Abstract
This paper reviews the nutritional quality and safety of edible farmed insects from the point of view of the Czech-Slovenian bilateral project: Quality, Safety and Authenticity of Insect Protein-based Food and Feed (INPROFF). Insects as a sustainable source of dietary protein for animal feed and even humans, when integrated into the European agrifood system, could offer a solution to Europe’s feed protein deficit and help alleviate environmental pressure from increasing protein demand, such as declining availability of land, water, marine and energy resources, the overuse of pesticides and reduced biodiversity. However, despite a growing interest in the European Union (EU) in farming edible insects, many economic, scientific, technological, and social barriers remain. In response, Slovenia, represented by the Jožef Stefan Institute, the Biotechnical Faculty of the University of Ljubljana, and Jata Emona d.o.o. (the leading supplier of feedstuffs for the Slovenian market), a country with no history of insect rearing but an interest in alternative protein, joined with the Czech University of Life Sciences Prague, representing the Czech Republic—a country with a history of insect rearing and research into edible insects—to establish INPROFF a three-year bilateral project that aims to close the knowledge gap regarding the quality and safety of insect-based products and boost the farmed insect food and feed value chain. Specifically, it comprises three thematic pillars: (P1) nutritional enhancement, (P2) safety and quality and (P3) authenticity, traceability, and consumer acceptance. The paper also discusses the gaps in the metrological challenges of analysing insects, which will be critical for ensuring safety, quality, and sustainability. The paper finds that although much work has been done, many exciting avenues remain for new research.
Keywords
Farming insects, alternative protein, quality, fermentation, safety, authenticity, traceability, metrologyIntroduction
As demand for sustainable protein intensifies, many food scientists propose using farmed insect protein for animal feed and even for human consumption. Moreover, in 2013, the Food and Agriculture Organisation (FAO), a part of the United Nations, published a report recommending consuming insects as a sustainable solution to the shortage of world food supplies [1] and are now generally accepted as a sustainable way to help alleviate Europe’s feed protein deficit having a significant role in the EU’s protein strategy [2–5].
Unlike wild-caught insects in many parts of the world, farmed insects in Europe are considered (legally) no different from other farmed animals and must comply with National and EU legislation, and although a full review of the EU legislation is beyond the scope of this paper, the growth of the farmed insect industry will be significantly impacted by the legislation. Historically, given that insects were not consumed in the countries that make up the EU, they are covered by Regulation (EU) 2015/2283 on Novel Foods [6], which governs the authorisation and marketing of novel foods, including insects, within the EU following an assessment by the European Food Safety Authority (EFSA) and, as of the time of writing, it includes yellow mealworm (Tenebrio molitor), house cricket (Acheta domesticus), migratory locust (Locusta migratoria) and the buffalo worm (Alphitobius diaperinus). Producers must also comply with, among others, regulations regarding general principles and standards governing food and feed safety (Regulation [European Commission (EC)] 178/2002), hygiene rules [Regulation (EC) 852/2004], animal health [Regulation (EU) 2016/429], proper labelling with regards allergen information, nutrition labelling, and country of origin labelling [Regulation (EU) 1169/2011]; importation [Regulation (EU) 2019/626], and use of animal by-products [Regulation (EU) 142/2011] [7–12].
Strict regulations also exist regarding animal feed, including placing feed on the market concerning safety, labelling, and traceability requirements [Regulation (EC) No 767/2009] and a catalogue of authorised feed materials, which includes insects and their products, that can be used in animal feed formulations [Regulation (EU) No 68/2013] [13, 14]. Moreover, in the EU, insect protein is classified as processed animal protein (PAP), and its use, production, and labelling are strictly controlled under specific rules [Regulation (EU) 2017/893] [15]. Insects approved for animal feed in aquaculture include black soldier fly (Hermetia illucens), yellow mealworm, common housefly (Musca domestica), lesser mealworm (Alphitobius diaperinus), house cricket, field cricket (Gryllus assimilis) and, as of 2024, the silkworm (Bombyx mori) pupae are authorised for use in aquaculture feed. The recent changes to the regulations allowing certain insect PAPs in pig and poultry feed represent a boon for the farmed insect industry. However, ruminants still cannot feed insects due to transmissible spongiform encephalopathies (TSEs). Overall, many producers and promoters of edible insects feel that legislation regarding what substrate (e.g., food waste) insects can be fed is restrictive and is holding back the field [2]. A detailed review of all the regulations relating to EU law is published by the International Platform of Insects for Food and Feed (IPIFF) [16].
Even though insects are now on the market and have passed scrutiny by EFSA, there remain many gaps in the knowledge regarding farmed insects and many challenges in improving their economic viability (feed modification) and acceptability to the consumer. In response, in 2021, the Jožef Stefan Institute (JSI), the Biotechnical Faculty of the University of Ljubljana (UL-BF), the Czech University of Life Sciences Prague (CZU) and Emona Nutrition Research & Development Department of Jata Emona d.o.o. (ENR-JE), Slovenia’s leading feed producer established a joint three-year bilateral research project INPROFF: Quality, Safety and Authenticity of Insect Protein-based Food and Feed. The project, jointly funded by the Slovenian Research Agency (ARIS) and The Czech Science Foundation (GAČR), is designed to generate new knowledge and stimulate scientific cooperation. It employs various strategies to close the knowledge gap regarding the quality and safety of insect-based products. The project focuses on the yellow mealworm, house cricket and migratory locust. These were chosen because they are already on the market and are relevant as both food and feed.
