Abstract
Aim:
As far as is known, the pharmaceutical effects of neem on human B-lymphoblastoid (TK6) cells have not been studied until now. Hence, the present study aimed to obtain neem phenolic extracts for inhibits the proliferation of TK6 cells and explore some possible underlying mechanisms involved in these effects.
Methods:
Hexane extract (HE) was obtained in the first step. After that, the residual hexane was removed from the neem. The dried neem sample was used in a new extraction for obtaining the ethyl acetate extract (EAE). Total phenolic compounds (TPC) and total flavonoid contents (TFC) were determined by spectrophotometric methods. Lactate dehydrogenase (LDH) and 3-(4,5-dimethylthiazol-2-yl)-2,5-diphenyltetrazolium bromide (MTT) tests were used to evaluate the cytotoxicity in TK6 cells. The stop at G0/G1 cell cycle and inducing apoptosis in the TK6 cells were analyzed by flow cytometry. For deoxyribonucleic acid (DNA) damage evaluation, the alkaline comet test was used.
Results:
The higher TFC (65.50 mg/g of extract ± 1.17 mg/g of extract) and TPC (52.08 mg of extract ± 0.88 mg of extract) were obtained in EAE compared to HE that was obtained TFC of 14.61 mg/g of extract ± 0.60 mg/g of extract and TPC of 3.20 mg/g of extract ± 1.20 mg/g of extract. EAE was more significantly cytotoxic to TK6 cells than HE. The apoptosis induction was higher after exposure to 15.0 µg/mL of EAE (11.29%) in comparison to 15.0 µg/mL of HE (2.52%). The G0/G1 phase increased from 72% negative control (NC) to 83% after treatment with neem extracts (15 µg/mL). Neem extracts were also able to cause DNA strand breaks in TK6 cells.
Conclusions:
The extraction residue from neem leaf after hexane extraction is a source important of cytotoxic and genotoxic molecules against TK6 cells, the results also can suggest that the toxic effects in TK6 cells can be provided most likely due to the presence of high content of TPC from neem extracts.
Keywords
Medicinal plant, bioactive extracts, sequential pressurized fluids, antiproliferative effect, human B-lymphoblastoid cellsIntroduction
Cancer is a major cause of global mortality and morbidity [1]. According to data collected by the World Health Organization, the annual worldwide incidence of cancer new cases has been estimated at 19.3 million by 2025 and is expected to reach 28.9 million by 2040. Among the cancer types most frequently detected are lung, breast, colorectal, stomach, liver, cervical, and blood cancers [2, 3]. Lymphoma and leukemia are hematologic malignancies more common in childhood and young adults [4] and can have as precursors B-lymphoblastic cells [5].
In this context, much research has been conducted to better understand the risk factors associated with cancer and identify new or existing chemicals that may contribute to cancer prevention and/or treatment [3, 6]. Therefore, the cancer chemopreventive potential of natural phytochemicals is of major interest [7, 8].
The majority of the active ingredients of medicines have been from natural sources. As well as the larger part of drug substances are inspired by a natural molecule [9, 10]. Associated with this, the use of natural compounds for disease treatment has become, in the last decades, a popular topic of research [11] with different works showing that phenolic compounds, such as catechins and other flavonoids may present anticancer properties [8, 12, 13]. These properties have been associated with different molecular mechanisms, which include modulation of cancer cell signaling, inhibiting the progression of the cell cycle, apoptosis induction, and modulation of enzymatic activity [14].
The flowers, seeds, bark, fruit, and leaves from medicinal plants such as Azadirachta indica A. Juss (neem) have been a natural drug source for over 4,500 years. Neem has a high content of bioactive molecules, they have been associated with carcinogen-detoxification, free radical scavenging, deoxyribonucleic acid (DNA) repair, immunomodulatory, anti-inflammatory, antiangiogenic, cell-cycle arrest, cell death induction, and antimetastatic effects [15].
