting in an efficient delivery system with minimal damage to normal cells. Additionally, the same vehicle was used to deliver the ROS activatable EPI prodrug and potent NQO1 substrate, KP372-1, resulting in specific inhibition of BC cell proliferation [117]. This cascade drug activation lowered toxicity in normal cells and broadened the selectivity window during intravesical BC chemotherapy. This system has great potential as a targeted DDS for BC treatment.
In the treatment of BC, a fluorinated polymer DDS has the potential to improve drug penetration. Li et al. [118] developed self-assembling fluorinated PEI (F-PEI) based NPs by mixing with Chorin-e6-coupled catalase (CAT; CAT-Ce6). By permeating bladder tumors to decompose endogenous H2O2, CAT-Ce6/F-PEI NPs effectively relieved tumor hypoxia and benefited PDT, exhibiting improved transmembrane, transmucosal, and intratumoral penetration capabilities. In another study, Sun et al. [119] developed a photoactivatable H2 nanogenerator composed of fluorinated chitosan, chemotherapeutic drugs, and an H2 production catalyst, which could form self-assembled NPs. These NPs could effectively generate H2 in situ under laser irradiation, improving the efficacy of hydrogen chemotherapy against cancer in vitro and in vivo.
Barbara et al. [120] developed a two-step method to load hydrophobic photosensitizer Ce6 into PDA NPs, which maintains their surface charge and hydrophilicity, potentially allowing for mucosal drug delivery. This approach shows promise for effective cancer phototherapy. In another study, Tan et al. [121] developed a cathepsin B-sensitive polymer-paclitaxel (PTX) prodrug-based nanosized DDS named NPs@Ce6, comprising of a photosensitizer Ce6 and a stimuli-responsive poly [oligoethylene glycol methacrylate (OEGMA)]-PTX [poly(OEGMA)-PTX]. This system achieved photochemical internalization effect and enhanced CDT through a two-stage light irradiation strategy (Figure 12). In PDX models, the application of NPs@Ce6 resulted in tumor growth inhibition exceeding 98%. These results suggest that NPs@Ce6 could serve as a potential candidate for BC therapy.
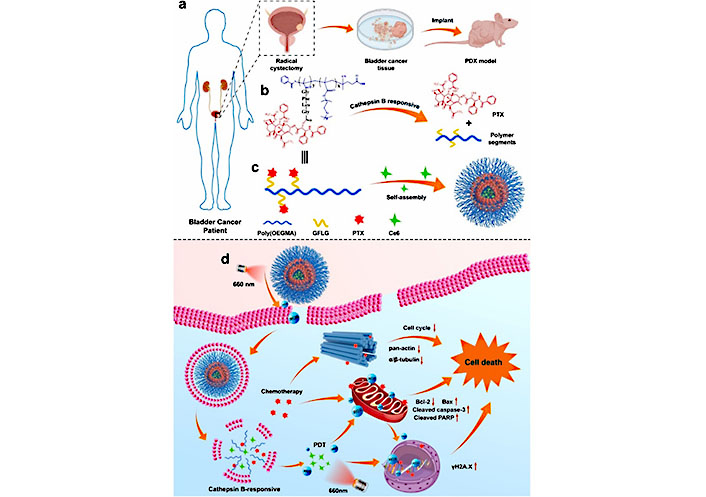
Schematic illustration of a BC PDX model and the use of a poly(OEGMA)-based polymer prodrug to release PTX upon cathepsin B treatment. Self-assembly of the polymer with PTX and Ce6 results in NPs@Ce6 NPs, which produce ROS and exert PCI under short-term light irradiation while also enhancing cellular uptake. Under long-term irradiation, Ce6 produces a large amount of ROS that synergizes with PTX to induce tumor cell death. GFLG: Gly-Phe-Leu-Gly [121]
Note. Adapted from “Enhanced chemo-photodynamic therapy of an enzyme-responsive prodrug in bladder cancer patient-derived xenograft models,” by Tan P, Cai H, Wei Q, Tang X, Zhang Q, Kopytynski M, et al. Biomaterials. 2021;277:121061 (https://www.sciencedirect.com/science/article/pii/S0142961221004178?via%3Dihub). © 2021 Elsevier Ltd.
Liposomes
Liposomes are nanovesicles consisting of synthetic phospholipids and a bilayer membrane shape, which have been widely used as carriers for various biological and biochemical molecules, including drugs, nucleotides, and proteins [122–124]. Liposomes offer several advantages, including the ability to dissolve both hydrophilic and hydrophobic molecules, biocompatibility due to their derivation from cholesterol and phospholipids, good stability, and the potential for effective drug transport when prepared using appropriate procedures.
