Abstract
Pickering emulsions have emerged as suitable alternatives to healthily and sustainably deliver unstable compounds, addressing the demands of consumers, increasingly concerned about the nutritional value and environmental impact of the products they consume. They are stabilized by insoluble solid particles that partially hydrate both the oil (O) and aqueous (W) phases through a combination of steric and electrostatic repulsions determined by their surface properties. Since the desorption energy of the particles is very high, their adsorption is considered irreversible, which accounts for their greater stability compared to conventional emulsions. Proteins and polysaccharides, used either individually or in combination, can stabilize Pickering emulsions, and recent studies have revealed that microorganisms are also suitable stabilizing particles. This review provides an overview of recent research on Pickering emulsions, highlighting the properties of the stabilizing particles, and their ability to deliver hydrophobic and/or unstable compounds. The use of Pickering emulsions as fat-replacers, edible inks for 3D-printing or their incorporation into packaging material are also presented and discussed, pointing out their great potential for further innovation.
Keywords
Surface properties, interface adsorption, food grade emulsions, delivery systems, 3D-printing edible ink, fat replacersIntroduction
Emulsions are commonly used in the food industry for the manufacture of different products (e.g., beverages, milk, smoothies, creams, sauces, desserts, and dressings, among others), imparting attributes such as texture or flavor among others. They have also been extensively employed as delivery and release systems for bioactive compounds, including vitamins or antioxidants, as well as lactic acid bacteria [1–4].
From a physicochemical point of view, emulsions are thermodynamically unstable systems, consisting of two immiscible phases [usually oil (O) and water (W)], requiring surfactants to reduce the interfacial tension and stabilize them. Several surfactants employed in food emulsions are chemically synthesized, but they may have a negative environmental impact and potential adverse effect on consumers’ health (e.g., alterations in intestinal microbiota, allergies) [5, 6], who in turn, are increasingly concerned about the nutritional value of the products they consume. This has led to the current trend of reducing their use, challenging technologists to formulate stable emulsions without them. Pickering emulsions emerge as suitable solutions to overcome this problem, as they can be stabilized by insoluble solid particles adsorbed to the interface [7]. These particles can partially hydrate both the O and W phases through a combination of steric and electrostatic repulsions, determined by their surface properties (e.g., hydrophobicity, contact angle, and superficial tension). Besides the fact of being free of surfactants, sustainable, and physico-chemically stable [8, 9], it is important to point out that their stability also prevents degradation during exposure to gastro-intestinal conditions. This additional advantage improves the bioaccessibility of the compounds encapsulated in them [10].
Different types of particles have been used, namely inorganic safe compounds (e.g., silica, calcium carbonate, hydroxyapatite, clay, magnetic nanoparticles intended for biomedical applications), chitosan, cyclodextrins, or carbon nanotubes [11]. Natural stabilizers can be also employed, and they have become the most appropriate choice to stabilize Pickering emulsions intended for food applications. Among this second group of stabilizing particles, one can count on proteins (e.g., whey, zein, soy, pea), carbohydrates (e.g., starch, chitosan, cellulose), or safe microorganisms [11].
The diverse nature of the stabilizing particles opens up the possibility of generating innovative technological applications, suitable to be incorporated into food and nutraceutical products. The literature addressing these applications is very recent, as articles date back mostly from the last 4–5 years. Besides that, several of those articles discuss the formulation and physico-chemical properties of “empty” emulsions, focusing on the role of natural particles as Pickering stabilizers, without exploring the emulsions’ suitability for encapsulating unstable compounds. This also highlights the novelty of the topic, underscoring the potential for further innovation. Hydrophobic compounds with food relevance are suitable to be encapsulated into Pickering emulsions and this way, extend their shelf-life and their stability when exposed to gastro-intestinal conditions. Although the attention to these issues has increased in recent years, only a few unstable compounds have been encapsulated (e.g., β-carotene, curcumin, astaxanthin, lycopene, certain essential oils, hydrophobic vitamins), which underlines the significant and yet unexplored potential of Pickering emulsions as delivery systems. Indeed, the great potential of Pickering emulsions goes beyond the encapsulation of unstable compounds. They can be incorporated into the formulation of functional foods, sustainable and antimicrobial packaging materials, and even used as fat replacers and edible inks for 3D-printing food products.
Unlike previous reviews that primarily focused on the formulation and physico-chemical properties of “empty” emulsions using natural particles as stabilizers, this manuscript delves into the practical applications and benefits of encapsulating unstable compounds. This shift in focus highlights the emerging trend and novelty in the use of Pickering emulsions, particularly their capability to extend the shelf-life and stability of hydrophobic compounds (e.g., β-carotene, curcumin, astaxanthin, lycopene, certain essential oils, hydrophobic vitamins) when exposed to gastro-intestinal conditions. Moreover, the innovative applications involving packaging materials, fat-replacers, and edible inks for 3D-printing food products, having gained significant traction in the last 2–3 years, put into relevance the vast and yet largely unexploited potential of Pickering emulsions in advancing food technology.
Stabilizing particles in Pickering emulsions
The stabilizing effect of solid particles on emulsions has been identified for the first time by Ramsden in 1903, and experimentally confirmed by Pickering four years later [12]. Pickering emulsions are emulsions stabilized by the adsorption of insoluble solid particles at the O-W interface through steric, electrostatic, and hydration repulsions [7]. Their greater stability compared to conventional emulsions can be explained by considering the adsorption and desorption energy of the particles at the interface, defined by the thermal energy of Brownian motion (Equation 1).
where E represents thermal energy (Joules); kB represents Boltzmann constant (Joules/Kelvin); T represents absolute temperature (K).
For surfactants used in conventional emulsions, the desorption energy ΔGd is less than 10× kBT, which results in a dynamic equilibrium of interface adsorption/desorption that makes the adsorption reversible [13]. In contrast, when particles with “appropriate” contact angles (θ) (far from 0° and 180°) stabilize emulsions, ΔGd is much greater than kBT, leading to practically irreversible adsorption.
The adsorbed particles cause the interface to curve towards the phase with lower affinity, and thus, the surface properties of the particles will determine the type of emulsion: more hydrophilic particles will be more suitable for oil in water (O/W) emulsions, and more hydrophobic particles for W/O emulsions (Figure 1).
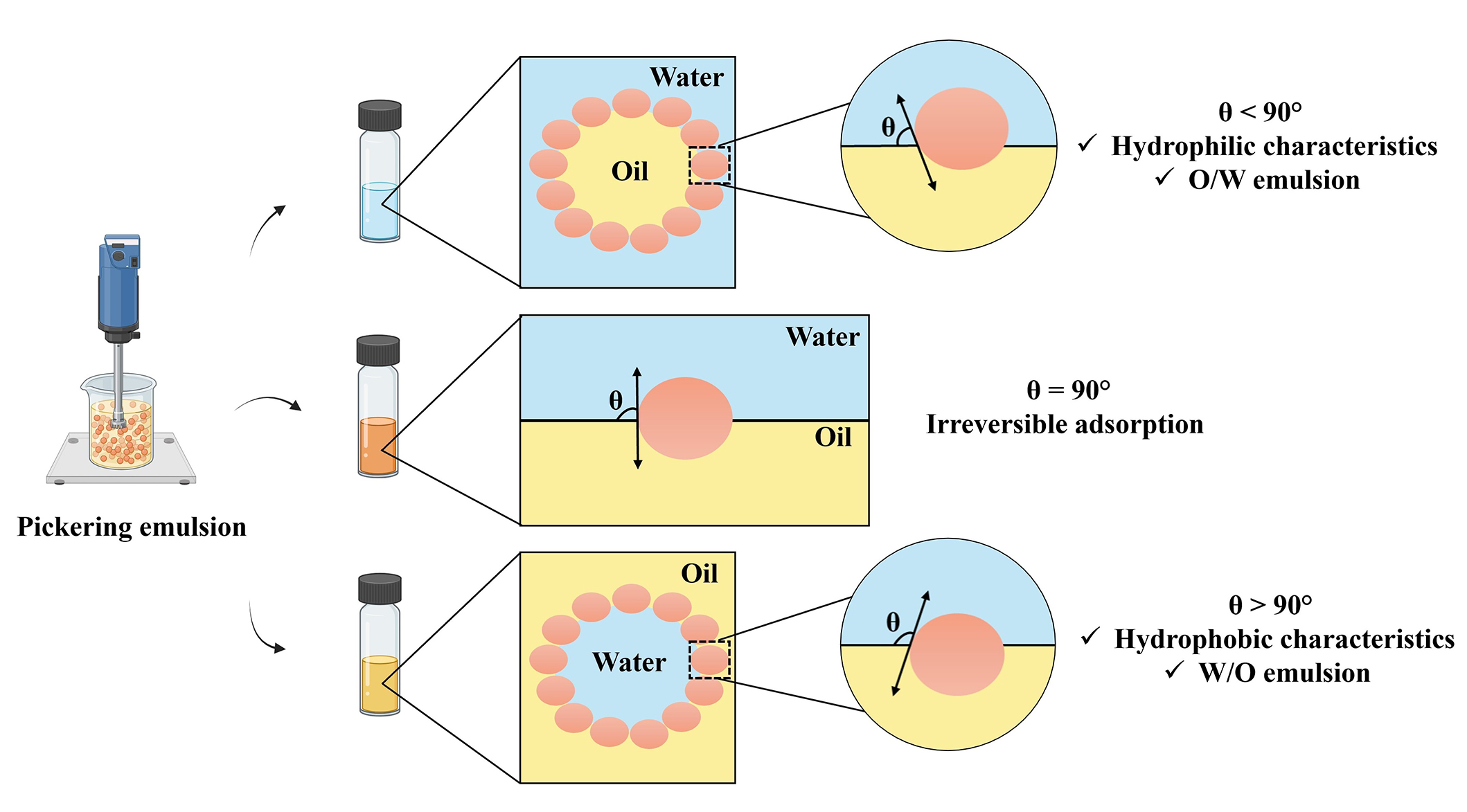
The contact angle of spherical particles at the interface of oil and water and the favored type of emulsion. O/W: oil in water; W/O: water in oil
For applications in food and nutraceutical products, the particles adsorbed to the interface of Pickering emulsions should have a QPS (Qualified Presumption of Safe, EFSA) or GRAS (Generally Recognized as Safe, FDA) status [14]. This not only ensures consumer safety but also meets the demand of plant-based consumers, who are often concerned about the sustainability of production processes. This has been the main reason why applications of Pickering emulsions in the above-mentioned industries are relatively recent. In the next section, the use of proteins, polysaccharides, and microorganisms will be thoroughly discussed, as examples of natural GRAS stabilizing particles, highlighting the advantages of using them as feasible strategies for applying Pickering emulsion in real products.
