Abstract
Aim:
Triticale is a well adaptable crop, tolerant of disease and abiotic stresses, and able to grow with good yields even in poor soil, thus representing a good choice to develop a new industrial agri-chain in Italy in a sustainability contest, to cope with its soil problems due to incoming desertification.
Methods:
Two triticale elite lines were grown in marginal lands in controlled field experiments. The lines were harvested at two different development stages, namely green mass and seeds, and suitable standard protocols were applied to test their potential to produce bioethanol in line with the emerging bioenergy processes.
Results:
The protocols applied were able to obtain bioethanol with a good yield from both feedstocks. In particular, very efficient fermentation kinetics was observed using seed feedstock, with a sharp curve between 15 h and 24 h, reaching 84% of the total alcohol obtained (final time 72 h).
Conclusions:
Therefore, the results of this research point to new sustainable potential for industrial applications of triticale crops in Italy. Furthermore, the high activity of the endogenous amylolytic enzymes, mainly α-amylase, and the high starch content suggest the potential use of triticale in other industrial applications, like the brewing industry.
Keywords
Industrial crop, triticale, bioethanol, fermentation, beer productionIntroduction
Triticale is a hybrid cereal produced by crossing a wheat “mother” with a rye “father”. Triticale hybrids are all amphidiploid, which means the plant is diploid for two crossed genomes and is thus an allotetraploid [1]. Consequently, combining the parental traits such as rusticity, tolerance to biotic and abiotic stresses, high yield potential, and good grain quality, triticale represents a chance to optimize land use and recover marginal soils, in the framework of sustainable rural development. In particular, triticale has a positive environmental impact through its ability to reduce leaching into groundwater, thus acting as a soil improver, due to its extensive root system which is able to bind erosion-prone soils [2]. After its first constitution in 1875 in Scotland and further breeding experiments by Rimpau in 1888, several scientists worldwide developed different varieties that, through ups and downs, were commercially released in the ‘60s. The triticale improvement program at CIMMYT (Centro Internacional de Mejoramiento de Maíz y Trigo, MX) started in 1964 and continues currently to be the leading international program worldwide [2]. At Italian National Agency for New Technologies, Energy and Sustainable Economic Development (ENEA), the triticale breeding program started in the late ‘60s and released two varieties, Mizar (1979) and Rigel (1983), both characterized by high yields and good resistance to pathogens and still grown in Italy. The ENEA triticale breeding program continued with the release of the Quirinale triticale variety in 2014.
Currently, triticale is grown in more than 30 countries, including European countries, Canada, China, Australia, and Mexico, and is mainly produced in Europe, with a 90% world harvest of which 78% in the European Union (EU), in 2021. Poland is the main European producer (36%), with more than 3 million acres harvested (https://data.un.org/Data.aspx?d=FAO&f=itemCode:97).
Bioethanol from biomass is considered an important renewable alternative to fossil fuels in the EU’s transport sector, helping to reduce greenhouse gas emissions and improve the EU’s security of supply [3–5]. The EU is committed to promoting the use of renewable energy, particularly in transport (under the Renewable Energy Directive), with an at least 14% share by 2030, including a minimum share of 3.5% for advanced biofuels [6]. The EU emphasizes the necessity of sustainability of the production of biofuels, including bioethanol, in order to avoid threatening biodiversity and food security [7]. Most bioethanol sold in the EU is produced by fermenting sugars contained in crops like wheat, maize, and sugar beet (first-generation bioethanol), possibly raising concerns. The EU Commission encourages research into developing new materials to be used in bio-alcohol production, taking into account the environment and sustainability, especially as regards the use of agricultural wastes such as lignocellulosic raw materials (second-generation bioethanol) [8].