At the project’s core are three thematic pillars: (P1) nutritional enhancement, (P2) safety and quality and (P3) authenticity, traceability and consumer acceptance, all of which are underpinned by one horizontal activity: (H1) insect production and characterisation (Figure 1). Pillar I seeks to optimise the nutritional quality of insect biomass by investigating the rearing of insects on both conventional and alternative feeds and through fermentation of their biomass. Pillar II is concerned with the safety of farmed insects by developing advanced analytical protocols to assess their chemical and biological safety. Finally, Pillar III is about developing forensic tools to verify the authenticity of insect-based products, promoting transparency and trust in the market, as well as seeking to understand consumer attitudes and acceptance towards eating insects. Moreover, the project is intended to catalyse future collaborations for continual advancements in insect farming research and innovation.
In this paper, we review the current state-of-the-art and challenges regarding farmed edible insects pertaining to the three thematic pillars of the INPROFF project. Among four primary academic peer-reviewed databases: Google Scholar, Scopus, Web of Science, and Science Direct, Scopus was selected as it is one of the leading international and prominent databases, familiarity, and delivered a significant number of hits for the search term “edible insects” (Table 1). The search term “edible insects” although it includes wild-caught species not covered by this review, was a better catch-all term than “farmed insects” and “entomophagy”. The search was restricted to the following fields: Title, Abstract, and Author Keywords. Acceptance criteria included all articles published in English between 2004 and 2024. The analysis identified over 2,000 papers going back 20 years (Table 1). A comprehensive list of search terms is provided in the supplementary information (Table S1), while Table S2 presents the search results (hits) for the term “edible insects” along with the studied species. The findings indicate that the most studied species is “Tenebrio molitor”.
Search results (hits) for the search term “edible insects” in different databases and results of connected terms performed in Scopus (Search fields: article title, abstract, keywords)
Search term | Hits (2004–2024) | |
---|---|---|
“Edible insects” | ||
Google Scholar | 1,710 | |
Scopus | 2,011 | |
Web of Science | 1,401 | |
Science Direct | 673 | |
“Insects” | 236,679 | |
“Edible insects” | 2,011 | |
“Entomophagy” | 866 | |
“Farmed insects” | 43 | |
“Edible insects” AND … | ||
“Nutrition” (Pillar I) | 315 | |
“Safety” (Pillar II) | 362 | |
“Authenticity” (Pillar II) | 12 | |
“Metrology” | 0 |
When the number of publications is plotted against years, the results reveal how scientific interest in edible insects has increased significantly since 2014 and the publication of the FAO’s paper on Edible Insects Future Prospects for Food and Feed Security by van Huis et al. [1] (Figure 2). The retrieved articles were further probed using targeted keywords tailored to each pillar theme to identify significant knowledge gaps, and then they were systematically scrutinised for inclusion in the review. Regarding the different thematic pillars, safety, followed closely by nutrition, produced similar hits. The search term “authenticity” produced a significantly smaller number of hits, while the term “metrology” produced no hits (Table 1). The full results are presented in the supplementary (Table S1).
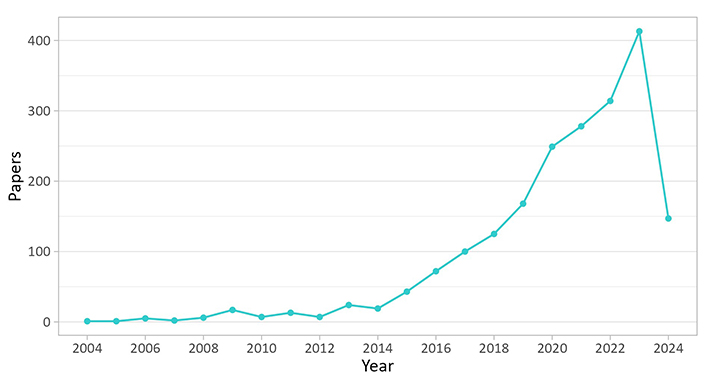
Plot of number of papers vs. publication year (Search fields: article title, abstract, keywords: “edible insects”, data: Scopus)
Pillar I: enhancing the nutritional quality of insect biomass
As mentioned, as part of a more sustainable European protein strategy, farmed insect protein content is a viable option for becoming more self-sufficient in protein production for feed and human consumption. Pillar I aims to achieve two objectives: enhancing the nutritional and functional value of insect biomass and adapting insect feed composition to include agri-byproducts, thereby enhancing their nutritional quality. For instance, the INPROFF project explores how fermentation can boost nutritional values, sensorial properties, and safety/shelf life. It also looks at ways to modify insect feed by adding agricultural by-products to make insects more economically viable and competitive with conventional protein sources, such as soybeans.
The literature review reveals that farmed insects are a rich source of easily digestible quality protein [≈ 40–75 g/kg of dry matter (DM)] equivalent to meat [17, 18]. They can also provide most of the essential amino acids, including lysine, leucine, phenylalanine and tryptophan, fat and lipids (approx. 20–55 g/100 g) [19, 20] along with several essential fatty acids with total polyunsaturated fatty acids (PUFA) accounting for 3–50% [20]. They also contain fibre (8.5–27 g/100 g) and carbohydrates, mostly chitin (2.7 mg and 49.8 mg per kg of fresh weight), as well as minerals (Ca, K, P, Na, Mg, Cu, Fe, Zn, and Mn) and vitamins A, D, E, K, and C and B groups vitamins [20]. Insects also represent an excellent sustainable source of chitin (5–15% w/w) [21]. The latter values were obtained using a reliable electrophoresis assay developed within INPORFF, which overcomes the inherent problem of being unable to distinguish between chitin and cellulose.