Neem compounds showed higher activity and specificity in tumor cells than the cisplatin antitumor agent [16, 17]. However, phenolic compounds are present in neem at high levels and seem to be significantly involved in the medicinal effects of this plant [9]. In any case, it is important to note that the amount and composition of polyphenolic compounds from vegetable matrices may considerably differ according to the polarity of the used solvent and extraction process [15, 18].
Pressurized liquid extraction (PLE) is an extraction process that is potential to recover natural compounds. For the PLE, different solvents have been used under high pressure to enhance the effectiveness of the bioactive compound extraction [19, 20]. Several solvents such as ethanol, water, hexane, ethyl acetate, and chloroform have been used for obtaining bioactive molecules, including terpenes and polyphenols from neem [15, 21, 22].
The use of extraction processes in only one step can result in the inefficient separation of bioactive molecules from the biomass, due mainly to the distinct polarity of the phytochemical compounds present in the plants [18, 23]. Hexane has been used in the first step to removing nonpolar molecules from vegetal matrices. The residual biomass after hexane extraction can be used in a new extraction with ethyl acetate to obtain an extract richer in phenolic compounds [18].
After hexane extraction, the residual biomass from neem leaves has been reported as a potential source of antiproliferative molecules [21]. However, there is little information available in the literature about the cytotoxic and genotoxic effects of neem leaf extracts, obtained with ethyl acetate after hexane extraction, under high pressure, on human B-lymphoblastoid (TK6) cells. Hence, the present study aimed to obtain neem leaf extracts by PLE, in a fixed bed, using hexane and ethyl acetate sequentially, to determine its total polyphenol contents, and to investigate their cytotoxic potential on TK6 cells, while exploring some possible underlying mechanisms related to these effects.
Materials and methods
Chemical reagents
The hexane and ethyl acetate were bought from Vetec (Rio de Janeiro, Brazil). Ultrapure water was treated in a Milli-DI® Water Purification System (Millipore® Bedford, MA, USA). Lactate dehydrogenase (LDH) was bought from Millipore Sigma. Folin-Ciocalteu, sodium carbonate, gallic acid (GA), aluminum chloride (AlCl3), sodium nitrite, sodium hydroxide (NaOH), epicatechin, 3-(4,5-dimethylthiazol-2-yl)-2,5-diphenyltetrazolium bromide (MTT), propidium iodide (PI), low and normal melting agarose, disodium ethylenediaminetetraacetate dihydrate (Na2EDTA), ribonuclease (RNase), Triton™ X-100, NaOH, and Tris-base were all obtained from Sigma-Aldrich® (St. Louis, USA). Fetal bovine serum (FBS), streptomycin-penicillin and trypsin-ethylenediaminetetraacetic acid (EDTA) solution were acquired from Biochrom KG (Berlin, Germany). Dimethyl sulfoxide (DMSO) was bought from VWR Chemicals (Solon, OH, USA). Phosphate-buffered saline (PBS) was bought from Lonza (Lutterworth, UK). SYBR™ Gold was supplied by Thermo Fisher Scientific (Madrid, Spain).
Vegetable material and PLE
Neem leaf samples were harvested in the year 2015 and kindly provided by Embrapa Coastal Tablelands (Brazilian Agricultural Research Corporation), in Aracaju, Sergipe, Brazil. After this, neem samples were dried for 36 h at 45℃ in a kiln with hot air circulating and then milled up to particle size ranges from 0.5 mm to 1.0 mm.
The sequential PLE process was performed using 20 g of neem leaf in a stainless-steel extractor with an internal volume of about 100 mL at 25℃ and the flow rate was set at 1.0 mL/min for 60 min under 100 bar, for each solvent. The hexane extract (HE) was obtained in the first step. After this, the residual hexane was removed from the neem sample by using carbon dioxide (CO2) under depressurization from 30 bar to 0 bar. The dried neem sample was used in a new extraction carried out with ethyl acetate at 25℃, a flow rate of 1.0 mL/min for 60 min under 100 bar, for obtaining the ethyl acetate extract (EAE). Thereby, obtaining two extracts from the same neem mass was possible using two distinct solvents (hexane and ethyl acetate). The hexane and ethyl acetate solvents were evaporated from their extracts in a hot-air circulation oven at 40°C [21]. All the extracts obtained were frozen at –20°C until analysis.