Triticale grains are considered a very promising biomass source in temperate regions of Europe [9, 10]. In Poland, attention is paid mainly to variety selection, agriculture conditions, and fertilization, in order to evaluate their effects on feedstock yields and bioethanol produced. For triticale, Klikocka et al. [11] reported a mean theoretical ethanol efficiency of 479.5 L/ton and a mean yield of 2,486.2 L ha–1. In Canada, large efforts are being made to identify the best candidate crops; in a study, 16 cereal cultivars, including different soft wheat and triticale lines, were analyzed. The conclusion was that triticale was found to be superior to the other crops tested in many agronomic traits related to ethanol and biomass production [12]. On the other hand, the production of ethanol from lignocellulosic raw material is considered the most promising option for the future, but it is still not fully competitive [13], even if significant progress has been made and the first advanced industrial demonstration bioethanol plant in the EU was built in Italy [COMETHA project 2014–2018 industrial scale pre-commercial plant, Porto Marghera, Italy, supported by Framework Program for Research 7 (FP7)] [14].
In this study, eight triticale elite lines constituted in the framework of the triticale breeding program at CIMMYT were grown in marginal areas in rainfed experimental fields using a low-input farming system to investigate their adaptability and performance in Italy. They were characterized during various crop seasons and the potential bioethanol yield was evaluated. Two different fermentation substrates, namely green biomass and meals (milled seeds) were tested. Two protocols were selected in the literature and applied, taking into account their final conclusions, in order to evaluate the potential of these triticale lines to produce bioethanol. The results from triticale seeds appeared particularly interesting as the good level of ethanol achieved using exclusively endogenous amylolytic enzymes suggested an additional application in the brewing industry, either as the main or supplementary cereal.
Materials and methods
Materials
Triticale lines 2 (T2) and 5 (T5) were selected for the fermentation experiments from a set of eight elite lines, kindly provided by CIMMYT in the framework of its longstanding collaboration with ENEA. The selection was carried out after the evaluation of the agronomical performances of the whole set of triticale lines analyzed during three different field experiments in marginal Italian areas, in three seasons, in rainfed conditions, using a low nitrogen input [15, 16]. In the third season, the two lines were harvested as green biomass at the milk stage (i.e. when the grains start to be filled, after flowering, continuing for about 2 weeks and with a lactescent appearance) and then, later on at the complete maturity stage, when the grains fill the spikes.
A waxy line of bread wheat, EW9, kindly provided by Società Produttori Sementi (Bologna, IT), was also included in the field experiments and used for comparison in the fermentation from seeds experiment.
Green biomass characterization
The aerial part of T2 plants was collected at the milk stage. A representative sample was air-dried and stored, following the National Renewable Energy Laboratory (NREL) standard procedure [17]. The total solids, ash contents, and compositional analyses were performed following the NREL standard procedures [18–20].
Carbohydrate analysis
The carbohydrate content of the untreated and pre-treated feedstocks and hydrolysates from enzymatic hydrolysis was determined by measuring the monomeric sugars. Structural carbohydrate analysis was carried out using the following procedure [20]: triplicate samples of 300.0 mg ± 10.0 mg were placed into tared pressure tubes with 3 mL ± 0.01 mL of 72% sulfuric acid, incubated in a water bath set at 30°C ± 3°C for 60 min ± 5 min and stirred every ten minutes without removing the sample from the bath. Upon completion of the 60 min hydrolysis, the acid was diluted to 4% by adding 84.00 mL ± 0.04 mL deionized water using an automatic burette. A set of sugar recovery standards (SRS) was used to correct for losses due to the destruction of sugars during dilute acid hydrolysis. SRS include D-(+)-glucose, D-(+)-xylose, D-(+)-galactose, L-(+)-arabinose, and D-(+)-mannose. SRS sugar concentrations were chosen to most closely resemble the concentrations of sugars in the test sample. Triticale biomass samples and SRS were autoclaved for one hour at 121°C. After completion of the autoclave cycle, the samples were slowly cooled to near room temperature. A series of calibration standards was prepared in the following concentration range (mg/mL): D-cellobiose 0.1–4.0; D-(+)-glucose 0.1–4.0; D-(+)-xylose 0.1–4.0; D-(+)-galactose 0.1–4.0; L-(+)-arabinose 0.1–4.0; D-(+)-mannose 0.1–4.0. Calcium carbonate was used to neutralize each sample to pH 5–6. The sample for analysis was prepared by passing the decanted liquid through a 0.2 µm filter into an autosampler vial. Calibration standards and samples were quantified by high performance liquid chromatography (HPLC; Series 200 system, Perkin Elmer, USA), equipped with a quaternary gradient pump, a refractive index detector, and a Perkin Elmer Chem-station (software Turbochrom, v6.1.1. 2000). An ionic exclusion monosaccharide Pb2+ chromatographic column, Repromer Pb (repro-Gel; 300 mm × 7.8 mm, 9 μm; Dr. Maisch, Germany), and the corresponding guard column (4 mm × 50 mm; Dr. Maisch, Germany) were used for chromatographic separation. The following operating parameters were applied: injection volume: 10 µL; mobile phase: HPLC grade water, 0.2 µm filtered and degassed; flow rate: 0.6 mL/min; column temperature: 80°C; run time: 35 min. HPLC analyses were carried out in triplicate.