Natural solid particles stabilizing Pickering emulsions
Natural compounds mostly used to stabilize Pickering emulsions include different biopolymers (proteins, carbohydrates) and QPS microorganisms (Figure 2) [11].
Proteins
Proteins are excellent candidates for stabilizing Pickering emulsions due to their adsorption ability at the oil-water interface, forming a dense layer that significantly reduces interfacial tension. Additionally, they may enhance emulsion stability through electrostatic repulsion and steric hindrance, preventing droplet coalescence, Ostwald ripening, or phase separations. The use of proteins as Pickering particles offers versatility due to their biocompatibility, biodegradability, and responsiveness to environmental changes such as pH, temperature, and ionic strength.
However, for proteins to act as efficient stabilizers, certain surface properties of the protein particles should be carefully considered. For example, the protein’s isoelectric point (pI) is an important parameter that determines the stabilization of Pickering emulsions. At pH values far from the pI, proteins carry a net charge, which enhances stabilization through electrostatic repulsion between droplets. Conversely, when pH = pI, proteins have no net charge, leading to increased aggregation and potential coalescence, thereby reducing emulsions’ stability. Thus, optimizing the pH and ionic strength of the aqueous phase is crucial to enhancing proteins’ solubility and electrostatic stabilization. Another important aspect to consider is the molecular size of proteins. Smaller proteins can form more cohesive interfacial layers, enhancing emulsion stability while still retaining their functionality. These proteins can quickly adsorb to the oil-water interface and cover droplets effectively, providing better stabilization.
Controlling protein aggregation can also enhance the stabilization of Pickering emulsions. Self-aggregation involves the interaction of protein molecules with each other to form larger complexes or aggregates. This depends on the inherent properties of proteins (e.g., molecular weight, surface charge, hydrophobicity) and occurs under certain conditions, namely pH, temperature, ionic force, presence of other molecules, protein concentration, and processing conditions. As aggregates form larger structures with increased surface area, self-aggregation can be promoted in the formulation of Pickering emulsions. This leads to more efficient adsorption at the interface with better packing arrangements and rheological properties. Several authors have exploited proteins’ self-aggregation to fine-tune proteins as stabilizers of Pickering emulsions (Table 1). Physical treatments, including mild thermal treatments, ultrasonication, high pressures, precipitation with antisolvents, solvent evaporation, and pH adjustment, can also contribute to facilitating the adsorption of proteins to the emulsions’ interface. Another strategy widely employed to optimize the stabilizing capacity of proteins is to esterify them with octenyl succinic anhydride (OSA) esterification, which covalently binds specific amino acid residues (e.g., lysine) on the protein surface. As a result, the superficial properties of the proteins are modified, making them more suitable for stabilizing emulsions intended for food or nutraceutical applications [15–18].
The interaction of proteins with carbohydrates can also improve the emulsifying properties by providing additional steric or electrostatic stabilization [14]. Such interaction can occur both by covalent or non-covalent bonds, enzymatically (e.g., transglycosidases or glycosyltransferases) or chemically (e.g., reductive amination, reductive alkylation to lysine residues on the surface), and results in the modification of surface properties, leading to better interface adsorption. Glycosylated proteins usually exhibit better stability in a wide range of pH and temperatures, thus making emulsions more stable to processing conditions. The choice of carbohydrate moiety and the degree of glycosylation can be tailored to engineer the most appropriate surface properties leading to stabilized emulsions with the most appropriate rheological properties (Table 1). The proteins mostly used to stabilize Pickering emulsions include whey, zein, soy, and pea ones [19].
Native whey proteins are not suitable to stabilize Pickering emulsions and their utilization requires treatments including aggregation (heat denaturation), treatment with tannic acid, glycosylation with glucose, lactose, or maltodextrin to produce nanofibrils suitable to adsorb at the interfaces, and also production of nanoparticles generated by Ca2+ cross-linking (Table 1). Treatments with high hydrostatic pressures have been also employed to modify whey protein isolate surface.