Determination of total phenolic and flavonoids contents
Total phenolic compounds
The total phenolic compounds (TPC) were analyzed by using Folin-Ciocalteu’s method. For this, 30 µL of neem extract solution (100 µg/mL) was mixed with 150 µL of 10% Folin-Ciocalteu’s reagent. Sequentially, 120 µL of 7.5% sodium carbonate solution was added, and the mixture was incubated for 15 min at 45℃ and then kept for 30 min at room temperature. The TPC was performed in a microplate reader (Synergy™ HT, BioTek, USA) at 765 nm and its quantification was carried out according to the calibration curve (y = 0.012x – 0.0145, R2 = 0.998) for GA was built on a series concentration ranging from 5 µg/mL to 100 µg/mL. The result was reported as mg of gallic acid equivalents per g (mg GAE/g) of neem extract [24].
Total flavonoid contents
Total flavonoid contents (TFC) were measured by using the AlCl3 method. For this assay, 30 µL of neem extract (10-4) was mixed with 45 µL of 1% sodium nitrite and then 75 µL of ultrapure water was added. The mixture was allowed to stand for 5 min at room temperature in the absence of light. Sequentially, 45 µL of AlCl3 was added and kept for 1 min; finally, 60 µL of NaOH and 45 µL of ultrapure water were added. The absorbance (Abs) of the mixture was measured at 510 nm using a microplate reader (Synergy™ HT, BioTek, USA). For the quantification of TFC, a calibration curve (y = 0.0015x + 0.0047; R2 = 0.999) for epicatechin at concentrations ranging from 5 µg/mL to 300 µg/mL was used and the value was reported as mg of epicatechin equivalents per g (mg ECE/g) of neem extract [24].
Cell culture
The TK6 cells used in this study originated from the European Collection of Cell Cultures (Cat. No. 95111735). TK6 cells were cultured in the atmosphere of 5% CO2, 37°C in Roswell Park Memorial Institute (RPMI)-1640 medium (Merck, Darmstadt, Germany) supplemented with 2% L-glutamine, 10% FBS, and 1% penicillin/streptomycin.
Cell treatment
TK6 cells were seeded with the density of approximately 25,000 cells per well in 96-well microplates (for analyzes of cell viability by the MTT and LDH) and 40,000 cells per well in 12-well microplates (for the cell cycle, apoptosis, and comet assays) and then incubated overnight. On the following day, cells were incubated for 48 h with neem extracts (dissolved in DMSO) at concentrations ranging from 2–25 µg/mL. All experiments for MTT and LDH assays were carried out using negative control (NC) prepared with 1% DMSO and positive control (PC) with 1% Triton™ X-100, both prepared in the cell culture medium.
MTT assay
The cell viability of the neem extracts was assessed by MTT assay, according to Mosmann [25], 1983. After incubation for 48 h, the microplate was centrifuged (200 g) for 5 min. Sequentially, neem extracts were removed and 200 µL of MTT (0.5 mg/mL) were added to each well. The microplate was incubated at 37℃ for 4 h in the absence of light, and then centrifuged (200 g) for 5 min. Afterward, the supernatant was removed, and the formazan crystals produced were dissolved into 100 µL of DMSO. Abs was measured at 570 nm in a Cambrex ELx808 microplate reader equipped with KC4 Data Analysis Software (Biotek, Winooski, VT, USA) [26]. Cell viability (%) was calculated as follows: (Abs-sample/Abs NC) × 100.
LDH leakage assay
Membrane integrity was detected by measuring LDH released from TK6 cells after neem extract treatment. LDH from dead cells that leaked was quantitatively assessed by cytotoxicity colorimetric kit (Millipore Sigma, Roche, Cat. No. 11644793001), according to the manufacturer’s procedure. Abs was measured in a Bio-Rad model 680 microplate reader at 490 nm using 655 nm background correction. The results were expressed as LDH release (%) and calculated according to Malhão et al. [27].