Bioactive compounds like antioxidant enzymes and peptides are also present in insects [22], some of which have therapeutic potential [23]. For instance, cantharidin from blister beetles can induce apoptosis in cancer cells by affecting nucleic acid and protein metabolism in hepatocellular carcinoma cells. Cecropin peptides found in some insects have shown cytotoxic effects against leukaemia and lymphoma cells by forming pores in their plasma membranes [24]. Traditional Chinese medicine frequently utilises insect extracts to treat various ailments, including cancer [23]. Not all bioactive compounds are necessarily beneficial. Insects belong to foods with high levels of total purine (3.23–13.22 g/kg DM) and uricogenic purines (adenine and hypoxanthine: (1.55–8.86 g/kg edible DM), which contain amounts analogous to meat (3.61–5.53 g/kg meat DM) and are a problem for those who suffer from gout and kidney stones [25]. From this perspective, the study’s authors conclude that the study insects make unsuitable alternatives for low-purine diets.
Although polyphenols are commonly associated with plants, insects also contain polyphenolic compounds, which act as antioxidants with anti-inflammatory, anti-cancer, antityrosinase, antigenotoxic, and pancreatic lipase inhibitory activity [26]. Insects also possess polyphenols serving as pigments and chemical defences, and they can synthesise and store non-dietary phenolic compounds through sclerotisation [27]. They also have the capability to selectively absorb and accumulate flavonoids in their bodies from the larval stage via their host plant, so their diet is important in determining the type and amount of polyphenols present [26]. Research into the content and specific bioactive properties of insect-derived polyphenols remains ongoing.
Nutritionally, the case for insects is strong; however, more rigorous analyses are needed, including assessments of insect nutrients not thoroughly investigated (particularly vitamins), the presence of non-nutrients and anti-nutrients and how they influence the digestibility and bioavailability of nutrients in various species and processed products. A lack of such studies also means there is currently a limited understanding of how insect-based foods affect the human and animal gut microbiome [28]. Imbalances in the human gut microbiome are linked to metabolic and non-communicable diseases, gastrointestinal conditions, allergies, asthma, and even neuropsychiatric disorders [29]. An even more significant gap exists (search hits = 8) in our understanding of the insect gut microbiome.
Insects are also promoted as a more sustainable option than conventional non-plant protein sources [30–32]. For example, the rearing of insects through vertical farming, which, unlike locusts, is particularly suited to the mealworm, requires far less land (3.6 m2/kg of fresh insects to 94.7 m2/kg of dry insects), significantly less water (0.42–0.82 m3 of water depleted per 1 kg of fresh insects); have highly efficient feed conversion rates (50–70%); produce less waste and amenable to further processing and rearing in modern production systems [33–35]. The result is lower greenhouse gas emissions (2.3–3.1 kg CO2 eq per kg of fresh insects) than other non-plant protein sources [36, 37]. Insects, those in larvae form, can also serve as aqua feed alternatives, replacing traditional non-sustainable options like fish meal [3].
The nutritional content of insects varies based on numerous factors, which means that it can be improved using specific measures in farming technology to improve the efficiency of the animal’s diet [38]. For insects to compete economically with other sources of animal feed, it will be necessary to rear them on by-products and waste from the food industry and agriculture, i.e., transforming low-value organic by-products into high-value food or feed [32, 39, 40], thereby reducing costs and increasing the economic viability of insect-based feed [41]. However, this will require the development of nutritionally balanced insect diets. Similarly, insects can also be a source (bio-factories) of other valuable secondary commodities for pharmaceuticals, biofuels and lubricants [42, 43], and their waste (frass) can be used as fertiliser [44, 45].
Farming edible insects allows an opportunity to manipulate the nutrient content of insects via their feed [46, 47]. For example, the transfer of beneficial substances such as carotenoids or omega-3 fatty acids (n-3) from feed to insects has been observed [38, 48]. These fatty acids, omega 3 (n‐3) and omega 6 (n‐6), are required in mammalian diets because mammals cannot synthesise these de novo. Although essential for health, Western diets contain too little n‐3 and too much n‐6 PUFA. Increasing the beneficial fatty acids amounts of n‐3 PUFA, which would result in more favourable n‐6/n‐3 ratios, would benefit animal feed and humans since it can reduce coronary heart disease, cancer, autoimmune and inflammatory diseases [49]. For example, Škvorová et al. [47] have shown that rapeseed cake can substitute soybean meal in feeding the field cricket, resulting in higher crude protein levels and lower amounts of palmitic acid.
Fermentation is an effective method for enhancing the nutritional properties of certain foods and is being investigated to extend their shelf life [50, 51], microbiological safety and sensory characteristics [50, 52, 53]. It is also being used as a way of increasing the efficiency of their original bioactive compounds (functional value) via their release or transformation by lactic acid bacteria (LAB) as well as enriching the substrate in their metabolites, e.g., polysaccharides, bioactive peptides, vitamin B12, short-chain fatty acids [54, 55]. These transformations are associated with improving the bioaccessibility and bioavailability of food components and their health-related properties [55].
Moreover, fermentation can also increase the digestibility and stability of waste before being utilised as insect feed by converting food and by-product wastes into ingredients for artificial insect diets [51, 56]. Companilactobacillus appears most promising, resulting in a significant increase in free glutamic and aspartic acid. These compounds are responsible for the umami flavour of fermented foods and might stimulate consumer acceptance of insects and their consumption [57]. Hadj Saadoun et al. [58] applied lactic acid fermentation of waste to the puparia and dead adults of black soldier flies. The authors showed that the fermented puparium has a high antimicrobial activity against three pathogenic strains: Salmonella enterica, Listeria monocytogenes, and Escherichia coli. The authors also reported a shift in lipid composition from that typical of insects to one containing fatty acids, while the LAB strains could modify the biomass’s protein composition depending on the specific LAB strain and the substrate.