Protein particles usually employed to stabilize Pickering emulsions (publications since 2020)
Proteins | Modifications | Encapsulated bioactive | Characteristics of the emulsions | Reference |
---|---|---|---|---|
Whey protein | Glycosylated whey protein isolate-chitosan complexes | Algal oil [docosahexaenoic acid (DHA)] | -Better thermal, storage, and oxidative stability.-Efficient release of free fatty acids during digestion.-Increased bioavailability of DHA. | [20] |
Whey protein isolate fibril complexed with hordein (main storage protein in barley seeds, with high content of hydrophobic aminoacids) by anti-solvent precipitation method at pH 2.5. | Quercetin | Enhanced bioaccessibility of quercetin. | [21] | |
Pectin methyl esterase-responsive nanocomplex prepared using heat-induced whey protein isolate and high methoxyl pectin (pH 4.5, 85°C, 15 min). | Thyme essential oil | -pH stability (stabilizing effect of hydrophobic, hydrogen bonding, and electrostatic interactions).-Pectin methyl esterase triggers the demethylation of high methoxyl pectin within the Pickering emulsion, conferring response characteristics to the enzyme (control of the thyme essential oil release). | [22] | |
Whey protein isolate-vanillin complexes (Schiff-base reaction) | Vitamin E | -Bacteriostatic agent.-Enhanced bioaccessibility of vitamin E in the small intestine (81%). | [23] | |
Whey protein gel particles prepared by heat-induced, enzyme cross-linking and calcium ion cross-linking methods | CoQ10 | -Increased bioavailability of CoQ10.-Stability of emulsions 4°C for 28 days. | [24] | |
Whey protein with tannic acid, gallic acid, tea polyphenol, and vanillic acid | Vitamin D | -Enhanced bioaccessibility of vitamin D.-Enhanced stability of the emulsions. | [25] | |
Whey protein isolate covalently conjugated with epigallocatechin-3-gallate | Lactiplantibacillus plantarum | Stable systems for L. plantarum delivery. | [26] | |
Whey protein isolate glycosylated with short chain inulin/cyanidin 3-glucoside | Curcumin | Increased bioaccessibility of curcumin. | [27] | |
Heat-denatured whey protein | DHA oil | Enhanced bioaccessibility of DHA. | [28] | |
Resveratrol crystals dissolved in ethanol, added to soy proteins dispersed in deionized water at different pH | Vitamin D3 | Protection of resveratrol against precipitation and oxidation. | [29] | |
Whey protein isolate fibrils | Nobiletin | -Improved long term stability.-Extent of lipolysis.-Increased nobiletin bioaccessibility. | [30] | |
Whey protein isolate nanofibers prepared with subcritical water | Curcumin | Better loading effect and antioxidant activities. | [31] | |
Whey protein isolate/epigallocatechin-3-gallate covalent conjugates obtained by free-radical induction reaction | Lactiplantibacillus plantarum | Enhanced viable cell count after 14 days of storage and gastro-intestinal digestion. | [32] | |
Whey protein isolate microgel cross-linked with organic acids (tannic and citric acids) | Roasted coffee oil | Tannic acid resulted in a suitable crosslinker for providing stability to whey protein isolate emulsions. | [33] | |
Gel protein isolate gel particles obtained by high hydrostatic pressure treatments | Curcumin | -High loading efficiency of curcumin.-Stability against light degradation. | [34] | |
Whey protein isolate microgels, natural whey protein isolate, Gum arabic, whey protein isolate combined with gum arabic, maltodextrin, and modified starch (Capsul®) | Pomegranate seed oil | -All particles protected pomegranate seed oil from oxidation.-Whey protein isolate combined with modified starch was protecting the best. | [35] | |
Zein protein | Zein and tannic acid complexes | Cinnamon essential oil | -Tannic acid decreases the superficial tension and accelerates zein adsorption.-Controlled release of cinnamon essential oil.-Antimicrobial activity against spoilage organisms. | [36] |
Zein non-covalently bonded sodium abietate | Avermectin (pesticide) dissolved in tea tree oil | -Delivery system for pesticides (faster release at acidic or alkalyne conditions in comparison with neutral ones).-Enhanced UV-resistance of avermectin.-Antibacterial and insecticidal activities in vitro. | [37] | |
Pea protein isolate-zein complex particle prepared by hydrophobic interactions | Curcumin | -Good storage stability (up to 30 days).-Ionic strength resistance (up to 500 mM).-High-temperature stability (80°C, 48 h).-pH stability (pH 2–9).-Enhanced stability of curcumin. | [38] | |
Zein-gallic acid covalent complex prepared by alkali treatment | Cinnamon essential oil | Pickering emulsions incorporated into chitosan films facilitated a slow release of the essential oil, extending the antimicrobial activity of the films. | [39] | |
Zein/tannic acid nanoparticles are obtained by covalent interactions of tannic acid with zein amino groups, followed by self-assembling to form nanoparticles through antisolvent precipitation | Oregano oil | -Successful incorporation of the emulsions into konjac glucomannan films.-Enhanced antibacterial and antioxidant activities of the films. | [40] | |
Zein/hyaluronic acid nanoparticles obtained by non-covalent interaction | Astaxanthin | -Stability of emulsions towards pepsin hydrolysis.-Enhanced bioaccessibility of astaxanthin. | [41] | |
Zein-tannic acid-sodium alginate complexes | β-carotene | -Good pH and ionic strength stability.-Enhanced bioaccessibility of fatty acids and carotene.-Rheological properties support the potential application of edible ink. | [42] | |
Covalent and non-covalent zein-gallic acid composite nanoparticles | Astaxanthin | Covalently bonded composites significantly delayed the oxidation of the encapsulated algal oil, protected astaxanthin from heat, and increased its bioaccessibility. | [43] | |
Zein nanoparticles and gum Arabic | Peach polyphenols | -Improved stability of peach polyphenols during UV irradiation, storage, and heating.-Enhanced bioaccessibility of polyphenols. | [44] | |
Zein nanoparticles | Cinnamon essential oil | Enhanced antimicrobial properties and control release of chitosan/gelatin films. | [45] | |
Zein-proanthocyanidins-pectin ternary composites | Curcumin | -Long term stabilized gel-like emulsions.-Enhanced bioaccessibility of curcumin. | [46] | |
Zein/Adzuki bean seed coat polyphenol nanoparticles | Astaxanthin | -Enhanced stability of the emulsions against ionic strength and heat treatment.-Retaining astaxanthin after exposure to high levels of UV light irradiation.-Enhanced bioaccessibility of astaxanthin. | [47] | |
Zein nanoparticles coated with bioactive glycyrrhizic acid, through cross-linking with tannic acid | Curcumin | Enhanced bioaccessibility of curcumin when orally administered. | [48] | |
Zein-lecithin-epigallocatechin complex nanoparticles | Peppermint oil | Set-up of the formulation and physico-chemical characterization. | [49] | |
Zein and sodium caseinate nanoparticles | Clove essential oil | -Clove essential oil-loaded zein-sodium caseinate successfully incorporated into chitosan films, decreasing the water vapor permeation.-Controlled release of clove essential oil from the films in 96 h.-Increased tensile strength and break elongation of chitosan films.-Increased antibacterial properties. | [50] | |
Bare zein particles (hydrophobicity modulated by changing pH) | Lactiplantibacillus plantarum | -Optimal pH for zein particle adsorption: 6.6–8.9.-Storage stability of microorganisms at 4°C. | [51] | |
Zein colloid particles | Clove essential oil | -Emulsions incorporated into chitosan films.-Enhanced antibacterial properties of the films with Pickering emulsions. | [52] | |
Soy protein | Green tea polysaccharide conjugates-soy protein isolate complex | Curcumin | Protection of curcumin from adverse pH, light, and temperature effects, with a retention rate of over 74.00%. | [53] |
Crosslinking soy protein isolate and chitooligosaccharide using genipin | Fucoxanthin | -Improved fucoxanthin light retention.-Theoretical support to preserve hydrophobic nutrients in commercial products. | [54] | |
Soybean protein isolate-citrus pectin-gallic acid complex | β-carotene | Theoretical guidance for the design of protein-polysaccharide-polyphenol stabilized Pickering emulsions. | [55] | |
Soy protein hydrolyzate microgel particles produced at various pH (3, 5, 7, and 9) with and without ultrasonication | Quercetin | -Storage stability of the emulsions.-Suitable system to efficiently encapsulate quercetin, and also for its sustainable release. | [56] | |
Pea protein | Ultrasound-treated pea protein isolate and mung bean starch complexes | β-carotene | -Improved stability of β-carotene.-Higher bioaccessibility of β-carotene. | [57] |
Pea protein isolate-quillaja saponin-tannic acid self-assembled nanoparticles through non-covalent interactions | Curcumin | -Theoretical support for multi-scale exploration of structure-properties relationships of nanoparticle.-Tannic acid provides additional stability to the emulsions. | [58] | |
Pea protein-κ-carrageenan complexes | Curcumin | Enhanced stability of curcumin for the generation of 3D printed cake decorations. | [59] | |
Pea protein isolate nanoparticles obtained by heat-assisted pH-shifting | Curcumin | Theoretical basis for fabricating a prospective delivery system for improving bioavailability of hydrophobic nutraceuticals. | [60] | |
Hydrolyzed pea protein at pH 3 and overnight storage at 4°C | Thymol | Smart release of bactericidal agents. | [61] | |
Pea protein-naringin complexes | Naringin | Mask the bitter taste of naringin. | [62] | |
Pea protein amyloid fibrils obtained by thermal treatment of purified pea protein in an acidic environment, leading to hydrolysis and re-assembly | Lutein | Stability of lutein against ultraviolet irradiation, heating, and iron. | [63] | |
Pea protein-pectin-epigallocatechin gallate complexes for extrusion 3D-printing | Cinnamaldehyde | Retain the cinnamaldehyde flavor, which supports the incorporation of emulsions in printed food. | [64] | |
Pea protein and high methoxyl pectin colloidal particles | β-carotene | Enhanced stability and controlled release of β-carotene. | [65] |
Zein is a natural amphiphilic protein that can be extracted from corn and is also a co-product of the ethanol industry. It has a high percentage of nonpolar aminoacids (e.g., proline, alanine, leucine) and based on its insolubility in water and solubility in alcohol, it can form colloidal particles through self-assembly without surface modification at pH different from its pI [66]. Its solubility properties make it a suitable compound to obtain colloidal particles by nanoprecipitation (anti-solvent precipitation). Using zein as Pickering emulsions’ stabilizers requires the set-up of particle concentration, pH, and ionic strength. Stable zein composite particles can be generated using biodegradable and edible materials including sodium caseinate, sodium alginate, or chitosan, through electrostatic adsorption [66] or producing zein complexes with xanthan gum, which improves viscoelastic properties (Table 1).