Seed characterization
Grain yields (GYs) and yield-related traits were measured in T5 and EW9 line plants harvested at maturity. The thousand kernel weight (TKW) was calculated as the mean weight of 1,000 grains according to the method ISO 520:2010 [21] and the results were expressed as grams. The test weight (TW) was measured with a shopper chondrometer (Nilema-litre, Chopin Technologies, France) equipped with a 1 L container and the results were expressed as kg/hL following the ISO 7971-1:2009 method [22]. Kernel hardness was determined using the Perten Single Kernel Characterization System (SKCS) 4100 (Springfield, IL, USA) in line with the manufacturer’s operating procedures. The instrument was set in a range of hardness values between –40 and +120, and the SKCS index was recorded as the average of 300 kernels. The grains were ground by a laboratory mill (Cyclotec, FOSS, Denmark) equipped with a 0.5 mm sieve prior to the other analyses. Moisture content was analyzed with a thermo balance (Sartorius MA 40, Sartorius, Germany) at 120°C. Protein content was determined by a Kjeldhal nitrogen analysis (N × 5.7) and was expressed as a percentage on a dry weight basis (ICC 105/2). The α- and β-amylase activities, particularly relevant for seed fermentation, were determined using the Ceralpha assay kit (Megazyme, Ireland) [23] and the Betamyl assay kit (Megazyme, Ireland) [24], respectively. The starch content was measured using Ewers polarimetric method ISO 10520 [25].
Yeast strains
Two different yeast strains were used in the fermentation of hay and seeds:
Hay fermentation: Saccharomyces cerevisiae M861 (ENEA patent, Italian number 1206039), able to resist high ethanol concentrations, was used. The yeast was revitalized from a frozen stock, enriched on potato dextrose agar (PDA) plates, and finally used to inoculate 1.5 L 2% glucose yeast extract peptone dextrose (YEPD). This broth was incubated in an orbital shaker at 30°C, 130 rpm, for 18 h. After the evaluation of cell numbers in a Neubauer counting chamber, the right volume of inoculum was centrifuged and directly resuspended in order to have a final concentration of 109 cell/mL in each sample.
Seed fermentation: Baker’s yeast S. cerevisiae (AB Mauri, Italy) was used to prepare the inoculum. The yeast was suspended in 0.1% sterile peptone water and incubated at 30°C, 140 rpm, overnight. The culture growth was diluted and monitored with a biophotometer at OD600 and the final cell number was counted in a Neubauer counting chamber. The final yeast solution was adjusted in order to obtain 38 × 106 colony forming units (CFU)/mL in the fermentation bottles.
Procedure for obtaining bioethanol from triticale hay fermentation
Green biomass was used to produce bioethanol by applying a separate hydrolysis and fermentation (SHF) protocol, in accordance with the Chen et al. [26] procedure and taking into account their final results and conclusions.
