Abstract
Over the last 60 years, only four new classes of antibiotics have been introduced, while the prevalence of antibiotic-resistant Gram-positive and Gram-negative bacteria has risen. This underscores the urgent need for new antibacterial therapeutics. This commentary leverages the recent exploration of γ-substituted-N-acylated-N-aminoethyl amino acid peptides (γ-AApeptides) to mimic the structures and function of natural antimicrobial peptides (AMPs), highlighting the promise and limitations for developing a new, effective treatment for antibiotic-resistant bacteria.
Keywords
Antimicrobial peptides, peptidomimetics, γ-AApeptides, amphipathicIntroduction
In 1939, gramicidin A as a peptide was discovered from the soil bacterium Bacillus brevis, marking the first commercially available antibiotic and a cornerstone for the development of antimicrobial therapeutics [1]. According to the Database of Antimicrobial Activity and Structure of Peptides (DAASP), over 22,000 peptides with antimicrobial properties have been identified to date [2]. Antimicrobial peptides (AMPs) are evolutionary biomolecules formed as part of the defense mechanisms of numerous organisms [3]. Despite their prevalence and diversity, AMPs typically contain fewer than 50 amino acids with positive charges ranging from +2 to +11, enabling interaction with negatively charged bacterial membranes through electrostatic interactions and hydrophobic residues for membrane disruption and penetration. AMPs could be characterized by their secondary structures, predominantly α-helix or β-sheet [4]. Through careful manipulation of hydrophobic and hydrophilic residues, AMPs exhibit broad-spectrum antimicrobial activity and immunomodulatory properties with negligible bacterial resistance and low toxicity [5]. However, AMPs face several limitations, including high manufacturing costs, high in vivo toxicity, low in vivo efficacy, susceptibility to proteolytic degradation, and rapid clearance [6].
Researchers are exploring various strategies to overcome these challenges. For instance, Nazeer et al. [7] reported the stabilization of macrocyclic AMPs through cross-linked swapping, enhancing conformation rigidity and antimicrobial activity via side chain-to-side chain lactam cross-links. Other approaches include transdermal delivery methods, such as incorporating nanoparticles to encapsulate AMPs, allowing for controlled release and resistance to enzymatic degradation [6]. The limitations of naturally occurring AMPs have driven the development of synthetic AMP mimetics, including small molecule AMP mimics, cyclic peptides (including stapled peptides), and peptidomimetics like γ-substituted-N-acylated-N-aminoethyl amino acid peptides (γ-AApeptides) developed by our lab (Figure 1). Among these, sulfonyl-γ-AApeptides are notable for forming α-helical secondary structures and exhibiting enhanced proteolytic degradation stability and cell permeability [8]. It could be a viable strategy to employ sulfonyl-γ-AApeptides to address the barriers surrounding AMPs and meet the growing need for therapeutics against drug-resistant bacteria. Alternatively, there have been several successful reported cases of synthetic peptidomimetics with potent antimicrobial activity, for example, Zhang et al. [9] demonstrated the eradication of Staphylococcus aureus from the incorporation of β-peptides. In 2022, Tallet et al. [10] reported a series of oligourea inspired peptides consisting of strategically distributed histidine arginine, tryptophan, and γ-valine residues to mimic the amphiphilic nature of AMPs. Very recently Firdous et al. [11] developed a series of short lipidated urea-containing α/β hybrid peptides which showed excellent antibacterial activity against both Gram-positive and Gram-negative bacteria.