Soy proteins contain essential aminoacids, flavonoids, and vitamin E, whose two major components are glycinin and β-conglycinin. They consist of a hydrophilic shell and a hydrophobic kernel in aqueous environment [67]. This explains the good emulsifying and gelling properties of soy proteins, although pH, solubility, ionic strength, and temperature shall be controlled when used as Pickering stabilizers. Soy protein isolates have been complexed with pectins through electrostatic interactions to be suitable as Pickering stabilizers [68]. In addition, Maillard reaction has been also used to generate complexes between soy proteins and carbohydrates, leading to an enhanced stability of Pickering emulsions [69] (Table 1).
Pea proteins arise from yellow peas, having gained attention as stabilizers of Pickering emulsions because of their functional properties, sustainability, and allergen-free nature. They have amphiphilic properties, which makes them suitable to adsorb at the O/W interface through their hydrophobic aminoacid residues (e.g., leucine, isoleucine, and valine). The concentration of pea proteins shall be optimized in order to control the size of the emulsions’ droplets and prevent coalescence. Being stable in a wide range of pH and ionic strength, pea proteins are suitable stabilizers of Pickering emulsions submitted to food processing. However, they impart color and flavor that cannot be disregarded and their interaction with polyphenols must also be considered as this can lead to a decrease in the functionality of antioxidants (Table 1).
This section highlights the need for deep knowledge about the physico-chemical properties of proteins (both general and particular to each protein) to develop the best strategy for application in real products. Compared to synthetic stabilizers, proteins offer a natural and sustainable alternative, which is particularly advantageous in food and nutraceuticals, providing a versatile and eco-friendly option. However, as previously explained, their application may require prior treatments and careful consideration of formulation parameters to achieve optimal results.
Polysaccharides
Polysaccharides represent other groups of compounds, widely employed in the formulation of Pickering emulsions. Unlike protein-based particles, which reduce interfacial tension and form a viscoelastic film, polysaccharides stabilize emulsions by forming a protective network around the droplets, providing steric hindrance. Besides that, they can introduce additional technological and functional properties, including greater viscosity, gelation, or controlled release of encapsulated compounds. This versatility supports their increasing use to tailor emulsions’ properties and expand the potential of Pickering emulsions in different technological applications, including fat replacement, packaging, or 3D-printing. However, for polysaccharides to effectively act as stabilizers in Pickering emulsions, several key aspects should be considered. For instance, polysaccharides containing charged functional groups can facilitate electrostatic interactions between droplets, thereby enhancing stability. In general, a higher charge density and uniform distribution of these charged groups may promote stronger electrostatic interactions between polysaccharide molecules and the droplet interface, resulting in more efficient stabilization of the emulsion. Polysaccharides should be water-soluble to disperse easily and adhere effectively to interfaces. Additionally, they should have the ability to swell, which increases the viscosity of the continuous phase and reduces creaming, thereby enhancing the stability of the emulsion. Another important aspect to consider is the particle size and concentration of the polysaccharides-based particles. The particles should be small enough to properly adsorb onto the droplet surface. Likewise, increasing the particle concentration is necessary to cover a larger and thicker interfacial area, which reduces the creaming speed of small oil droplets and inhibits Ostwald ripening. Polysaccharides have poor hydrophobicity and need to be modified physically or chemically to enhance their wettability. Another strategy to enhance wettability is by making them interact with other polymers (polysaccharides, proteins). As these properties vary among different polysaccharides, this section will show the most recent findings regarding the most common polysaccharides used for Pickering emulsions: starch, chitosan, and cellulose.
Starch is an amphiphilic, cost-effective, biocompatible, biodegradable, and non-toxic polysaccharide composed of glucose units. Native starch granules are suitable stabilizers of Pickering emulsions because of their size, shape, and stability over a wide range of processing conditions (pH, temperature, shear rate), making them suitable alternatives to traditional stabilizers. However, the poor stability and hydrophobicity weaken its capacity of starch to adsorb to the emulsion’s interface, thus requiring modifications, such as hydrolysis (chemical or enzymatic), nanoprecipitation, recrystallization, or esterification with OSA to increase the hydrophobicity (Table 2).
Chitosan is another polysaccharide occurring in shrimp and crab shells and fungi, as a result of chitin deacetylation. It also has antibacterial properties, is non-toxic and biodegradable, generally employed as a drug delivery system and film-forming material. Because of the presence of hydrophilic groups (-NH3+; -OH−) embedded along its backbone, its utilization as Pickering emulsions’ stabilizer requires processes to increase its hydrophobicity (e.g., pH and adjustment of the degree of deacetylation). At low pH [pH < pKa (6.5)], amino groups are positively charged, which leads to electrostatic repulsion and improves the stability of emulsions. Regarding deacetylation, a moderate one appears to be the optimal one as hydrophobic acetyl groups are not completely removed [70].
Self-aggregation is also a suitable process to promote the adsorption of chitosan particles to the emulsions’ interface. However, ultrasonication should be avoided as a pretreatment, as it was reported to decrease the hydrophobicity of chitosan [71]. As chitosan is one of the few polysaccharides with acidic pKa, it is very suitable to interact with negatively charged groups (e.g., sodium tripolypohosphate) in cross-linking reactions, also employed to modify chitosan and make it suitable as Pickering emulsions’ stabilizer [72]. Complexes of chitosan with proteins (e.g., gliadin) have been also developed as stabilizers, leading to emulsions with high viscoelasticity [73] (Table 2).
Cellulose is a polysaccharide composed of glucose units, insoluble in water and organic solvents [74], naturally occurring in plant cell walls, and also being produced by certain bacteria. When submitted to chemical and mechanical treatments leading to replacement hydroxyl groups with methyl, hydroxypropylmethyl, and carboxymethyl, cellulose’s hydrophobicity becomes suitable to prepare Pickering emulsions. In addition, acid hydrolysis or physical treatments (high hydrostatic pressures) promote the generation of microfibrillated cellulose, macroscopic fibers, or microcrystalline cellulose, that can act as Pickering stabilizers [75]. In turn, bacterial cellulose nanoparticles have demonstrated good hydrophilic and lipophilic properties to stabilize Pickering emulsions at low concentrations of nanofibers [76] (Table 2).
Natural carbohydrate particles usually employed to stabilize Pickering emulsions (publications since 2022)
Carbohydrate particles | Modifications | Encapsulated bioactive | Characteristics of the emulsions | Reference |
---|---|---|---|---|
Starch | Starch nanoparticles obtained by heating under mildly acidic conditions | Catechin | -Catechin encapsulated in the starch nanoparticles exhibits higher water solubility and UV stability than pure ones.-Catechin-starch nanoparticle composites improve the encapsulation efficiency, water-solubility, stability of catechins, and Pickering emulsion stability. | [77] |
Debranched-waxy corn starch and chitosan | Curcumin | -Debranched-waxy corn starch and chitosan polymers lead to greater emulsifying stability and lower gel strength than native starch and chitosan-prepared emulsion.-Enhanced stability and bioaccessibility of the encapsulated curcumin. | [78] | |
Cana edulis starch and starch nanoparticles modified with octenyl succinic anhydride | Curcumin | -Enhanced curcumin storage protection and controlled release.-Stabilizing Pickering emulsions has a positive effect on gut microbiota and improves the intestinal environment. | [79] | |
Quinoa and maize starch nanoparticles prepared by sono-precipitation and modified with nonenyl succinic anhydride and octenyl succinic acid | Ferulic acid | -Stable emulsions against coalescence and Ostwald ripening.-Long-term stability of the emulsions.-Sustained release of ferulic acid from the strong gel network.-Encapsulation efficiency close to 99% after 15 days of storage. | [80] | |
Potato starch and polyvinyl alcohol | Clove essential oil | -Efficiency of encapsulation: 58%, which ensured the antimicrobial effectiveness of clove essential oil.-Emulsions were applied for pork meat preservation, enabling the slow release of the encapsulated compound.-Prolonged preservation period (10 days) and potent inhibition of E. coli and S. aureus. | [81] | |