Generally speaking, lignocellulose consists of cellulose (C6 sugar polymers), hemicellulose (C5 sugar polymers), and lignin (aromatic alcohol polymers). In SHF, hydrolysis and fermentation take place in separate bioreactors, each one at their optimal temperatures (50°C ± 5°C for hydrolysis and 28°–32°C for yeast fermentation). A pre-treatment is first applied to the raw material to separate the different elements and increase accessibility to enzymes for hydrolysis. Once the cellulose and the hemi-cellulose are separated from the lignin, the solid phase is separated from the liquid phase and saccharification is carried out by the addition of cellulolytic enzymes (enzymatic hydrolysis). After the enzymatic hydrolysis, hydrolysate is added with yeast for fermentation.
Feedstock samples consisted of the T2 line harvested at the milk stage, air-dried to a moisture content < 10%, and milled and sieved (1 mm screen) [17]. Three samples (20 g each) were pre-treated with 2.0% NaOH (w/v) and a substrate solid loading of 10% (w/v, dry weight basis) in an autoclave at 121°C and 15 psi pressure for 60 min. The pre-treated solids were washed with 1.5 L of hot deionized water and stored at 4°C before enzymatic hydrolysis; a fraction was used for evaluating the effect of chemical treatment on feedstock composition.
The enzymatic hydrolysis was performed in 250 mL Erlenmeyer flasks. Wet, pre-treated samples containing 1 g of dry sample (as determined by moisture analysis) were mixed with 30 mL citrate buffer (pH 4.8, 50 mM) containing 40 μg/mL tetracycline hydrochloride, to avoid microbial contamination. Sample flasks were preincubated in a shaking water bath at 55°C and 150 rpm for 10 min before the addition of the enzymes.
Cellulase from Trichoderma reesei, Celluclast 1.5 L (C2730, Sigma-Aldrich, USA) with an activity of 700 U/g, and cellobiase from Aspergillus niger (C6105, Sigma-Aldrich, USA), 254 U/g, were used for enzymatic hydrolysis. Excess cellobiase (β glucosidase) was added to Celluclast 1.5 L to avoid end-product inhibition due to cellobiose build-up [27]. The two enzymes were mixed at a ratio of 1:1.75 (v/v) and used for hydrolysis experiments at a cellulase activity of 40 filter paper activity (FPU)/g glucan. Control samples, without enzymes added, were also included to determine the background sugar concentrations in the pre-treated feedstocks. The samples were incubated in a shaking incubator at 55°C and 150 rpm for 72 h. After the enzymatic hydrolysis, hydrolysates were sampled and samples (2 mL) were immediately chilled on ice, centrifuged at 5,000× g for 10 min, and analyzed for fermentable sugar content. Supernatants (20 mL) were transferred to 100 mL serum bottles for fermentation and inoculated with S. cerevisiae M861 at a cell concentration of 10 g dry matter/L. All samples were then incubated at 30°C ± 1°C for 24 h and analyzed for ethanol content after fermentation.
Ethanol content in the fermentation broth was analyzed using HPLC [28]. All samples were centrifuged at 5,000× g for 10 min before analysis.
The ethanol yield was calculated as a percentage of the theoretical maximum ethanol yield (Ymax) as per Equation 1 [29].
where E and G represent Ethanol (g) produced during fermentation and Glucose (g) in the hydrolysates, respectively. The constant 0.511 is the theoretical yield of ethanol produced from glucose.