Cell-cycle analysis and apoptosis detection
After treatment, TK6 cells were centrifuged (1,000 g) for 5 min. Then, the cells were resuspended in PBS and fixed in pre-chilled 70% ethanol at 4ºC overnight. Sequentially, the suspension cell was centrifuged for 5 min at 1,000 g. After PBS, the cell pellets were centrifuged (1,000 g) again for 5 min. The collected pellets were resuspended in 500 µL of staining solution of PI at 40 µg/mL and RNase (50 µg/mL), prepared in PBS (flow cytometry grade) and incubated for 30 min at 37°C. Cells (%) in each cell cycle phase [sub-G1 (apoptosis), G0/G1, S, and G2/M] were measured using a Guava® easyCyte™ 8HT Flow Cytometer (Luminex, Austin, TX, USA), according to Valdiglesias et al. [28]. For this, a minimum of 10,000 events were acquired and evaluated using Guava™ Software version 3.1.1.
Genotoxicity assessment—comet assay
To evaluate the DNA damage, the alkaline comet assay was performed according to Costa et al. [29], with slight modifications. TK6 cells treated with neem extracts for 48 h were washed twice with prechilled PBS. Sequentially, TK6 cells were centrifuged at 1,000 g for 5 min. Cell pellets obtained were resuspended in 200 µL of 0.6% low melting point agarose (LMA). Then, the cells were dropped onto a frosted slide previously coated with 1% normal melting point agarose (2 replicates in each slide). The slides were placed on ice for 5 min and then immersed in a lysis solution [100 mmol/L EDTA, 2.5 mol/L sodium chloride, 10 mmol/L Tris-base, 10 mol/L NaOH (pH 10), and 1% Triton™ X-100] at 4℃ for 1 h, in the absence of light. Sequentially, slides were placed on a horizontal tank and immersed in a freshly made alkaline electrophoresis solution (0.3 mol/L NaOH and 1 mmol/L Na2EDTA) for 40 min in the absence of light. Electrophoresis was performed for 20 min at 1.15 V/cm. Then, the slides were washed for 10 min with prechilled PBS at 4℃, 10 min with prechilled ultrapure water at 4℃, fixed with 70% and 96% ethanol for 15 min (for each solvent) at room temperature, and dried overnight. Before analysis, slides were stained with SYBR™ Gold for 30 min. After that, the slides were visualized at a magnification of 250× using a Nikon Eclipse E400 microscope equipped with an epi-fluorescence attachment and analyzed by using Comet Assay IV software (Perceptive Instruments, Suffolk, UK). At least 100 cells were scored and DNA in the comet tail (TDNA) was used as the DNA damage parameter (%) [30].
Statistical analysis
Results are shown as a mean ± standard error of the mean (SEM). Statistical analysis was performed with GraphPad Prism 5.0 (La Jolla, California, USA). A unidirectional variance analysis (ANOVA), followed by a post hoc Tukey’s test, was carried out to verify the differences between the control and exposed cells. The in vitro cytotoxicity at half-maximal inhibitory concentration (IC50) value was obtained through nonlinear regression of the dose-response curve fit. The significant difference was concluded at a P < 0.05.
Results
Total phenolic and flavonoid contents
In general, the results of this present study demonstrated that flavonoids and other phenolic compounds were present in and EAE extracts (Figure 1). In the HE, the TPC-HE and TFC-HE were respectively 3.20 mg/g of extract ±1.20 mg/g of extract and 14.61 mg/g of extract ± 0.60 mg/g of extract. A new extraction using ethyl acetate, in the same sample extracted by hexane, allowed a significantly higher amount of TFC-EAE (65.50 mg/g of extract ± 1.17 mg/g of extract) and TPC-EAE (52.08 mg/g of extract ± 0.88 mg/g of extract).