Pillar II: safety and quality
Insect products regulated as novel foods or as feed are considered safe, having undergone an extensive risk assessment process, and, in the EU, where insects are allowed on the market, have to adhere to strict EU regulations [59, 60]. For example, [Regulation (EU) 2023/915] establishes maximum permitted levels in food for the specific contaminants [e.g., toxic elements (cadmium, lead, mercury, inorganic tin, arsenic), plant toxins (erucic acid)], mycotoxins (e.g., aflatoxins, ochratoxin A, fumonisins, and citrinin), processing contaminants [e.g., 3-MCPD, dioxins, dioxin-like polychlorinated biphenyls (PCBs) and nondioxin-like PCBs, polycyclic aromatic hydrocarbons (PAH), and polyfluorinated substances (PFAS)], and others (e.g., melamine and nitrate) [61]. However, despite the work on looking at the safety of insects [32, 46, 62–70], like with any new food or feed, there will be known and unknown so-called emerging risks [70]. A key part of the INPROFF project involves characterising less well-studied contaminants’ chemical and biological safety using state-of-the-art instrumentation. This task involves performing target analysis and developing nontarget screening workflows, which have yet to be fully utilised in the literature. The project also investigates the effect of specific bioactive substances and their cytotoxicity using in vitro gastrointestinal cell cultures.
Farmed insects, fed on cereal-based feeds, could be exposed to toxic elements, pesticide residues, mycotoxins and other biologically produced toxins and biohazards (bacteria, viruses, fungi, parasites), food chemicals and additives of natural or non-natural origin, many of which have the potential to bioaccumulate and physical foreign matter for example from the degradation of production equipment [66, 68, 71, 72]. Modern intensive farming production methods, even for minilivestock (insects), offer many routes for potential sources of contamination across the entire food supply chain, particularly given the many unknowns associated with scaling up insect production systems [70, 73, 74]. For insects destined for feed (e.g., black soldier fly), the risk of exposure will be amplified if, in the future, insects are reared on agri-byproducts, mixed wastes from processing plants, supermarkets and even restaurants [75].
Chemical contamination, especially the biotransfer, bioaccumulation, and biotransformation of contaminants from feed to food [76] and how their uptake is affected by species, life stages, and rearing environment [77] is also underexplored. The same is true concerning the effect that processing methods have on the presence of chemical hazards, even though there is some evidence that processing methods like boiling, frying and roasting increase the safety of edible insects [71]. However, conventional cooking conditions will most likely not be an effective way to eliminate contaminants such as per- and polyfluorinated substances (PFAS), so-called “forever chemicals” [78, 79] with a high potential to bioaccumulate [80]. Other potential risks addressed in food/feed but not yet studied concerning insect rearing include, for example, the uptake of micro and nano plastics from feed [81, 82], which could by themselves be problematic or act as carriers for other contaminants [83, 84]. Reducing such risks will require greater control over their feed and environment and the development of high throughput put and methods capable of detecting a broader range of compounds.
Among the now large number of publications, the majority are target analyses of a few compounds or multi-residue analyses [68, 85]. Targeted methods focus on detecting and quantifying pre-defined compounds or analytes in a sample, which can miss other potentially hazardous groups of contaminants present in the samples. In response, many scientists have turned to suspect and nontarget screening methods to define the chemical characterisation of particular samples [74, 80, 86].
The sheer number of potential organic chemical contaminants and their mixtures, which are constantly changing, also raises problems in assessing risk, and focusing on only a few specific target compounds could mean other hazards go undetected. For this reason, attention is turning towards exploring alternative analytical workflows, such as suspect and non-targeted ones, as part of an exposome concept [87]. Suspect screening involves searching for compounds on a pre-defined list of “suspect” compounds or compound classes, often based on their chemical properties or structural features [88, 89]. Such approaches offer a more comprehensive assessment by detecting unexpected contaminants or adulterants, enhancing the detection of emerging risks that may not be accounted for in targeted analyses, i.e., non-targeted analysis aims to screen and identify compounds present in a sample without prior knowledge. This ability to perform such analysis is partly due to the introduction of Time-of-Flight (ToF) and Orbitrap-mass spectrometry (MS) high-resolution benchtop instruments, which have the advantage of using full-scan acquisition mode with high sensitivity, high resolving power and accurate mass measurement, allowing the rapid characterisation of potentially thousands of compounds [88, 90, 91].
Ideally, all three approaches—targeted, suspect, and nontarget—are sequentially performed on the same set of samples. However, few insect-related studies have been published using the described approaches [74, 80, 86]. For instance, Poma et al. [74] performed targeted screening of organic contaminants [flame retardants, PCBs, dichlorodiphenyltrichloroethane (DDT), dioxin compounds and selected pesticides] and additional suspect pesticide screening of edible insects and insect-based food intended for human consumption. The organic chemical mass fractions of targeted compounds were typically lower than in common animal products. In contrast, the untargeted screening analysis revealed the presence of vinyl toluene, tributylphosphate (present in 75% of the samples), and pirimiphos-methyl (identified in 50% of the samples)—frequently identified contaminants that would have been missed with a targeted approach. Li and Bischel [80] also performed target and suspect screening to evaluate the bioaccumulation of trace organic contaminants in black soldier fly insect larvae fed on almond hulls, a by-product from the almond industry. Their study showed that the four targeted per- and polyfluoroalkyl substances (PFAS), perfluorobutanoic acid (PFBA), perfluorooctanoic acid (PFOA), perfluorobutanesulfonic acid (PFBS) and perfluorooctanesulfonic acid (PFOS), did not bioaccumulate in the larvae. However, employing a nontarget approach uncovered the presence of other PFAS compounds, specifically hydrogen-substituted polyfluoroalkyl carboxylic acids (H-PFCA), which were found to bioaccumulate in the larvae and were correlated with their presence in the feed (almond husks).