Procedure for obtaining bioethanol from triticale seeds
T5 seeds were used to produce bioethanol by applying a simultaneous saccharification and fermentation (SSF) protocol and a very high gravity (VHG) fermentation technology, in accordance with the Pejin et al. [30] procedure and taking into account their final results and conclusions. As triticale exhibits a high auto-amylolytic enzyme activity, the liquefaction/saccharification phases were carried out without using any additional saccharifying enzymes, and starch was hydrolyzed using only the endogenous enzymes. Bread wheat EW9 line was also used, for comparison. A time course experiment was designed based on five times (15 h, 24 h, 39 h, 48 h, and 72 h), with triplicate bottles for each time. Milled samples (25 g each) were dispersed into a water solution of calcium chloride pre-warmed to 60°C (sample to solution ratio 1:3, final calcium ion concentration 100 mg/L) in closed bottles kept with constant agitation in a shaking water bath (Heto SBD50, Heto, USA) at 60°C for 65 min to perform liquefaction. After the liquefaction, the samples were air cooled to 20°C. Subsequently, a magnesium sulfate solution (final magnesium ion concentration 160 mg/L) and the yeast inoculum were added to the samples and were fermented at 30°C. Single controls were carried out for each line. A control was prepared at T = 0, without adding inoculum, to check the liquefaction efficiency and the absence of ethanol and another control was carried out up to T = 72 h, without adding inoculum, to check the absence of ethanol. The bottles were equipped with water traps to prevent air from entering and allow carbon dioxide produced during the fermentation to escape. After fermentation, the fermented samples were centrifuged for 15 min (8,000 r/min at 10°C) in a refrigerated centrifuge (Sorvall RC 5, Sorvall, USA) and the supernatants were analyzed [European Brewery Convention (EBC), Analytica EBC Method 9.2.1 and Analytica EBC method 9.4] to determinate the bioethanol and the real extract contents by measuring the density at 20°C of the bioethanol distillate (with a digital Mettler Toledo DR-45 density meter, Mettler Toledo, USA) and of the distillation residue (obtained with a SDEE Digital Laboratory Distiller, Gibertini, Italy). The results were expressed as % w/w and % v/v and the standard deviation was also calculated.
Data analysis
IBM SPSS Statistics 23.0 was used for the analysis of variance (one-way ANOVA). Means were considered statistically different at a 95% confidence level.
Results
Bioethanol from triticale hay fermentation
As known, lignocellulosic biomass contains 43% cellulose, 20% hemicellulose, 27% lignin, and 10% other components, on average [13]. Cellulose is a polysaccharide consisting of a linear chain of several D-glucose units, while hemicelluloses are branched polysaccharides composed of diverse sugars, such as xylose and arabinose and also glucose, mannose, and galactose. The raw material from the T2 line was characterized for its main constituents by measuring the monomeric sugars following NREL procedure [20] and the results are shown in Table 1. Glucose and xylose represented the major carbohydrate components, arabinose and galactose accounting for only a small portion.
Compositional analyses of feedstock as raw material and after the chemical pre-treatment※
Raw material compositional analysis | Compositional analysis after alkaline pre-treatment | |||||||||
---|---|---|---|---|---|---|---|---|---|---|
Feedstock | % Total solids | % Ash | % ASL | Arabinose※※ | Galactose※※ | Glucose※※ | Xylose※※ | Arabinose※※ | Glucose※※ | Xylose※※ |
T2 | 89.10(0.20) | 5.26(0.16) | 1.49(0.06) | 1.77(0.01) | 0.71(0.01) | 30.77(0.05) | 14.65(0.03) | 3.17(0.40) | 66.08(1.39) | 26.97(0.79) |
※ Values in brackets are standard deviations; ※※ values are expressed as w/w referred to 100 gr of dry matter and were means of three samples. T2: triticale line 2; ASL: acid-soluble lignin
The first step of the SHF protocol was the alkaline pre-treatment of the biomass, aimed at breaking the H-bonding in cellulose and hemicellulose, the ester bonds cross-linking lignin and xylan, thus increasing the porosity and cellulose digestibility, and proving effective in lignin solubilization. The effect of this chemical pre-treatment on feedstock composition is shown in Table 1.