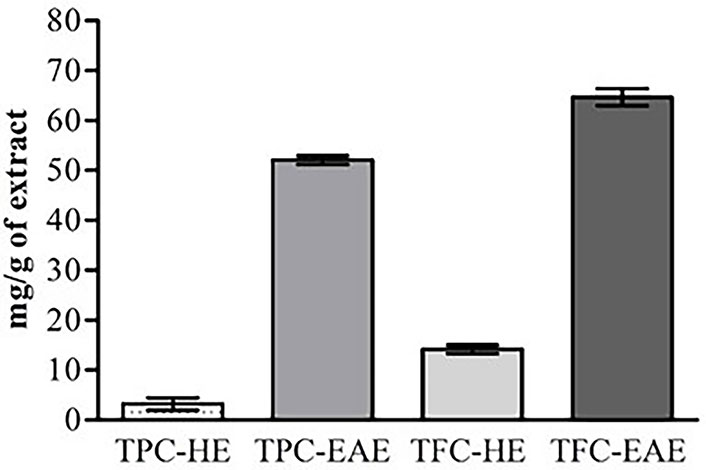
Polyphenol concentrations in neem extracts. All values are reported as mean ± SEM. TPC-HE: total phenolic compounds of HE; TPC-EAE: total phenolic compounds of EAE; TFC-HE: total flavonoid content of HE; TFC-EAE: total flavonoid content of EAE
Cytotoxicity analysis
This study used MTT and LDH assays to identify cytotoxicity in TK6 cells. According to the results of the MTT assay (Figure 2), HE and EAE significantly decreased TK6 cell viability at concentrations between 15 µg/mL and 25 µg/mL; this decrease in viability is more pronounced after exposure to EAE. The EAE at 25 µg/mL was more significantly cytotoxic to TK6 cells than HE at 25 µg/mL. However, EAE at 25 µg/mL showed a similar result to PC. This result is indicative of a high level of cell damage and toxicity caused by EAE at 25 µg/mL.
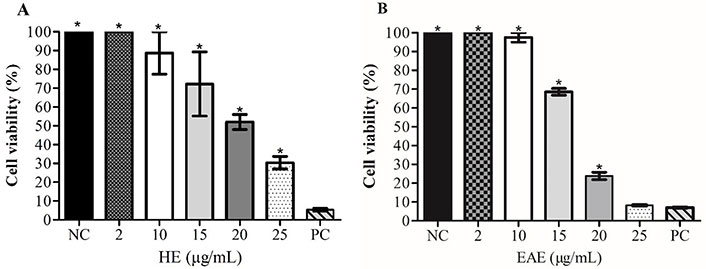
Cell viability (%) of TK6 cells treated with HE (A) and EAE (B), determined by MTT assay. 1% Triton™ X-100 was used as the PC; cell culture medium with 1% DMSO as the NC. Values reported as a mean ± SEM; * Significant difference to the NC (P < 0.05)
IC50 values were calculated based on these results and were 18.88 µg/mL ± 0.35 µg/mL for HE, and 16.62 µg/mL ± 0.05 µg/mL for EAE. Three concentrations of each extract were chosen for the other experiments based on the results obtained in the cell viability experiments (IC50).
LDH level was performed to quantitatively assess by measuring the activity of LDH released from the damaged or dead TK6 cells after exposure to concentrations from 10 µg/mL to 20 µg/mL of HE and EAE for 48 h. As shown in Figure 3, the EAE 20 µg/mL caused a more significant LDH leakage to TK6 cells than HE 20 µg/mL. LDH leakage increased significantly from 30.51% ± 1.82% to 62.31% ± 6.41% after exposure to EAE 15 µg/mL and EAE 20 µg/mL, respectively.