Similarly, Macan Schönleben et al. [86] performed target and suspect pesticide screening of 52 edible insects from Asia, Africa, and Europe. Fifty-six pesticides were determined using target (30) and suspect (26) screening approaches. Notably, in the case of targeted screening, the neonicotinoid imidacloprid had the highest detection frequency (DF, 70%), followed by fipronil (48%). The findings indicated that the levels of dichlorvos and fipronil were in the same order of magnitude as specific maximum residue limit (MRL) values for meat. Most detected compounds were below the defined MRLs. However, it underscores the necessity for further extensive investigations to provide a comprehensive chemical characterisation of potential risks relating to edible insects as well as the need to establish (MRL) values for edible insects. These limited studies that do not focus entirely on target analysis show the importance of suspect screening for providing valuable insights into food/feed chemical characterisation and facilitating a more proactive and holistic approach to ensuring the safety of farmed insects. However, the full potential of applying a nontarget approach is still to be explored.
Biological risks must also be considered when contemplating the use of insects. The microbiota of insects, found in the gut and other anatomical parts, is complex. While the microbiota of some commercially essential insects has been analysed [50, 92–95], more work in this area remains to be done. This task is essential, as insects tend to be consumed entirely, with a few exceptions. Although most pathogenic microbes that infect insects are harmless to humans due to phylogenetic differences, insects can be vectors or carriers of harmful microbes, primarily when insects are reared under poorly controlled hygienic conditions [96]. Among the most harmful bacteria that inhabit all three insect species analysed within the INPROFF project (yellow mealworm, house cricket, and migratory locust), Salmonella, Staphylococcus aureus, Clostridium perfringens, and Bacillus cereus could be mentioned. Several bacterial species have been associated with edible insects, both farm-reared and wild-caught, and some of these are not only pathogenic and opportunistic bacteria, but they can also reduce the shelf-life of edible insects [97].
Endospore-forming bacteria in edible insects are also a significant food safety concern as the heat-resistant spores may withstand the standard processing methods adopted for edible insects, like drying, boiling, and deep-frying [98]. In this context, the Bacillus cereus group seems to be one of the most suitable parameters for describing food safety and microbial quality [99]. So far, risks associated with foodborne viruses, like hepatitis A, hepatitis E and norovirus, from consuming edible insects are low. However, care must be taken not to introduce the viruses in insect production units through their feed and unsafe handling practices. Insect species deemed fit for mass production may be vectors for parasites, although this risk may not be exceptionally high and is poorly documented [100, 101]. Contamination of edible insects post-processing is also an area of consideration [102].
The study of the pathogenicity of insect-specific viruses in humans is also an area for further study. Insect viruses are assumed to be specific to insect species and cannot replicate in vertebrate cells. Despite this limited specificity, there is a negligible risk of new strains of mammalian-specific viruses emerging due to recombination and rearrangement of genetic material, potentially leading to host switching. Although pathogenic viruses of vertebrates cannot replicate in insects, edible insects can still passively transmit these pathogens [63, 103]. Research into extending the shelf-life of processed or farmed insect-derived foods and feeds and microbial studies on farmed edible insects is still in its early stages [104]. Similarly, there is a gap in our understanding of other microbial risks, e.g., protists [105]. Most microbes in or on insects are not considered harmful to human consumers. However, the microbial safety of insect-based foods intended for human consumption is still under debate, as insects could be vectors of potentially pathogenic bacteria.
Pillar III: authenticity, traceability, and consumer acceptance
The European Commission estimates that the total cost of food fraud globally is about 40 billion Euros annually [106]. Without the necessary tools, a nascent insect farming industry is ripe for economic fraud, such as product adulteration and mislabelling. The mapping of the insect supply chain in the study performed by Traynor et al. [70] illustrates the complexity that exists. This situation is worsened by the lack of standard procedures for determining the authenticity of insect products [107]. The literature review reveals a significant literature gap when searching “edible insects” and “authenticity” (hits = 12), with few studies investigating the potential fraud in edible insect food supply chains. INPROFF was partly set up to fill this gap by taking advantage of the IJS food authenticity and traceability expertise and applying it to farmed insect products. This task is made possible due to significant progress in developing accurate tools for determining the species-level authenticity of food and detecting adulterants [108–111].
One such tool involves the use of the stable isotope approach. Stable isotopes of light elements (expressed in δ2H, δ13C, δ15N, δ18O, and δ34S) have been widely used to investigate food authenticity and origin [112], although for farmed edible insects for food and feed its use is limited to a few examples and is more widespread in insect ecology [113]. Nevertheless, the information obtained in ecological studies contributes to the knowledge needed to determine the authenticity of insects for food and feed. For example, the δ13C values are strongly influenced by diet, providing insights about whether the insects fed on C3 or C4 plants [114]. The δ34S values are also related to the food insects consume. The values are influenced by geology, microbial processes in the soil (including aerobic and anaerobic growing conditions), the distance from the sea (“sea spray effect”) and fertilisation techniques [115, 116]. The δ15N values of the animals are strongly related to their trophic level [117]. Herbivorous species are expected to have a lower δ15N than carnivorous ones, as 15N accumulates along the trophic chain [112]. The δ2H and δ18O of water are strictly related parameters [118] that depend on latitude, altitude, and even distance from the sea [119]. For plants, the correlation between δ2H and δ18O is still valid but not that strict, as the only source of hydrogen is the water absorbed through the stomata [120]. As the sources of oxygen and hydrogen of the insects are both the water they drink and the plants they eat, their δ2H and δ18O are influenced by such factors.