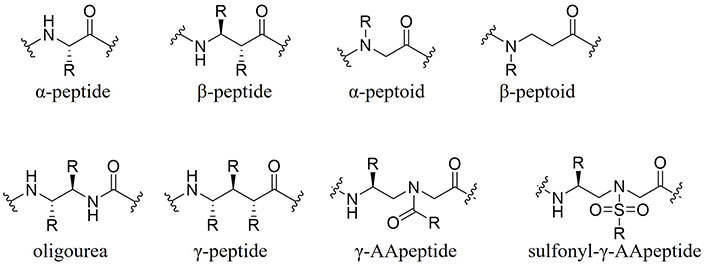
Structures of different peptidomimetic backbone scaffolds. γ-AApeptide: γ-substituted-N-acylated-N-aminoethyl amino acid peptide
Peptidomimetics as synthetic AMPs
Derived from feleucin-bombinin-1 (BO1), the cationic nonapeptide feleucin-K3 exhibits an amphiphilic α-helical structure with potent antimicrobial activity but fails to meet clinical therapeutic requirements due to low selectivity and poor stability. To this end, sulfonyl-γ-AApeptide building blocks were introduced at either the C or N terminus of the sequence with varying hydrophobic side chains to develop analogs. It was discovered that substituting the first two hydrophobic side chains of K3 with a sulfonyl-γ-AApeptide containing more hydrophobic side chains resulted in more potent antimicrobial activity, particularly against Gram-positive bacteria, K122 (Figure 2A). The addition of a sulfonyl-γ-AA unit led to negligible hemolytic activity, enhanced stability against mouse serum, and improved selectivity compared to K3 [12].
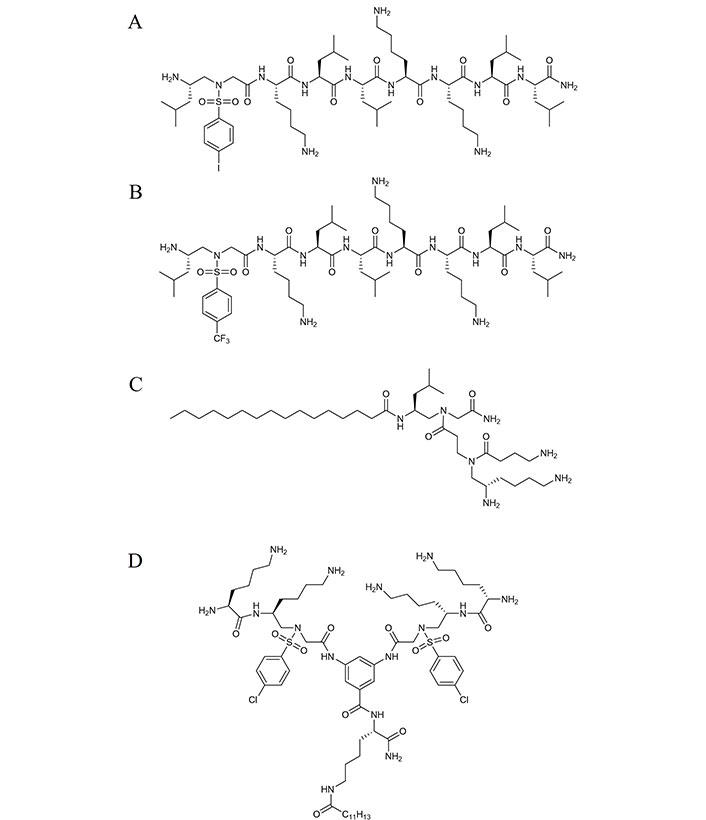
Structures of discussed antimicrobial peptides. (A) Structure of K122; (B) structure of CF3-K11; (C) structure of YW-11; (D) structure of compound 17
Within the world of medicinal chemistry, it is well known that by incorporating fluorine or fluorinated groups, one could potentially overcome protease degradation as well as substantially improve pharmacokinetic properties through its modulation of pKa influencing the bioavailability of a compound [13]. To this extent, authors theorized that by introducing fluorinated groups to sulfonyl-γ-AApeptide, a further enhanced feleucin-K3 inhibitor was developed. As seen in the structure of CF3-K11, it bears vast similarities to K122; however, it contains a fluorocarbon residue in the para position of a benzene ring (Figure 2B). This resulting influence of such a modification leads to the facilitation of hydrogen bonding stabilizing the secondary structure, as well as enhancing salt stability while maintaining its potent antibacterial activity [14].