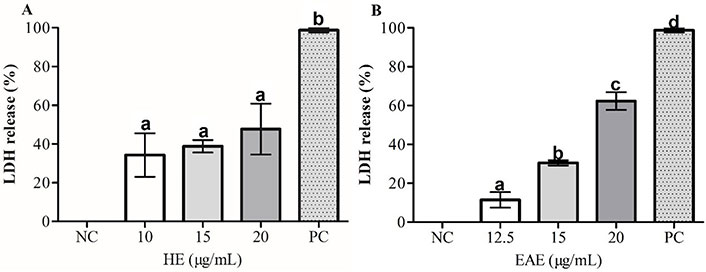
Effects of (A) HE and (B) EAE on the membrane integrity of TK6 cells evaluated using the LDH release assay. 1% Triton™ X-100 was used as the PC. DMSO values were normalized considering NC as 100%. The values are reported as mean ± SEM. Equal letters (a, b, c, and d) in the same chart indicate that there are no significant differences (P < 0.05) in LDH release (%)
Analysis of apoptosis induction and cell cycle distribution
The possible effect of HE and EAE on the induction of cell apoptosis was investigated by evaluation of subregion G0/G1 of the cell cycle distribution, indicating fragmentation of DNA in late apoptotic stages [31, 32]. In all tested concentrations, the cells in the sub-G0/G1 (%) region increased after treatment with HE and EAE (Figure 4A and B).
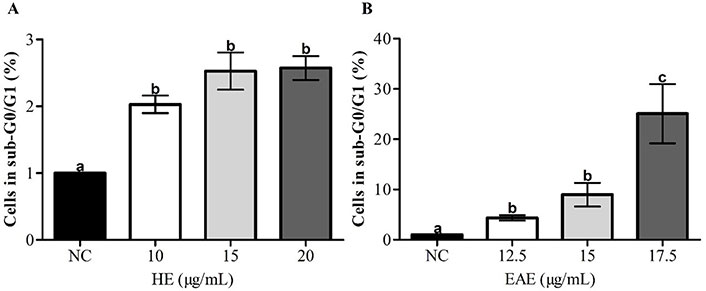
Apoptosis (sub-G0/G1 region) in TK6 cells, after 48 h of treatment with HE (A) and EAE (B), assessed by flow cytometry. Cell culture medium with 1% DMSO was used as NC. The values are reported as mean ± SEM. Different lowercase letter (a, b, and c) in the same chart indicates significant differences (P < 0.05)
The induction of apoptosis was higher after exposure to EAE when compared to HE; the treatment with the same concentration (15.0 µg/mL) of HE and EAE led to 2.52% and 11.29% of cells in the subregion G0/G1, respectively. In addition, EAE induced apoptosis in a dose-dependent which was not seen after treatment with HE.
The results in Figure 5A and B show that both extracts, HE and EAE, were able to alter the TK6 cell cycle after a treatment of 48 h, with an arrest in the G0/G1 phase. The cells (%) in G0/G1 phase increased from 72% (NC) to 83% after treatment with 15 µg/mL of either HE or EAE. In consequence, a reduction in the S and G2/M phases was observed in all tested conditions.
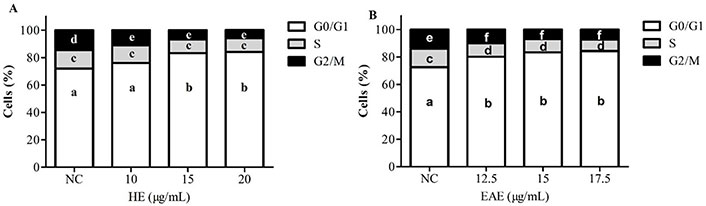
Cell cycle analysis in TK6 cells treated with HE (A) and EAE (B) for 48 h [cells (%)]. DMSO (1%) was used as NC. The values are reported as mean ± SEM. Different lowercase letter (a, b, c, d, e, and f) in the same chart indicates significant differences (P ˂ 0.05) on the cell-cycle phase
DNA damage
The results in Figure 6A and B exhibit the DNA damage in TK6 cells after a 48-h treatment with various concentrations of EAE and HE.