Pianezze et al. [121] analysed 40 samples of edible insects and four insect-based food items, providing δ13C, δ15N, δ34S, δ18O, and δ2H data. Due to ingredient and flavour additions, they found δ2H and δ18O helpful in differentiating natural from seasoned insects. Other parameters, like δ13C, δ15N and δ34S, showed promise in characterising insect diet, geographical origin, and trophic level. Macan Schönleben et al. [86] studied 52 edible insect species from Asia, Africa, and Europe, focusing on δ13C, δ15N, δ34S, and δ2H for food authenticity and traceability. Linear discriminative analysis differentiated geographical areas, with African samples showing distinct profiles, and accurately classified pesticide presence based on δ34S values at a 76% rate.
INPROFF is developing a crucial component in food authenticity and traceability: establishing a reference dataset based on genuine samples, as highlighted by Donarski et al. [122]. This dataset will serve as a benchmark against which insect-based products in the market can be compared. Moreover, many aspects must be considered when developing such a database, including insect species, diet, environment, developmental stage, and processing techniques.
A study by Fuso et al. [123] further evaluates the analytical tools currently available to authenticate insects and their rearing substrate. The study emphasises the need for specialised analytical methods to detect rearing substrate residues in insect products or to reveal metabolomic changes caused by different feeds. A novel method for authenticating edible insect powders was recently introduced by Tata et al. [124] based on obtaining direct analysis in real-time high-resolution MS (DART-HRMS) signatures, marking it the first application of ambient MS to screen novel foods. The study successfully differentiated between four test species (house cricket, yellow mealworm, migratory locust and silkworm) with a cross-validation accuracy of 100% for all training samples. A distinct metabolic fingerprint was observed for house cricket in particular. Additionally, Poma et al. [125] explored the potential of entometabolomics in food systems, marking a crucial step towards understanding edible insects’ metabolic profiles that can be used to identify the authenticity of insect food.
Consumer acceptance
For humans, the eating of insects, termed “entomophagy”, is not new and many species are already consumed by much of the world’s population. The literature commonly quotes two billion plus people who regularly consume insects and suggests that insects could become a regular part of the Western diet [64]. However, entomophagy is not a cultural norm in Europe, and convincing those not accustomed to eating insects remains a significant hurdle to their acceptance. However, research is dedicated to making insects more palatable [126]. Today, insect-based products such as pasta, snacks, and protein bars are available on the European market, projected to be two billion euros/year by 2030 [127].
Food scientists have identified various factors influencing consumer acceptance of insect consumption, among which food neophobia and disgust remain the primary barriers to eating insects [128–130]. However, familiarity, tradition, and sensory attributes also affect the willingness to consume insects across different geographical locations and cultures [131]. While Western countries emphasise nutritional value and sustainability, sensory qualities and cultural practices play a more substantial role in regions where entomophagy has a longstanding tradition [132, 133].
The availability of information and prior experience with edible insects can positively shape consumer attitudes [134–136]. In Western societies, incorporating insects into familiar food products and communicating their environmental and nutritional benefits can enhance their acceptance [126, 131]. Additionally, focusing on product development that aligns with the sensory expectations of consumers [137, 138] and sparks curiosity about edible insects [139, 140] can lower the initial resistance towards them. Within the INPROFF project, a study performed by Kulma et al. [141] found that in the case of the Czech Republic, a significant percentage of those taking part described their entomology experience as positive (43.8%), 11.8% stated they regularly consumed insects, a small fraction (6.7%) reported negative experiences, and 37.7% remained neutral. Also, the data showed consumer preference leaned towards insect powder and processed whole insects such as crickets, katydids, and locusts, with cockroaches being the least favoured.
Metrological approach and challenges
All three pillars of the INPROFF project rely on analytical methods, and ensuring robustness between and comparability of the data obtained requires using appropriate metrological tools. Metrology is critical in ensuring that food products meet safety standards and regulatory requirements across the food supply chain, which can only be achieved through accurate, consistent, and traceable measurements. Typically, these include quality reference standards, validated standardised methods, standardised sampling practices, proven calibration methods and, where possible, matrix-specific certified reference materials (CRMs) in addition to reference materials (RMs), and interlaboratory comparisons are used to achieve comparability of analytical results [142]. Despite its importance in food research, this literature review found no specific studies (zero hits) applying metrology to farmed insects or significant discussion regarding its importance to the field.
For food products, including edible insect products, metrology is the foundation for supporting accurate labelling of food products, including nutritional information, ingredient lists, allergens, and expiration dates [143]. As the determinants of general nutritive quality (Pillar I), proximates such as lipids, carbohydrates, proteins, and minerals must be considered. Here, the standards are general reference methods collected under the International Organization for Standardization (ISO) system [144]. Moreover, within lipids and proteins, the content of essential components, such as essential amino acids and essential fatty acids, are determined by amino acid analysis and multidimensional gas chromatography [145]. In the case of trace nutrients such as elements and vitamins, the methodology is much less standardised and under ongoing development [143].
Analytical methods for many known organic contaminants (Pillar II) in food have undergone extensive validation and standardisation [146]. Among the “new age” pollutants, pesticides are probably the most studied and multi-trace residue analysis methods are preferred over single-residue methods [147]. However, as already discussed earlier under Pillar II, the range of these pollutants is not complete and is expanding as the knowledge of the risks of consuming novel foods such as insects increases. Also, contaminants can transform in, for example, animal-derived food and predicting the structure and toxicity of their transformation products is a developing trend [148].