After the alkaline pre-treatment, the wet biomass was enzymatically saccharified and the supernatant recovered was analyzed to evaluate the process efficiency. The glucan conversion percentage was calculated as the ratio between glucose in the supernatant and glucan in the pre-treated samples (calculated applying 0.90 anhydro correction [20] on the glucose concentration). These values are reported in Table 2, in which the results of the fermentation are also shown.
Percentage of glucan conversions and fermentation of hydrolysate from enzymatic hydrolysis of feedstocks※
Feedstock | Glucose in the hydrolysate (g)※※ | Glucan conversion (%) | Ethanol yield(g/g sugar) | Maximum ethanol yield (%)※※※ |
---|---|---|---|---|
T2 | 0.29 (0.02) | 15.58 (0.04) | 0.31 (0.10) | 61.70 (3.29) |
※ Values were means of three samples, values in brackets are standard deviations; ※※ indicates total glucose available for fermentation; ※※※ ethanol yield calculated as a percentage of theoretical maximum ethanol yield (Ymax, Equation 1). T2: triticale line 2
Bioethanol from seed fermentation
The agronomical and other industrial parameters of the T5 and EW9 lines are shown in Table 3. The parameters relating to the evaluation of the endogenous amylolytic activity (starch content and α- and β-amylases) were particularly important, in view of the fermentation.
Agronomic traits and technological quality of the two lines analyzed※
Feedstock | GY (ton/ha) | TW (kg/hL) | TKW (g) | Moisture (%) | Protein(% dw) | Gluten(% dw) | SKCS index | α (CU/g) | β (B3U/g) | Starch(% dw) |
---|---|---|---|---|---|---|---|---|---|---|
T5 | 2.33(0.28) | 68.60(1.70) | 47.50(0.42) | 17.65(0.64) | 11.50(0.14) | 8.65(0.21) | 63.50(3.54) | 1.42(0.67) | 19.36(1.46) | 64.70(0.45) |
EW9 | 1.93(0.21) | 71.60(0.57) | 46.15(2.05) | 18.15(0.49) | 10.95(0.49) | 9.70(0.28) | 40.00(1.41) | 1.58(0.45) | 19.80(1.59) | 72.31(0.44) |
※ Values were means of three samples; values in brackets are standard deviations. α: units of α-amylase/g flour; β: units of β-amylase/g flour. GY: grain yield; TW: test weight; TKW: thousand kernel weight; dw: dry weight; SKCS: Single Kernel Characterization System; T5: triticale line 5
Table S1 shows the average values and standard deviations of the ethanol produced from the various samples analyzed during the fermentation process, expressed as % volume and % mass. Values of real extract, a parameter directly related to the quantity of sugars still present in the samples after distillation, were also included.
In Figure 1, the ethanol production during the time course experiment is shown, each point corresponding to the mean value of three samples.
The ethanol production of T5 grew sharply and rapidly from time T = 0 to time T = 15 h and then increased continuously suggesting a plateau after 72 h. The whole trend was significantly higher in comparison with EW9 ethanol production (P < 0.001).
In Figure 2, the real extract values are represented at each time, calculated on the basis of the quantity of sugars present in the distillation residue of each sample. Each point in Figure 2 corresponds to the mean value of three samples. As expected, the curves showed an opposite trend to the ethanol production ones. At time T = 0, when all sugars available had not been fermented, the value was highest and then decreased continuously during the ethanol production.
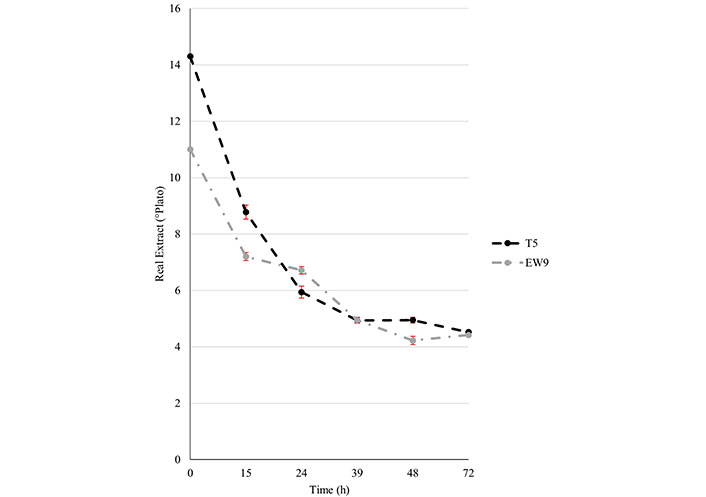
Real extract values measured during the time course fermentation experiment. T5: triticale line 5
The real extract values were also found to be significantly different (P < 0.01) at a lower level and overlapped at 39 h and 72 h.