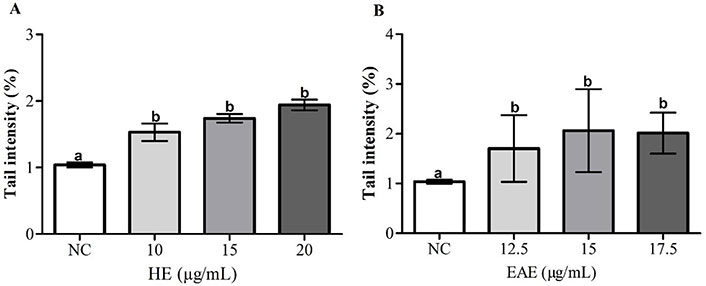
DNA damage [tail intensity (%): fold change negative to NC] of TK6 cells after treatment with HE (A) and EAE (B) during 48 h. DMSO (1%) was used as NC. The values are reported as mean ± SEM. The same lowercase letter (a and b) in the same chart indicates no significant differences (P > 0.05)
DNA damage increased significantly in TK6 cells after treatment with HE and EAE, compared to the NC in all tested concentrations. The induced DNA strand breaks were similar for both HE and EAE.
Discussion
Initially, a new extraction process has been performed in this study, aiming to obtain neem leaf extracts. The TPC and flavonoid contents were used to characterize the obtained extracts. The results presented in Figure 1 are in accordance with Arsul et al. [33], which also reported lower TFC and TPC in the HEs compared to the EAE. Arsul et al. [33] found the TFC (mg QE/g extract) of HEs ranging from 5.83 ± 0.10 (red varieties of bajakah) to 20.07 ± 1.26 (white varieties of bajakah) and TPC (mg GAE/g extract) from 84.57 ± 1.26 (red varieties of bajakah) to 172.49 ± 3.35 (white varieties of bajakah). The TFC (mg QE/g extract) of EAEs were ranging from 15.17 ± 0.17 (red varieties of bajakah) to 34.25 ± 0.36 (white varieties of bajakah) and TPC (mg GAE/g extract) from 217.98 ± 7.95 (red varieties of bajakah) to 653.53 ± 6.00 (white varieties of bajakah).
The study performed by Shewale et al. [34] using neem leaves obtained the TPC of 11.87 mg of GA/g of powder by using ultrasound-assisted extraction at 40ºC with 70% ethanol for 14 min.
Hemdan et al. [35] reported that phenolic compounds from HPLC analysis of neem leaves ethyl acetate fractions were GA, protocatechuic acid, p-hydroxybenzoic acid, catechin, chlorogenic acid, caffeic acid, syringic acid, vanillic acid, cinnamic acid, ferulic acid, rutin, quercetin, kaempferol, and chrysin.
This different feature can be due to the positive effect of the high pressure used in this study to extract the phenolics. These natural compounds are important active molecules against the growth of malignant cells [36].
Polyphenols can exert cytotoxic effects against cancer cells inhibiting tumor proliferation. Notably, polyphenols induce apoptosis and cell cycle arrest. These natural molecules interfere at the initial and progressive stages of cancer due to modulating different signaling pathways related to cell proliferation and cell death. Polyphenols play a chief role in the activation of gene p53 in the human body, which contributes to cancer control [37].
Plant flavonoids have anticarcinogenic effects due to their interfering with the development of cancer caused by the inhibition grown of cellular proliferation and apoptosis induction, for example [38].
Previous results from Santos et al. [21], showed that neem extracts, obtained by extraction procedure in three steps (1. hexane; 2. ethyl acetate; and 3. ethanol/water), presented a more selective antiproliferative activity for cancer cells than for non-tumor cells. Herein, neem extracts obtained by PLE were tested in vitro against a human leukemia cell line (TK6; human lymphoblastoid cell line), a widely used model to test chemotherapeutics cytotoxicity and genotoxicity [39].
The presented results support that both neem extracts (HE and EAE) can decrease TK6 viability, being this activity more evident in the EAE. Considering the total phenolic and flavonoid contents of each extract, it can be hypothesized that the increased EAE cytotoxic potential to TK6 cells may be correlated to its higher concentration of these bioactive molecules, and/or to a synergistic effect with other antiproliferative molecules also present in the extract. In fact, the literature indicates that neem leaves contain more than 140 different bioactive compounds [40–42]. The cytotoxic potential here observed has been previously reported on other cancer cell lines, such as prostate cancer cell line (PC-3) [43], breast adenocarcinoma (MCF-7), non-small cell lung cancer (NCI-H460), cervical carcinoma (HeLa), and hepatocellular carcinoma (HepG2) [21].