Ultrasonic esterified corn starch | Tangerine peel essential oil | -Pickering emulsions incorporated into corn films containing purple corncob anthocyanin.-Bacteriostatic ability against E. coli and S. aureus. | [82] | |
Starch nanocrystals and bacterial cellulose nanofibers | Satureja khuzestanica essential oil | Antibiofilm (Salmonella enterica) activity. | [83] | |
Carboxymethyl starch/xanthan gum combinations with different ratios | Pterostilbene | -High encapsulation efficiency (91.2%), enhanced stability of pterostilbene.-Controlled release of pterostilbene in the intestinal tract. | [84] | |
Butyric acid-modified porous starch | Paclitaxel | The emulsifying and sustained release capacity are significantly improved using higher substituted butyric acid-porous starch as stabilizers. | [85] | |
Gliadin/gelatinized starch nanocomposites | Astaxanthin | -Emulsions with shear-thinning behavior and high solid viscoelasticity.-Suitable rheology properties for 3D printing.-Enhanced stability (90% astaxanthin was retained after heating at 95°C for 30 min) and bioaccessibility of astaxanthin. | [86] | |
Acetalized starch-based nanoparticles | Curcumin | -Acetalized starch and its degradation products showed good biocompatibility.-Acid environments promote a better release of the encapsulated curcumin. | [87] | |
Chayote tuber starch functionalized by zein-pectin nanoparticle | Cinnamon essential oil | -Pickering emulsions were incorporated into biodegradable and bioactive starch-based films for food packaging applications.-Improve water-resistance of the films.-Sustained release of cinnamon essential oil into food stimulants. | [88] | |
Corn starch pregelatinized with a cellulose nanofiber | Basil essential oil | -Incorporation of the emulsions into edible coatings to prevent biochemical degradations and minimize color changes of mandarins.-Coated mandarins significantly suppressed quality losses, did not experience a loss of citric acid, and maintained color stability. | [89] | |
Acorn starch | β-carotene | -Low solubility and swelling power, and high retrogradation and gel strength of the acorn starch.-Free/bound phenolics exhibit stronger antioxidant activity.-Outstanding effect for protecting β-carotene against ultraviolet irradiation. | [90] | |
Octenyl succinic anhydride-modified corn, potato, and pea starch nanoparticles | Curcumin | -Stable emulsions against different environmental stresses (pH, ionic strength, and heating) and during 30 days of storage.-No-oiling-off observed over the storage time.-Improved protection of curcumin during storage and controlled release during in vitro digestion. | [91] | |
Octenyl succinic anhydride-starch | Thymol | -Enhanced bactericidal effects against E. coli, S. aureus, and Aspergillus flavus by inducing ROS eruption, membrane lipid peroxidation, and cell shrink.-Time-sustained bactericidal effect (9 days) upon intermittent exposure to E. coli, S. aureus. and A. flavus in vitro (in comparison with thymol alone). | [92] | |
Starch-fatty acid complexes prepared using different long chain fatty acids (myristic acid, palmitic acid, and stearic acid) and native rice starch | Curcumin | Curcumin was successfully retained after 28 days of storage stability (79.4%) and after exposure to gastrointestinal conditions (80.8%), attributed to the enhancement of the coverage of particles at the oil-water interface. | [93] | |
Octenyl succinic anhydride starch/chitosan complexes | Resveratrol | -Strong stability when subjected to light, high temperature, UV radiation, and freeze-thaw treatment.-Resveratrol retention greatly improved with the increasing addition of complexes and resveratrol.-Pickering emulsions were suitable systems to overcome the stratum corneum barrier (ca. 3–5-fold increase in resveratrol deposition) in deep skin compared to bulk oil. | [94] | |
Gliadin/starch nanocomposites | Astaxanthin | -Gelatinized starch improved the wettability of particles, and thus, the stability of emulsions.-Pickering emulsions stable at pH within 3 and 11, and tolerant to high ionic strength (up to 1000 mM NaCl).-Enhanced retention of astaxanthin (half-life 2.3 times longer than that in oil).-Bioaccessibility of astaxanthin ca. 1.5 times higher than that of oil. | [95] | |
Starch crystals and chitosan | Curcumin | -Chitosan-coated emulsions are stable in the mouth and stomach phases, and slowly digested in the intestine phase.-Permeability of encapsulated curcumin enhanced ca. 9.5-fold compared to the curcumin solution.-Enhanced intestinal permeability of curcumin ascribed to the electrostatic mucoadhesion and reversible epithelial tight junction opening effects of the coating. | [96] | |
Dihydromyricetin/high-amylose corn starch composite particles | β-carotene | Stability of β-carotene against UV irradiation, enhanced bioaccessibility and starch hydrolysis inhibition during digestion. | [97] | |
2-(dimethylamine)ethyl methacrylate (DMAEMA) grafted onto maize starch via free radical polymerization | Lipase from Candida rugosa | -Emulsions readily applied as recyclable microreactors for the n-butanol/vinyl acetate transesterification.-Catalytic activity and good recyclability. | [98] | |
Oxidized high-amylose starch | β-carotene | -Stable emulsions at pH within 3 and 7, salt concentrations up to 1 M, and temperatures within –25°C and 80°C.-Storage stability for up to 30 days.-Controlled-release of β-carotene in vitro, with antioxidant activity-maintained ca. 50% of initial activity when exposed to 80°C. | [99] | |
Chestnut starch nanocrystal/macadamia protein isolate complexes | Quercetin | High encapsulation efficiency for quercetin (> 93%). | [100] | |
Starch-based nanoparticles obtained by nanoprecipitation and ultrasonication | Ferulic acid | Preserved ferulic bioactivities in the Pickering emulsions (anti-cancer, anti-diabetic, angiotensin-converting enzyme inhibition). | [101] | |
Ultrasound and high-pressure homogenization treated starch nanoparticles | Carotenoids extracted from the peel of passion fruit (Passiflora edulis) | -Pickering nanoemulsions rich in carotenoids and total phenolic content, with high antioxidant activity and stability.-Stable emulsions to heat and freeze-thaw treatments and storage at 6°C and 25°C. | [18] | |
Chitosan | Cross-linked carboxymethyl cellulose/chitosan submicron particles through polyelectrolyte self-assembly method in conjunction with isocyanide-based multicomponent reactions | Piperine | -Stabilization of the emulsion’s droplets by carboxymethyl cellulose/chitosan particles.-Highly level emulsions regarding changes in pH, temperature, and ionic strength.-Controlled release of piperine in vitro in both acidic and neutral media. | [102] |
β-carboxymethyl chitosan and gelatin-A | Curcumin | Physico-chemical characterization supporting cosmeceutical applications (uptake of curcumin into fibroblasts in vitro). | [103] | |
Chitosan/alginate nanoparticles and Ca2+ | D-limonene | -Set-up of the emulsion’s formulation and encapsulation process.-Encapsulated limonene had higher activity, higher resistance to ultraviolet (UV), and higher temperature than free D-limonene. | [104] | |
Self-aggregated chitosan particles prepared by a pH-responsive self-assembling method by tuning pH, degree of deacetylation, and molecular weight | Curcumin | -Networked structures generated by chitosan aggregation led to highly elastic gels more resistant to the breakdown of Pickering emulsion at ambient temperature.-Molecular weight and degree of deacetylation determine curcumin loading, encapsulation efficiency, and release profile. | [105] | |
Self-assembled chitosan complexed with natural phytosterol particles | β-carotene (hydrophobic) and epigallocatechin gallate (hydrophilic) | -Storage stability at 4°C and 25°C (at least 2 months).-Suitability to co-encapsulate hydrophilic and hydrophobic bioactive compounds, shielding them against UV exposure and long-term storage. | [106] | |
Chitosan-based nanoparticles obtained by ionic gelation modified by flaxseed gum or sodium tripolyphosphate | Ferulic acid | -Complex nanoparticles had high surface activity.-Controlled topical release of ferulic acid.-Ferulic acid in the emulsion had higher penetration and retention ability in the skin dermis. | [107] | |
ε-polylysine-carboxymethyl chitosan nanoparticles | Oregano essential oil | -Pickering emulsions incorporated into gelatin films.-The mechanical properties, barrier properties, anti-oxidation, and antibacterial properties of the films were improved with the incorporation of Pickering emulsions.-Extension of shelf life of beef and strawberries with excellent antioxidant and antibacterial properties. | [108] | |
Resveratrol-grafted zein covalent conjugate combined with quaternary ammonium chitosan | Peppermint oil | Enhanced antioxidant effect against DPPH and ABTS free radicals. | [109] | |
Rice peptide aggregate-chitosan complexes | Curcumin | Enhanced storage stability, lower free fatty acids release, and higher curcumin bioaccessibility (65.2% and 68.2%, respectively). | [110] | |
Soy protein isolate-chitosan nanoparticles | Docohexaenoic acid (DHA) | Enhanced retention rate of DHA under storage, ionic strength, and thermal conditions. | [111] | |
Soybean protein isolate/chitosan hydrochloride composite particles | Citrus essential oil | -Good storage and oxidation stabilities and rheological properties.-Preservative effect on freshly-cut apple slices. | [112] | |