The dry weight of the feedstocks and the obtained amount of ethanol were used to calculate the ethanol yields. These results are shown in Table 4.
Calculated ethanol yields※
Feedstock | ton grains/ton ethanol | L ethanol/ton grains |
---|---|---|
T5 | 3.04 | 416 |
EW9 | 4.95 | 258 |
※ Values refer to dry weight (dw). T5: triticale line 5
Discussion
Bioethanol from triticale hay fermentation
The protocol used came from the results of Chen et al. [26] as regards the choice of a basic pre-treatment, the concentration of sodium hydroxide, and the enzymes loading. The procedure appeared very challenging and required some adjustment, mainly as regards the quantification of intermediates, which is necessary to calculate the yield. In line with suggestions for the preparation of samples [17], we used lyophilization to calculate the percentage of total solids starting from a wet pre-treated sample of slurry. This value was used to carry out enzymatic hydrolysis and in subsequent calculations. The composition of the feedstock used in this study (Table 1) is comparable to the values reported by Chen et al. [26] and the effect of the pre-treatment was a comparable composition of sugars (Table 1). Many factors may have negatively influenced the efficiency of the enzymatic hydrolysis, which resulted in only 15.58% conversion of glucan (Table 2). Hemicellulose and cellulose hydrolysis is considered one of the major drawbacks in the overall process of bioethanol production from lignocellulosic biomass [8]. Even if the pre-treated wet sample had the expected composition [26], it could be possible that it was not completely swelled, thus affecting the digestibility of the cellulose in the subsequent enzymatic hydrolysis. It was also possible that the enzymes (cellulase and β glucosidase) were not completely able to operate, since they were inhibited by the end products (cellobiose and glucose), and the build-up of any of these products negatively affects cellulose hydrolysis [27]. Nevertheless, the ethanol yield obtained (0.31 g/g sugar) is comparable to that reported by Chen et al. [26] (0.34 g/g sugar), even if we could only use the result of fermentation after 24 h, because after 48 h the samples analyzed were found to be contaminated. A further analysis allowed us to relate ethanol yield to dry biomass and inferred that 5.42 tons of dry weight biomass produced one ton of ethanol.
Bioethanol from seed fermentation
The comparison with data from Pejin et al. [30] shows that our experimental apparatus and the protocol used were able to obtain reliable data in line with theirs. It is noteworthy that our values were measured at 72 h of fermentation using Ca++ and Mg++ solutions simultaneously, while in Pejin et al. [30], the fermentation lasted up to 96 h but the ions solutions were tested separately. Overall, our results at 72 h suggest a possible synergic action in the simultaneous use of Ca++ and Mg++ solutions.
Our results highlight the different behavior in ethanol production between the T5 and EW9 lines.