A subclass of γ-AApeptides explored recently as potential antibacterial agents are short lipidated dendrimeric γ-AApeptides. We hypothesized that introducing a positively charged γ-AA building block to a lipidated hydrophobic γ-AA building block could mimic the amphiphilic nature of canonical AMPs. It was found that building blocks conjugated with C16 tails exhibited effective antimicrobial activity, particularly YW-1, which demonstrated the ability to disrupt cell membranes with good selectivity and low hemolytic toxicity (Figure 2C) [15]. The addition of a long hydrophobic tail on γ-AApeptides, a method our group first employed in 2012, showed unprecedented potential as a novel antibiotic therapeutic due to its large diversification potential, ease of synthesis, stability in the presence of serum, and potent selective activity against fungi and clinically relevant Gram-positive and Gram-negative bacteria [16]. Since then, functionalized γ-AApeptides were investigated to mimic not only the antimicrobial aspects of AMPs but also the immunomodulatory response, as seen in the cyclic γ-AApeptides we reported in 2014 [17].
To take advantage of the amphiphilic nature of lipidated sulfonyl-AApeptides, we examined the influence of dimerization to interact more strongly with the negatively charged surface of bacterial membranes. We synthesized a series of multiple dimeric lipo-α/sulfonyl-γ-AA hybrid peptides consisting of varying hydrophobic tail lengths, cationic groups, and hydrophobic achiral side chains to assess their influence on mimicking AMPs. From this series, we noted lead compound 17 possessed potent antibacterial activity with high selectivity towards bacteria cells. Similar to previously reported sulfonyl-γ-AA containing peptides, compound 17 exhibited excellent stability with seemingly no degradation after 24 h in the presence of serum (Figure 2D). The bacterial resistance was tested against a common antibiotic, ciprofloxacin, and revealed compound 17’s reduced susceptibility towards bacterial resistance. These promising results allow for further exploration of dimeric lipo-α/Sulfonyl-γ-AA hybrid peptides as potential AMP mimics [18].
Future directions
Despite significant advances in the development of AMP mimics, there have been no new FDA-approved antibiotic treatments. Our continued efforts to address the challenges associated with AMPs, such as toxicity, pharmacokinetic profiles, and low in vivo activity against drug-resistant Gram-negative bacteria, through the functionalization of γ-AApeptides are promising. However, our lab has greater success in targeting Gram-positive bacteria, such as methicillin-resistant Staphylococcus aureus (MRSA) and methicillin-resistant Staphylococcus epidermis (MRSE), likely due to the ability of amphiphilic peptidomimetics to penetrate and disrupt the thick peptidoglycan layer of Gram-positive bacteria. In contrast, Gram-negative bacteria, with their thin peptidoglycan layer protected by an outer membrane, present a more challenging target. It is our hope that through the fine-tuning of hydrophobic and hydrophilic residues, we can design peptidomimetics with potent broad-spectrum antibacterial activity as potential therapeutics. Looking forward, our efforts are also geared towards developing AMPs targeting specific bacteria such as Clostridium difficile. Overall, we hope to inspire future researchers to aid in the development of AMP mimetics as potential therapeutics towards the fight against drug-resistant bacteria.
Abbreviations
AMPs: | antimicrobial peptides |
γ-AApeptides: | γ-substituted-N-acylated-N-aminoethyl amino acid peptides |
Declarations
Author contributions
JF: Writing—original draft, Writing—review & editing. JC: Writing—review & editing, Supervision, Funding acquisition.
Conflicts of interest
The authors declare no conflicts of interest.
Ethical approval
Not applicable.
Consent to participate
Not applicable.
Consent to publication
Not applicable.
Availability of data and materials
All relevant data is contained within the manuscript.
Funding
This work was supported by NIH [2R01AG056569-06, 1R01GM150196]. The funders had no role in study design, data collection and analysis, decision to publish, or preparation of the manuscript.
Copyright
© The Author(s) 2025.
Publisher’s note
Open Exploration maintains a neutral stance on jurisdictional claims in published institutional affiliations and maps. All opinions expressed in this article are the personal views of the author(s) and do not represent the stance of the editorial team or the publisher.