In addition to cytotoxicity, apoptosis, cell cycle alterations, and DNA damage induced by treatment with neem extracts were also explored in this study, as they are important targets for cancer therapy [44]. Both extracts significantly increase cells in the sub-G0/G1 (%), suggesting that neem can induce apoptosis. Similar results have already been reported by other authors [45].
In the case of neem extracts, apoptosis may occur by intrinsic and extrinsic pathways, depending on the concentration and type of bioactive compounds [46, 47]. As for cytotoxicity, EAE also induced higher levels of apoptosis than HE; again the content of total polyphenols seems to be at the basis of this result.
Polyphenols contribute to stopping at G0/G1 cell cycle due to the downregulation of cyclin D1, cyclin-dependent kinase (CDK) 4, 6, and the upregulation of the CDK inhibitors such as p21 and p27 [32, 48]. In line with this, in the present study, neem extracts were also responsible for a G0/G1 arrest. Notably, the G1 phase is a period that influences cell division, and therefore, an arrest in G0/G1 positively contributes to controlling malignant cell growth [49]. The results obtained in this study show that HE and EAE were also able to cause genotoxicity, a common mechanism of chemotherapeutics; in line with this, other studies have reported that plant polyphenols can catalyze DNA breakage in malignant cells as an anticancer mechanism [50, 51]. Moreover, DNA damage accumulation may also be associated with cell cycle arrest and apoptotic cell death [52].
According to the results of this present study, after the hexane extraction, the neem residual biomass contains cytotoxic and genotoxic molecules, able to be extracted by other solvents with different polarities such as ethyl acetate. EAE showed higher total phenol contents than HE. EAE and HE revealed potential cytotoxicity for TK6 cells. Its antiproliferative mechanisms can promote cell cycle arrest and induction of cell death, mainly by apoptosis. However, EAE showed higher antiproliferative effects than HE. EAE seems to be interesting for the development of promising drugs against malignant hematologic cells. In general, the findings of the present study indicate that neem extracts (HE and EAE) obtained through PLE are able to induce cytotoxicity, associated with apoptosis, cell cycle alterations, and genetic damage. Considering these results, further evaluation of neem extracts as chemotherapeutics candidates is needed.
Abbreviations
Abs: |
absorbance |
AlCl3: |
aluminum chloride |
DMSO: |
dimethyl sulfoxide |
DNA: |
deoxyribonucleic acid |
EAE: |
ethyl acetate extract |
GA: |
gallic acid |
HE: |
hexane extract |
IC50: |
half-maximal inhibitory concentration |
LDH: |
lactate dehydrogenase |
mg GAE/g: |
mg of gallic acid equivalents per g |
MTT: |
3-(4,5-dimethylthiazol-2-yl)-2,5-diphenyltetrazolium bromide |
NaOH: |
sodium hydroxide |
NC: |
negative control |
PBS: |
phosphate-buffered saline |
PC: |
positive control |
PLE: |
pressurized liquid extraction |
SEM: |
standard error of the mean |
TFC: |
total flavonoid contents |
TK6: |
human B-lymphoblastoid |
TPC: |
total phenolic compounds |
Declarations
Author contributions
KSS: Methodology, Investigation, Writing—original draft, Visualization. CC: Methodology, Data curation, Writing—original draft. MJB: Methodology. JPT: Methodology, Data curation. AVCdSM: Writing—original draft. FFP: Conceptualization, Supervision. CD: Writing—review & editing, Supervision. MBPPO: Conceptualization, Writing—review & editing, Supervision.
Conflicts of interest
The authors declare that there are no conflicts of interest.
Ethical approval
Not applicable.
Consent to participate
Not applicable.
Consent to publication
Not applicable.
Availability of data and materials
Not applicable.
Funding
The
Copyright
© The Author(s) 2023.