Chitosan and soy protein isolate colloid particles | Cinnamon essential oil | -Pickering emulsions incorporated in collagen films enhanced their thermal stability, UV-blocking properties, and water resistance.-Improved antioxidant (DPPH scavenging activity) and antimicrobial properties (E. coli, S. aureus, P. fluorescence).-4-day shelf-life extension of pork coated with the functionalized films. | [113] | |
Spirulina protein-chitosan complex | Astaxanthin | -Improved the stability of astaxanthin in different environments.-Enhanced bioaccessibility of astaxanthin. | [114] | |
Gallic acid modified-chitosan nanoparticles | Garlic essential oil and curcumin | -Improved bioaccessibility of garlic essential oil and curcumin.-Good biocompatibility and enhanced cellular uptake of garlic essential oil and curcumin. | [115] | |
Chlorella pyrenoidosa protein-chitosan complex | Lutein | -Stability of encapsulated lutein when UV irradiated for 48 hours.-Enhanced bioaccessibility of lutein. | [116] | |
Zein and chitosan nanoparticles | Citral and/or cinnamaldehyde | -Effective antifungal system (decrease of Aspergillus spp. growth and ochratoxin production).-Stable emulsions within 15 days and good sustained release ability during 9-day storage experiment. | [117] | |
Phosphorylated perilla protein isolate-chitosan composite nanoparticles | β-carotene | -No coalescence during long-term storage, centrifugation, and heat treatment.-Increasing the chitosan concentration leads to a progressive strengthening of viscosity, viscoelasticity, and thixotropy-recovery capacity of the emulsions, allowing their controllable injectability and printability during 3D printing.-Enhanced stability of β-carotene in emulsions exposed to environmental stresses. | [118] | |
Phytosterol/chitosan complex particles | Epigallocatechin gallate | Protection of epigallocatechin gallate from heat and pH shock | [119] | |
Chitosan tripolyphosphate nanoparticles | Andrographolide | -Improved apparent digestibility coefficient of protein, fiber, carbohydrates, and energy for carps fed with the encapsulated andrographolide.-Protection of carps against koi herpes virus. | [120] | |
Soybean protein isolate-chitosan composite | Cinnamon essential oil | -Encapsulation efficiency of cinnamon essential oil: 65.23%.-Stabilization of the encapsulated compound. | [121] | |
Resveratrol-loaded α-lactalbumin-chitosan particles | Curcumin | -High (64%) curcumin retention up to 30 days.-Enhanced curcumin bioaccessibility. | [122] | |
Chitosan with different molecular weights functionalized with protocatechuic acid by free-radical grafting reaction, | β-carotene | -Stability of β-carotene upon exposure of emulsions to ultraviolet irradiation, natural light exposure, and heat treatment.-Oxidative stability of β-carotene. | [123] | |
Whey protein isolate-chitosan complexes | Apigenin | -95% of apigenin retention when emulsions are stored under refrigerated conditions.-Enhanced bioaccessibility of apigenin. | [124] | |
Chitosan/guar gum nanoparticles were formed by hydrogen bond interactions between amino groups of chitosan and hydroxyl groups of guar gum. | Astaxanthin | Retention rate of astaxanthin: 86% when stored at 37°C for 30 days. | [125] | |
Alginate-coated chitosan-stabilized | Tocotrienol (vitamin E) | Enhanced retention of tocotrienol upon processing and storage. | [126] | |
Zein-chitosan nanoparticles | Curcumin, oil red, and oil blue | -Better chroma (based on L*a*b* values) with lower incorporation of pigments (under the same amount of pigment, the saturation of the emulsion increases by 81.5%).-Potential application as a color control strategy for complex food systems. | [127] | |
Chitosan nanoparticles obtained by cross-linking with sodium tripolyphosphate | Chlorogenic acid and cinnamon essential oil | -Stable emulsions after 5 days of storage.-Suitable co-encapsulation of cinnamon essential oil and chlorogenic acid. | [128] | |
Carboxymethyl chitosan-sodium alginate nanoparticles to obtain hydrogel emulsions | Curcumin | -Controlled release of curcumin in vitro.-Antibacterial properties against E. coli and S. aureus.-Improved wound healing. | [129] | |
Pea protein isolate-chitosan nanoparticles | Eicosapentaenoic acid | Sustained release in vitro digestion and enhanced bioaccessibility of eicosapentaenoic acid. | [130] | |
Potato protein-chitosan complex | β-carotene | Sustained release rate of β-carotene in vitro. | [131] | |
Ovotransferrin-carboxymethyl chitosan nanoparticles to prepare oleogels | Curcumin | Enhanced bioaccessibility of curcumin, stable during storage and with high retention of the encapsulate. | [132] | |
Ultrasonicated chitosan | β-carotene | Stable emulsions during heating (121°C), processing and storage at 37°C (constant color parameters). | [133] | |
Chitosan nanoparticles produced by self-aggregation or by crosslinking with tripolyphosphate, further freeze-dried, or spray-dried | Roasted coffee | Increased oil retention in the microcapsules spray-drying promotes better retention of polyphenolic compounds and antioxidant activity during in vitro digestion. | [134] | |
Cellulose | Cellulose nanofibrils, holocellulose nanofibrils, and lignocellulose nanofibrils were obtained using deep eutectic solvents | Curcumin | -Encapsulation efficiency of curcumin: 94.80%.-Inhibitory effect against S. aureus. | [135] |
Cellulose nanocrystals and hydroxypropyl methylcellulose | Omega-3 polyunsaturated fatty acids (n-3 PUFA) | -Enhanced bioavailability of omega-3 fatty acids in dogs.-Stomach oxidation of n-3 PUFA prevented. | [136] | |
Nanocellulose synthesized from coconut milk waste residue using 38–42% sulfuric acid and/or ultrasound (5–10 min) separately and in combination | Curcumin | -Stable emulsions at pH 2 and 63°C.-Stomach release: 38%; intestinal release: 52%, which supports emulsions as curcumin delivery systems. | [137] | |
Cellulose nanocrystals | D-limonene | -Pickering emulsions incorporated into citrus pectin-based films aiming to coat fruits.-Improved mechanical properties of the films (tensile strength, elongation), water barrier, and film clarity.-Inhibition of harmful microbes causing rotting of fresh fruits. | [138] | |
Nanocrystalline cellulose | Butterfly pea petal extract rich in anthocyanins | Set the encapsulation conditions to retain the greatest amounts of extracts. | [139] | |
β-cyclodextrin, cellulose nanocrystals, and bacterial cellulose | Citrus essential oil | Controlled delivery system for flavors. | [140] | |
Alginate beads and cellulose nanocrystal-stabilized Pickering emulsion | Curcumin | -Improved storage stability of curcumin (half-life 160 days).-Feasible incorporation of emulsions into milk, apple juice, yogurt, and mineral water, that can be stored u to 26 days.-Full release of curcumin in the intestinal phase of in vitro digestion. | [141] | |
Cellulose nanofibrils and cellulose nanocrystals synthesized from pomelo peels | Lycopene | High ail fractions are beneficial for controlling lycopene release during gastrointestinal digestion. | [142] | |
Cellulose nanocrystals-whey protein isolate complex | Curcumin | -Encapsulation efficiency: 89.4%.-Stable a stomach pH.-Release of curcumin in the intestinal phase. | [143] | |
Bacterial cellulose from fermented kombucha | Curcumin | Enhanced stability (temperatures, low pH, sunlight, UV-365 nm) and antioxidant capacity of curcumin. | [144] | |
Nanofibrillated cellulose | Astaxanthin | -Increased stability of astaxanthin with the increase in the concentration of nanofibrillated cellulose.-Enhanced bioaccessibility of astaxanthin. | [145] | |
Hydrophobic-hydrophilic cellulose particles | Vitamin B9 | Responsiveness of emulsions at pH 2, 4, and 7, vitamin B9 release depending on pH. | [146] | |
Cellulose nanocrystals | Clove bud oil | Pickering emulsions incorporated into pearl millet starch films with antimicrobial activity. | [147] | |
Tempo-oxidized cellulose nanocrystals | Ginger essential oil | -Pickering emulsions incorporated into starch-based films with improved antibacterial activity and tensile strength properties, decreased water vapor permeability.-Improved storage of tomatoes when coated with the films. | [148] | |
Fungal (Pleurotus eryngii) cellulose nanocrystals | Triterpenes | Enhanced stability of triterpenes. | [149] | |
Cellulose nanocrystals | Astaxanthin | -Structural stability of astaxanthin.-Inhibitory effect against E. coli and S. aureus. | [150] | |
Pineapple peel cellulose nanocrystals and (−)-epigallocatechin-3-gallate | Curcumin | -Improved bioaccessibility of curcumin.-Thermal and UV-light stability of emulsions, with a curcumin retention of 92%. | [151] | |
Cellulose nanocrystalline | Curcumin | -Stable emulsions up to 1 month.-Encapsulation efficiency: 99%.-Half-life of encapsulated curcumin: 98 days. | [152] | |
Undaria pinnatifida nanocellulose | Astaxanthin | -Stable emulsions at 50°C and 14 days.-Enhanced bioaccessibility of astaxanthin and release of free fatty acids. | [153] |
ROS: reactive oxygen species
As a whole, polysaccharides like cellulose, chitosan, and alginate offer highly versatile, sustainable, and environmentally friendly alternatives for stabilizing Pickering emulsions, compared to synthetic ones. Optimizing the formulation parameters maximizes the emulsion’s stability and functionality, making them particularly suitable for use in food-related applications such as functional foods, packaging, and 3D printing.