In trace analysis, quantitation is also more susceptible to analytical errors and statistical imprecision and demand methods with increased sensitivity and specificity, which, especially for the latter, is effectively achieved through chromatography (most commonly liquid) hyphenated to MS. However, although liquid chromatography-MS (LC-MS) is a highly specific and sensitive analytical technique, matrix effects are often caused by the alteration of ionisation efficiency of target analytes in the presence of co-eluting compounds in the same matrix and often impede signal intensity and even mass accuracy. Accordingly, the evaluation of matrix effects is explicitly suggested by regulative bodies like the United States Food and Drug Administration (US FDA) guidelines on bioanalytical analysis [149].
One of the most common approaches to account for uncertainty issues in target analysis is using an internal standard (IS). These are typically suitable surrogate compounds with properties similar to the target analyte, such as chemical structure, solubility, and volatility. In MS, they are ideally isotopically labelled chemicals (13C, 15N, or 2H) since they are absent in samples but have the same characteristics. In MS-based target analysis of organic micropollutants, the addition of IS is used to compensate for losses of physicochemically similar compounds when internal calibration approaches are used. When targeting more extensive groups of contaminants, the set of IS should ideally cover the whole range of the chemical space to be explored (retention time, mass range, ionisation mode, and physicochemical properties). However, besides being impossible to account for all substances potentially present in the sample, only a limited number of compounds are commercially available in isotope-labelled form, and high price also limits their application [150].
The trend towards non-targeted high-resolution MS (HRMS) also raises metrological issues. Regarding nontarget analysis and suspect screening, methods focus on compound identification rather than quantitation. One of the most important scopes of NTA is that it can directly support chemical risk characterisation but must provide quantitative estimates. Even though limited quantitative NTA methods exist, they do not fully consider estimation uncertainty, and most nontarget approaches still need harmonisation and validation [151, 152]. Equally, in the case of new threats such as from nanoparticles and micro-/nanoplastics, as also discussed in Pillar II, their detection and quantification are limited by the complexity of the food matrix absence of suitable standards, making their identification and quantification challenging and represent major bottlenecks [153].
From a microbial risk perspective, appropriate ISO norms and standardised procedures exist to determine biological safety for classical food hygiene parameters [154]. For example, total aerobic and anaerobic bacterial counts, counts of Staphylococcus aureus, E. coli and Enterobacteriaceae, Clostridium perfringens, spore-forming bacteria, enterococci, and the presence of Salmonella were determined. Bacillus cereus (a microbial quality indicator) was detected according to ČSN EN ISO 7932 [155], identified by 16S rDNA sequencing, and its pathogenic potential was studied by detecting virulence-associated genes.
Another challenge is maintaining consistency and reproducibility in constructing microbial communities, especially in highly diverse microbial communities as in the case of fermentation [156, 157] or studying the effects of consuming insects on human gut microbiome [28] human and feed modification on the insect microbiome [41, 158] or for biotechnological applications [159]. Another trend that calls for the establishment of metrological tools is highlighted by Mattarozzi et al. [160], namely, the rapid development of point-of-need devices and low-cost portable testing, frequently intended for use by non-experts. While this trend benefits the food industry enormously (e.g., faster results, on-site testing, improved food safety, cost reductions, enhanced traceability, and empowering non-experts), it leaves many metrological questions open regarding accuracy and reliability, standardisation, calibration, validation, and regulatory acceptance [160].
Metrology is particularly important for Pillar III since accurate measurements are essential to support the certification systems that ensure authenticity, prevent misleading claims, and prevent adulteration. Semiautomated and fast stable isotope-ratio analysis (SIRA) in continuous-flow mode with an elemental analyser (EA) interfaced with isotope-ratio MS (IRMS) has become a routine and invaluable tool for food authenticity and traceability (Pillar III) [161–163]. Several standardised analytical techniques have been officially recognised as well-established, validated and acknowledged by the Association of Official Agricultural Chemists (AOAC), the European Committee for Standardization (CEN), or EU methods [162]. Importantly, accurate measurements of relative stable isotope ratios of hydrogen (expressed as δ2H), carbon (δ13C), nitrogen (δ15N), oxygen (δ18O), and sulfur (δ34S) require normalisation using at least two isotopic RMs with contrasting isotopic compositions to (i) anchor δ-values to conventional isotope—delta scales and (ii) compensate for differences in responses of analytical instrumentation, which commonly compress isotope—delta scales [164–166]. The new guidelines for reporting HCNOS isotopic delta values with JSI participation were recently published [167]. While authenticity identification usually works successfully with one or two stable isotope parameters, geographical origin determination often requires multiple stable isotope parameters and even the inclusion of elemental composition or heavier isotopes such as strontium. Other metrological tools include participation in interlaboratory [168] and the Consultative Committee for Metrology in Chemistry and Biology (CCQM) organised assessment [169] and development of new RMs of plant and animal origin [170].
From a metrological perspective and across all three thematic pillars, there is a notable deficiency in suitable RMs and interlaboratory trials, including collaborative trials, which aim to verify the performance of an analytical method, and proficiency testing, which focuses on assessing participants’ performance [146]. RMs (and CRMs) represent one of the main metrological tools supporting reliable measurements regarding calibration, method validation and quality control, guaranteeing metrological traceability, method validation and quality control [146]. Ideally, RMs must be chemically as similar as possible to the sample to account for matrix effects, i.e., based on the studied insects.