Liposome-based DDS have shown promise for the treatment of BC, with studies demonstrating targeted drug delivery and enhanced therapeutic effects. Zhai et al. [125] developed a peptide-targeted liposome that carried an amino-terminal fragment with β-elemene to deliver drugs directly to urokinase plasminogen activator receptor overexpressing BC cells, in combination with cisplatin for effective BC treatment. Lv et al. [126] showed that the release of phosphatidylglycerol-based thermosensitive liposomes (DPPG-TSL)-triggered drug may prevent the toxicity of surgical cystectomy and systemic chemotherapy. The combination of liposomes and intravesical hormone therapy demonstrated superiority compared to systemic and intravesical DOX. Liposomes-encapsulated chemotherapy drugs may play a role in the future treatment of muscular invasive BC.
Ghaferi et al. [127] evaluated the therapeutic potential of cisplatin-loaded liposomes and PEGylated liposomes as nanoformulations for the therapy of BC. Compared with cisplatin, PEGylated liposomes is a potential approach to realize nanoformulations with enhanced anticancer effects and reduced toxicity. Zhang et al. [128] prepared signal self-amplifying photoacoustic (PA) liposomal nanoprobes with purpurine-18 aggregated and ammonium bicarbonate payload within a bilayer (Figure 13). Purpurine-18 aggregates generate localized heat efficiently under PA laser irradiation, which resulted in the emission of broadband ultrasonic emissions. The results showed that small tumor lesions (< 5 mm) were identified using this PA probe, with 18-fold enhancement compared to a similar tumor-to-normal tissue ratio, making tumor borders distinct. In conclusion, the study demonstrated a novel strategy to explore high-performance imaging probes, which may be beneficial for imaging-guided surgery in the clinic.
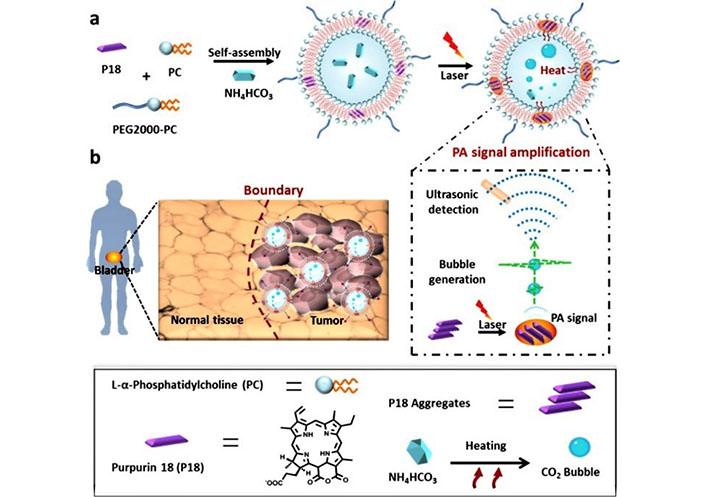
Schematic illustration of the nanobubble generation in nanoprobes under laser irradiation and the high-performance identification of human BC and tumor boundaries using nanoprobes as a PA imaging probe [128]
Note. Adapted from “High-performance identification of human bladder cancer using a signal self-amplifiable photoacoustic nanoprobe,” by Zhang D, Wang Z, Wang L, Wang Z, Wang H, Li G, et al. ACS Appl Mater Interfaces. 2018;10:28331–9 (https://pubs.acs.org/doi/10.1021/acsami.8b08357). © 2018 American Chemical Society.
As a typical application of liposomes in BC theranostics, Hong et al. [129] developed a novel bimodal imaging strategy that combines 3D NIR-II PA and optical coherence tomography angiography (OCTA) for guiding interventional PTT of BC (Figure 14). They used a liposome-coated NIR-II HA-IR-1048 photothermal agent with strong NIR-II optical absorption, high PTT efficacy, and better tumor targeting. This approach allowed dynamic monitoring of PTT response with high sensitivity and specificity, and precise treatment of in situ BC up to 4.38 mm deep. The complex microvascular morphology and angiogenesis of BC were well-monitored and evaluated in vivo using OCTA. Under the guidance of laparoscopy and ultrasound, the minimally invasive intervention PTT strategy was successfully implemented to achieve the controllable and accurate treatment of BC in situ. Overall, this multimodality NIR-II optical imaging combined with laparoscopic interventional tumor protocol represents a major advance in the therapy of primary tumors of the urinary system.