Microorganisms
Microorganisms have also been used as stabilizing particles in Pickering emulsions [13, 154]. In particular, the use of lactic acid bacteria in Pickering emulsions offers safety due to their GRAS and QPS status, and allows adding value to the final product, making microorganisms the choice for food applications [13]. Unlike polysaccharides-based particles, microorganisms stabilize emulsions through the physical adsorption of microbial cells at the interface. Thus, the shape, size, and surface properties of bacteria are relevant characteristics for the effective stabilization of emulsions. Different geometric shapes (e.g., cocci, bacilli) determine their adsorption at the interface and, consequently, the stability of emulsions [155]. The sphericity of particles (the ratio of the surface area of a sphere of equal volume to the actual surface area of the particle) affects flow properties, heat/mass transfer, and wettability at the O/W interface [13].
Regarding surface properties, surface roughness increases the accessible surface area, and surface charge (determined by carboxyl, amino, and phosphate groups) is important in adsorption at the interface. High electronegativity can decrease bacterial adsorption at the interface, while heterogeneity in surface charge increases it [156]. In Gram-positive bacteria, such as lactic acid bacteria, the cell wall has a thick layer of peptidoglycan, lipoteichoic acids, and polysaccharides, and some of them bear S-layer proteins [157]. All these molecules constitute the outermost bacterial layer and allow reducing interfacial tension, improving Pickering emulsion stability [158]. Furthermore, hydrophobic bacteria have an affinity for each other and self-assemble at the O/W interface, allowing them to resist coalescence and deformation, stabilizing O/W emulsions for prolonged periods. That’s why they are the most suitable for stabilizing Pickering emulsions.
There are very few publications in which food-grade microorganisms are used as stabilizing particles in Pickering emulsions, and most of them are from recent years. Firoozmand and Rousseau [155] studied the stabilization of O/W emulsions by S. cerevisiae, Lactobacillus acidophilus, and Streptococcus thermophilus at different microorganisms’ concentrations, observing that some emulsions remained stable for more than four months. Lactic acid bacteria can exhibit a range of surface properties, including both hydrophilic and hydrophobic characteristics, depending on the specific strain and environmental conditions. To enhance their functionality, different methods aiming to modify the physicochemical properties of the bacterial cell wall have been proposed. These methods include fermentation, adsorption of macromolecules such as polysaccharides, polyphenols, and proteins, as well as chemical modifications. Jiang et al. [159] reported that surface modification of L. acidophilus by OSA improved emulsion stability. In addition, the surface properties of L. rhamnosus and L. delbrueckii subsp. lactis have been altered through enzymatic treatments and the adsorption of hydrophobic zein proteins, enhancing their performance as Pickering stabilizers.
Compared to other synthetic stabilizers, several lactic acid bacteria provide the added value of their probiotic properties, leading to additional health benefits. In addition, since several lactobacilli strains are naturally highly hydrophobic, they usually do not require prior treatments to enhance hydrophobicity, as is necessary with proteins and polysaccharides. The use of lactobacilli as stabilizers also aligns with the growing demand for natural and sustainable ingredients. However, the use of lactic acid bacteria as Pickering stabilizers is still very scarce. The main challenge lies in the careful selection of strains and the optimization of emulsion conditions to achieve the desired stability while maintaining bacterial benefits. This opens up significant opportunities for innovative applications in food technology.
Applications of Pickering emulsions
Pickering emulsions offer a natural alternative to traditional emulsifiers without relying on synthetic additives and allowing for the encapsulation and controlled release of bioactive compounds. This supports relevant applications in the food and nutraceutical industries, paving the way for innovation in the formulation of functional foods, improving the delivery and absorption of nutrients and supplements, as well as formulating active packaging, suitable fat replacers, or even ink for 3D printing.
As a whole, the incorporation of Pickering emulsions into those products drives innovation, addressing consumer demand for safe, effective, and sustainable products. The state of the art and potential applications of Pickering emulsions in food and nutraceutical systems are provided in the next sections (Figure 3).
Food and nutraceutical
Using Pickering emulsions in food-related applications has primarily focused on investigating the physico-chemical properties that determine the stability of emulsions. However, the increased stability of Pickering emulsions supports the development of innovative processes to obtain healthier and sustainable food products, overcoming technological limitations and responding to the demands of increasingly exigent consumers.
Incorporation of Pickering emulsions in food formulation
The increased stability of Pickering emulsions provides systems retaining texture, appearance, and extended shelf-life in comparison with conventional emulsions. Besides that, the stabilization of oil droplets with solid particles generates low-fat emulsions without sacrificing sensory attributes, which represents a powerful tool to reduce the amount of fat in food formulations while maintaining the desired texture and mouthfeel. In addition, the possibility of adjusting the size and composition of the stabilizing particles enables precise control over the texture and rheology of food products. This allows manufacturers to tailor the mouthfeel and consistency of different sauces (e.g., salad dressings, mayonnaise), extending shelf-life, and preventing ingredient separation. Furthermore, when the encapsulated compounds impair flavor or aroma, Pickering emulsions appear to be suitable systems to provide prolonged sensory experiences in food products. The incorporation of Pickering emulsions into mayonnaises also represents a suitable alternative to replace egg yolk, thus providing innovative products to vegan consumers. In this regard, Pickering emulsions stabilized by apple pomace particles, gum nanoparticles, curcumin-based solid dispersion particles, pea protein isolate microgels, and black garlic, have been applied for the development of edible mayonnaises [160–164], that demonstrated stability both during storage and against different environmental stresses.
In the formulation of sausages and related food products, Pickering emulsions can act as templates to develop low-viscosity liquid oil into soft gels providing interesting textures that can be used to reduce trans- and/or saturated-fat content in food products, thus being healthier and oxidation-stable alternatives to saturated fatty acids [165–168]. In turn, the textural stability arising from the capacity of insoluble particles to maintain the structure of foam bubbles supports the application of Pickering emulsions in the formulation of aerated products (e.g., whipped creams, mousses, meringues) [169–173].
Considering that stabilizing particles can form a particle-particle network in the space between the droplets, effectively and irreversibly anchored at the oil-water interface, they play a crucial role as “structuring agents”, preventing droplets’ aggregation [174, 175]. Such gels or networks can impair enhanced stability and textural attributes when incorporated into structured foods (e.g., desserts, confectionery, bakery products, and ice cream) [176, 177].
Pickering emulsions in packaging materials
An innovative strategy involves the incorporation of Pickering emulsions into biopolymer matrices to create films, offering a promising alternative to petroleum-based packaging materials. The physico-chemical features of active packaging can be improved by incorporating Pickering emulsions loaded with bioactive substances, which increases their hydrophobicity, and introduces new functionalities. When selecting Pickering particles for film production, it is crucial to consider their long-term stability and desired properties. For example, Zhang et al. [178] discovered that by carefully choosing the concentration of zein-pectin Pickering emulsions loaded with oregano essential oil, they could change the hydrophilicity of Konjac glucomannan films. This change results from the enhanced hydrogen bonding and hydrophobic interactions driven by the amino acids present in zein. Also, Fan et al. [45] found that incorporating cinnamon essential oil-loaded zein Pickering emulsion improved the hydrophobic characteristics of chitosan-gelatin films. This enhancement is attributed to the hydrophobic cinnamon essential oil droplets in the emulsion and the interactions between the film’s biopolymeric matrix and the Pickering emulsions, which partially replace water interactions. In this line, integrating Pickering emulsions loaded with hydrophobic compounds into biopolymer-based films can enhance their barrier properties by establishing a uniform network structure within the film matrix that delays the diffusion of water molecules [45, 178]. Regarding the impact of adding Pickering emulsions on the mechanical properties of biopolymer-based films, results vary according to the polymers used, the bioactive compound encapsulated, and the concentration of Pickering particles employed. On the one hand, Yang et al. [39] noted that incorporating a small quantity of cinnamon essential oil Pickering emulsion, stabilized by zein-gallic acid, into chitosan films led to enhanced mechanical properties. This improvement was linked to the dispersion of the low-concentration emulsion within the films, which filled gaps and improved the polymer matrix’s continuity. Additionally, these authors suggested that introducing solid particles might facilitate the formation of new intermolecular hydrogen bonds with the film-forming matrix polymer, contributing to an increase in the cross-linking within the films [39]. Conversely, increased incorporation of Pickering particles may weaken the hydrogen bonds within the film matrix and substitute strong polymer-polymer interactions with weaker polymer-oil interactions. This could result in structural discontinuities thus, affecting the tensile properties of the film, and potentially compromising its structural integrity during processing, transportation, and storage [179]. Based on these findings, the concentration of Pickering emulsion, droplet size, and distribution of the loaded Pickering emulsion within the film during casting should all be carefully examined when producing a biopolymer-based film containing Pickering emulsion for a specific application.