While there has been an increase in the production of new RMs in recent years, there remains a shortage of RMs tailored explicitly for the agrifood sector despite the persistent demand to create new RMs with varied matrix/analyte combinations to meet evolving analytical needs. This necessity arises from several factors, including advancements in analytical techniques, the emergence of new parameters of interest (e.g., nanoparticles and micro/nanoplastics), the evolution of methods for food profiling for quality, authenticity, and traceability purposes, and the imperative to study emerging contaminants as discussed in this review highlights the emerging needs for new RMs. Addressing these methodological gaps presents a significant opportunity for future research [143, 146].
Conclusions
In this review, we addressed the current progress and challenges relating to farmed insects as food and feed, identified through the lens of the INPROFF project’s core themes: nutrition enhancement, quality and safety, and authenticity. Three things quickly become apparent. The first is that interest in farming insects in a European context continues to grow; second, that much research has been and is being done, resulting in a significant body of research; and third, but just as important, there remains much to be learnt, and farming insects creates unique issues. The argument for accepting insects as alternative proteins for feed is economical, while as food, the issues remain regarding their negative perception. Both could be resolved, for example, in the former case by substitution and fortification (valorisation) of feed and the latter by incorporating insects as ingredients and developing new foods with increased functionality as added value or by improving their sensorial qualities thereby sidestepping any potential neophobia and possibly by also taking a more heuristic approach to increase acceptance.
The safety of farmed insects still poses nutritional, chemical, and biological challenges and gaps in our understanding to be filled, something that will become more significant if insects are reared on by-products of agriculture and waste streams from food processing. More work is needed to understand the transfer of contaminants from raw materials during production and processing, including exogenous and endogenous nonbiological and biological risks. The large number of potential contaminants also drives the development of nontarget analytical workflows based on high-resolution mass spectrometry methods. Also important is addressing the gaps in metrological challenges of analysing insects (and novel foods in general), which will be critical for ensuring safety, quality, and sustainability as they continue to gain prominence in the food industry. Finally, authenticity and adulteration are only now being addressed. Fortunately, many authenticating tools developed for other food groups based on chemical, isotopic and genetic methods can be adapted to farmed insects.
Abbreviations
AOAC: | Association of Official Agricultural Chemists |
ARIS: | Slovenian Research Agency |
CCQM: | Consultative Committee for Metrology in Chemistry and Biology |
CEN: | European Committee for Standardization |
CRMs: | certified reference materials |
CZU: | Czech University of Life Sciences Prague |
DART-HRMS: | direct analysis in real-time high-resolution mass spectrometry |
DDT: | dichlorodiphenyltrichloroethane |
DM: | dry matter |
EA: | elemental analyser |
EC: | European Commission |
EFSA: | European Food Safety Authority |
EU: | European Union |
FAO: | Food and Agriculture Organisation |
GAČR: | The Czech Science Foundation |
H-PFCA: | hydrogen-substituted polyfluoroalkyl carboxylic acids |
HRMS: | high-resolution mass spectrometry |
INPROFF: | Quality, Safety and Authenticity of Insect Protein-Based Food and Feed |
IPIFF: | International Platform of Insects for Food and Feed |
IRMS: | isotope-ratio mass spectrometry |
IS: | internal standard |
ISO: | International Organization for Standardization |
JSI: | Jožef Stefan Institute |
LAB: | lactic acid bacteria |
LC-MS: | liquid chromatography-mass spectrometry |
MRL: | maximum residue limit |
MS: | mass spectrometry |
PAH: | polycyclic aromatic hydrocarbons |
PAP: | processed animal protein |
PCBs: | polychlorinated biphenyls |
PFAS: | polyfluorinated substances |
PFBA: | perfluorobutanoic acid |
PFBS: | perfluorobutanesulfonic acid |
PFOA: | perfluorooctanoic acid |
PFOS: | perfluorooctanesulfonic acid |
PUFA: | polyunsaturated fatty acids |
RM: | reference materials |
SIRA: | stable isotope-ratio analysis |
ToF: | Time-of-Flight |
TSEs: | transmissible spongiform encephalopathies |
US FDA: | United States Food and Drug Administration |
Supplementary materials
The supplementary material for this article is available at: https://www.explorationpub.com/uploads/Article/file/101041_sup_1.pdf.
Declarations
Author contributions
DH: Conceptualisation, Investigation, Writing—original draft, Writing—review & editing. NO: Conceptualisation, Investigation, Writing—original draft, Writing—review & editing. All other authors, LK, MK, PŠ, AV, HŠS, BL, NR, PJ, BJ, MČ, NPU, and EH were responsible for writing and editing. All authors have reviewed and approved the submitted manuscript.
Conflicts of interest
Nives Ogrinc who is a guest editor for the special issue “Metrological Aspects in the Analysis of Nutrients, Functional Compounds, Additives and Contaminants in Food and Feed” of Exploration of Foods and Foodomics had no involvement in the decision-making or the review process of this manuscript. The other authors declare that they have no conflicts of interest.
Ethical approval
Not applicable.
Consent to participate
Not applicable.
Consent to publication
Not applicable.
Availability of data and materials
Not applicable.
Funding
The authors kindly acknowledge the financial support from the Slovene Research and Innovation Agency (ARIS, program group P1-0143 and project number [J7-3155]); the Czech Science Foundation (GAČR, project number [21-47159L]); and the FoodTraNet Project funded by the EU Horizon 2020 research and innovation programme under the Marie Sklodowska-Curie grant agreement no. [956265]. The funders had no role in study design, data collection and analysis, decision to publish, or preparation of the manuscript.
Copyright
© The Author(s) 2024.