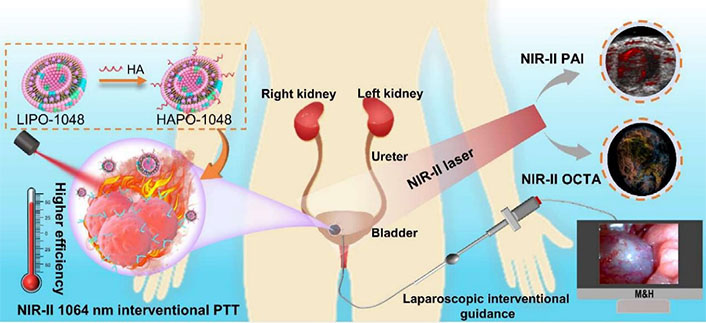
Schematic illustration of a non-invasive interventional PTT for BC enabled by dual-modality NIR-II optical imaging. The imaging modality can guide the therapy deeper into the tissue for effective treatment of orthotopic BC in vivo. HAPO-1048: HA-modified and liposome-coated IR1048 (LIPO-1048); M&H: minimally invasive interventional PTT system; PAI: photoacoustic imaging [129]
Note. Reprinted from “Deep NIR-II optical imaging combined with minimally invasive interventional photothermal therapy for orthotopic bladder cancer,” by Hong F, Geng X, Min G, Sun X, Zhang B, Yao Y, et al. Chem Eng J. 2022;449:137846 (https://www.sciencedirect.com/science/article/pii/S1385894722033332?via%3Dihub). © 2022 Elsevier B.V.
Conclusions
Theranostics of BC is a rapidly evolving and promising field of research. Currently, there is growing interest in the development of NP-based theranostic systems for BC, which offer improved accuracy of diagnosis and targeted drug delivery. These systems are designed to allow simultaneous imaging and treatment of BC, and have shown promising results in both preclinical and clinical studies.
The new and improved nano theranostic systems is one of the most promising future directions for BC treatment, which will likely to involve the integration of multiple imaging modalities and therapeutic approaches, as well as the exploration of novel and more effective NP platforms. Combination therapies that allow multiple drugs to treat BC concurrently represent another important area of research.
The integration of theranostic systems and personalized medicine represents an important future direction for BC treatment. This approach enables more personalized and effective treatment strategies to be developed based on a patient’s specific medical history and genetic profile. Using predictive algorithms and big data analytics is expected to play a key role in identifying the most effective theranostic protocol for each patient. These advances have the potential to significantly improve patient outcomes and reduce treatment-related toxicities, bringing us closer to the goal of precision medicine in BC.
In conclusion, the current state of BC theranostics is very promising, and there are many exciting opportunities for future research in this area. The continued development and refinement of NP-based theranostic systems, combined with advances in personalized medicine, is likely to lead to significant improvements in the accuracy of diagnosis and the efficacy of BC treatment.
Abbreviations
Au NCs: |
gold nanoclusters |
BC: |
bladder cancer |
CDT: |
chemo-photodynamic therapy |
CT: |
computed tomography |
DDS: |
drug delivery system |
DOX: |
doxorubicin |
FA: |
folic acid |
FGFR3: |
fibroblast growth factor receptor 3 |
GNPs: |
gold nanoparticles |
HA: |
hyaluronic acid |
IONPs: |
iron oxide nanoparticles |
MFB: |
magnetic fluorescent bead |
miRNA: |
microRNA |
MOFs: |
metal-organic frameworks |
MRI: |
magnetic resonance imaging |
MSNPs: |
mesoporous silica nanoparticles |
N-GQDs: |
nitrogen-doped graphene quantum dots |
NIR: |
near-infrared |
NIRF: |
near-infrared fluorescence |
NPs: |
nanoparticles |
OCTA: |
optical coherence tomography angiography |
OEGMA: |
oligoethylene glycol methacrylate |
PA: |
photoacoustic |
PAMAM: |
polyamidoamine |
PCL: |
poly(ε-caprolactone) |
PDA: |
polydopamine |
PDT: |
photodynamic therapy |
PDX: |
patient derived bladder cancer xenograft |
PEC: |
photoelectrochemical |
PEG: |
poly(ethylene glycol) |
PEI: |
polyethyleneimine |
PLGA: |
poly(lactide-coglycolide) |
PNPs: |
nanoporphyrin platform |
PSs: |
photosensitizers |
PTT: |
photothermal therapy |
PTX: |
paclitaxel |
QDs: |
quantum dots |
ROS: |
reactive oxygen species |
SERS: |
surface-enhanced Raman scattering |
sFRP1: |
secreted frizzled-related protein 1 |
SNPs: |
silica nanoparticles |
SPR: |
surface plasmon resonance |
TdT: |
terminal deoxynucleoside transferase |
TURBT: |
transurethral resection of bladder tumor |
ZIF-8: |
zeolitic imidazolate framework-8 |
Declarations
Author contributions
KL, JR, and QY: Conceptualization. KL and QM: Writing—original draft. ZD and SL: Writing—review & editing. JR and QY: Funding acquisition.
Conflicts of interest
The authors declare that they have no conflicts of interest.
Ethical approval
Not applicable.
Consent to participate
Not applicable.
Consent to publication
Not applicable.
Availability of data and materials
Not applicable.
Funding
This work was financially supported by the
Copyright
© The Author(s) 2023.