Furthermore, Pickering emulsions incorporating bioactive ingredients, such as essential oils or phenolic compounds, are designed to improve the film’s physico-chemical properties while also imparting antibacterial and antioxidant activities. These films work as active packaging systems, regulating the release of bioactive into the headspace throughout storage to prevent microbial growth and oxidative reactions, thus increasing the shelf-life of food products. Recent research has developed packaging films incorporating Pickering emulsion to preserve excellent postharvest quality and increase the shelf-life of mangoes [180], strawberries [181], and cherry tomatoes [182]. Likewise, biopolymer-based films containing Pickering particles have been successfully employed to enhance antimicrobial and antioxidant properties, as well as pH sensitivity, for potential applications in preserving and indicating freshness in pork meat [183] and fish [184]. Additionally, films incorporating Pickering emulsions loaded with essential oils exhibited antifungal activity, extending the shelf-life of sliced bread for up to nine weeks [185]. These recent findings from the last three years demonstrate the considerable potential of Pickering emulsion films for applications in food packaging, indicating ample opportunity for further innovation.
Pickering emulsions in 3D printed food products
The use of Pickering emulsions as building blocks to construct 3D scaffolds with customized structures and programmable functions is one of the latest trends in the applications of these emulsions, and food technology is one of the main applications’ fields [186]. Indeed, this has emerged as an innovative approach with an increasing number of reports in the latest years (Tables 1 and 2). Some of the applications include their use as fat replacers for decorating pastry [59, 64, 187–193].
Pickering emulsions are excellent systems to innovate in food products, including their formulation, processing, and product development, enabling the creation of healthier, more stable, and functional food options. The compatibility of Pickering emulsions with 3D-printing technology also opens up possibilities to create complex and tailorable food structures, that can be incorporated into different sectors (e.g., functional foods, personalized nutrition). This enables the production of food products, not only stable and nutritious but also aesthetically appealing, which can be particularly beneficial in gastronomy, thus transforming the culinary landscape by merging technology with traditional food preparation.
Pickering emulsion for nutraceutical applications
The capacity of Pickering emulsions to encapsulate bioactive compounds is somehow a “conservative” approach, extending upon the capabilities of conventional emulsions in this regard. However, the superior stability of Pickering emulsions makes them suitable delivery systems for bioactives with low bioaccessibility, ensuring their safe arrival to the intestine [194]. Comprehending the release capacity and gastrointestinal stability of lipophilic compounds encapsulated within Pickering emulsions is paramount, particularly in their potential application as nutraceutical products. The digestion process of Pickering emulsions varies based on their intended objectives, either to delay lipolysis, thereby aiding in post-meal satiation as a strategy to reduce calorie intake, or to facilitate the delivery of bioactive compounds. Therefore, it is essential to design Pickering emulsions that tune factors like particle concentration, particle shape and size, and oil fraction to create Pickering particles with high desorption energy that reduce lipid release [14]. Conversely, when designing Pickering emulsions for the delivery of bioactive compounds, it is important to ensure that the emulsion provides sufficient stability for the bioactive compounds to remain intact during passage through the gastrointestinal tract and enables their release upon reaching the intestine for absorption [195]. In this regard, the range of investigated low bioaccessible compounds remains somewhat limited, primarily focusing on β-carotenes, curcumin, lycopene, lutein, ω-3 fatty acids, essential oils, and certain vitamins (A, D3, E, K) [194] (Tables 1 and 2). However, there is limited understanding regarding the actual absorption of these compounds through intestinal cells, emphasizing the need for future in vivo and in vitro studies to address this gap. Moreover, given that most studies have primarily simulated conditions only up to the upper gastrointestinal stage, there has been limited exploration of the digestion of Pickering emulsions during colonic fermentation and their potential implications for human health through interactions with gut microbiota [196]. This is particularly crucial for those Pickering emulsions that maintain remarkable stability throughout gastrointestinal conditions, potentially reaching the colon in an undigested form. In a recent study, Hu et al. [196] observed that particle size influenced lipid digestibility, with smaller particles showing increased digestibility and release of bound phenolics during gastrointestinal digestion and colonic fermentation. The released phenolics displayed antioxidant activity even after colonic fermentation and were found to enhance the production of short-chain fatty acids linked to intestinal health [197]. Moreover, the released phenolics from Pickering emulsion stabilized by media-milled black rice particles were observed to modulate the composition of intestinal flora, suppressing harmful bacteria while promoting the growth of beneficial bacteria [196, 197]. These findings underscore the importance of exploring deeper into gastrointestinal digestion and examining the effects of Pickering emulsions on gut microbiota, which can in turn influence biological activity. This highlights the considerable room for innovation in this field.
Conclusions
Pickering emulsions revolutionized different aspects of food science and technology, addressing several challenges faced by the food industry, and making them valuable assets in the pursuit of sustainable and innovative food solutions. Their potential extends far beyond that of conventional emulsions offering sustainable solutions for delivery, fat replacers, film-makers and 3D food printing applications.
The future of Pickering emulsions in food and nutraceutical technology holds significant potential for innovation and enhanced functionality. One of the most promising areas is the delivery of hydrophobic compounds (e.g., vitamins, essential oils, and bioactive lipids), which are often challenging to incorporate into aqueous food systems. Advancements in understanding the interfacial properties of the different natural stabilizers addressed in this work (proteins, polysaccharides, microorganisms) will further optimize the stability and delivery efficiency of these emulsions. Another impactful consideration is the development of smart Pickering emulsions responding to environmental triggers (pH, temperature changes). This capability significantly contributes to the controlled release of encapsulated nutrients, including targeted release in specific organs and topical applications (e.g., biomedical applications, where targets may be at different temperatures, temperature-responsive stabilizing agents might be particularly useful).
Beyond delivering hydrophobic compounds, Pickering emulsions are poised to revolutionize cutting-edge food applications by offering new textures, flavors, and functional properties. The ability to create stable emulsions with natural, clean-label ingredients aligns with consumer demand for healthier and more sustainable food products. Future research may focus on tailoring the sensory attributes of Pickering emulsions to mimic or enhance traditional food textures (e.g., creaminess, juiciness), while maintaining nutritional value. Moreover, the incorporation of bioactive compounds into Pickering emulsions can transform them into multifunctional ingredients, providing not only nutritional benefits but also improving food safety and shelf life through antimicrobial properties. As the field advances, collaborations between food scientists, nutritionists, and material scientists will be crucial in developing innovative Pickering emulsion-based products that meet the evolving needs of the food and nutraceutical industries.
Abbreviations
GRAS: | Generally Recognized as Safe |
O/W: | oil in water |
OSA: | octenyl succinic anhydride |
pI: | isoelectric point |
QPS: | Qualified Presumption of Safe |
Declarations
Author contributions
AGZ: Conceptualization, Investigation, Writing—original draft, Writing—review & editing. LC: Investigation, Writing—original draft, Writing—review & editing. Both authors read and approved the submitted version.
Conflicts of interest
Both authors declare that they have no conflicts of interest.
Ethical approval
Not applicable.
Consent to participate
Not applicable.
Consent to publication
Not applicable.
Availability of data and materials
Not applicable.
Funding
This work was supported by the Argentinean Agency for Scientific and Technological Promotion (ANPCyT) [PICT/2020/0482, PICT (2020)/1602]. Lucía Cassani acknowledges Consellería de Educación, Ciencia, Universidades e Formación Profesional (Xunta de Galicia) for the financial support [ED481D-2024-002]. Andrea Gomez-Zavaglia is member of the research career of CONICET. The funders had no role in study design, data collection and analysis, decision to publish, or preparation of the manuscript.
Copyright
© The Authors 2024.