The T5 line showed a more efficient fermentation kinetics, with a sharp curve between 15 h and 24 h, reaching 84% of the total alcohol obtained at the end of the experiment (considering ethanol grams per 100 g dry weight material). On the other hand, the EW9 line showed a much slower trend with a notable decline at 24 h (only + 0.45% in 9 h), reaching about 61% in comparison with T5 at 72 h (considering ethanol grams per 100 g dry weight material). It is worth noting that the quantity of sugars available for fermentation also seemed to be significantly lower in the EW9 than in the T5 lines (–3.3°Plato, see Table S1) at the beginning of the experiment, suggesting a lack of efficiency during the liquefaction step, possibly due to lower endogenous amylase enzymes activity and to sub-optimal fermentation conditions, although EW9 is a waxy bread wheat line (see Table 3). In addition, fermentation kinetics was lower, suggesting some susceptibility of the used yeast strain to the wort derived from the EW9 line. However, it should be underlined for both lines that the residual fermentable sugars were not negligible. Different strategies can therefore be hypothesized to improve the efficiency of this system, such as the use of a different yeast strain, more resistant to the alcohol content, the setting of a higher temperature, or a lengthening of the liquefaction time.
The comparison of our ethanol yields (Table 4) with data found in the literature shows them to be similar for triticale. Klikocka et al. [11] reported a mean theoretical ethanol efficiency of 479.5 L/ton and, citing literature data, reported that 1,533 L were produced by 4.60 tons of triticale and 1,880 L were produced by 5.64 tons of winter wheat [31]. Furthermore, Smith et al. [32] reported data from the literature that estimated an average value of 441 L/ton for triticale. The comparison with wheat data confirms that ethanol yields from the EW9 line are in fact lower than expected for wheat.
A comparison between the ethanol yields produced with our two feedstocks, lignocellulosic biomass and grains, highlights the higher efficiency of the fermentation protocol applied to grains (3.04 ton grains/ton ethanol), compared to the procedure used for hay (5.42 ton dry weight biomass/ton ethanol). As is generally known, the use of lignocellulosic materials for producing bioethanol is an interesting alternative to starch sources but the process is challenging and still expensive, despite the huge interest in it and substantial progress in research and development. It requires a pre-treatment phase, the use of added enzymes, relatively expensive, microbial fermentation of the simple sugars to ethanol, as well as a separation and purification step, each one affecting the final bioethanol yield [33].
Our experimental work on ethanol production from triticale biomass was found to be reliable and gave very interesting results with a good cost/benefit ratio, taking into account not least that results from triticale grains were obtained without additional costs for enzyme supplementation. The question of sustainability is addressed by the cultivation on marginal land, and the use of low-input farming systems also contributes to lower pollution and cost. These results therefore point to new potential industrial applications of triticale crops, mainly in Italy.
In particular, our results together with the high activity of the endogenous amylolytic enzymes, mainly α-amylase, and the high starch content suggest that the triticale crop could be considered a new candidate for beer production, either as the main or supplementary cereal. It is worth noting that as a result, this crop, initially constituted for food, subsequently used for feed production, and then considered industrially for bio-alcohol production, would thus come back to an agri-food application in the form of human consumption of a new beverage, i.e. in beer production. In today’s brewing industry, supplements such as corn, rice, and sorghum are used to reduce production costs, providing a greater starchy substrate than barley and at a lower price. Triticale could represent a valuable new option either as the main or supplementary cereal and preliminary results obtained in a micro-scale laboratory look interesting. We are therefore planning to set up an optimal protocol and to involve a craft brewery in conducting experimental production.
Abbreviations
CIMMYT: | Centro Internacional de Mejoramiento de Maíz y Trigo |
EBC: | European Brewery Convention |
ENEA: | Italian National Agency for New Technologies, Energy and Sustainable Economic Development |
EU: | European Union |
HPLC: | high performance liquid chromatography |
NREL: | National Renewable Energy Laboratory |
SHF: | separate hydrolysis and fermentation |
SRS: | sugar recovery standards |
T2: | triticale line 2 |
Supplementary materials
The supplementary table for this article is available at: https://www.explorationpub.com/uploads/Article/file/101054_sup_1.pdf.
Declarations
Acknowledgments
We dedicate this paper to the memory of our ENEA colleague Tiziana Coccioletti, who gave her highly professional and human contribution to this work. The authors thank Dr. A. Massi (Società Produttori Sementi, Bologna, IT) for providing seeds of the waxy bread wheat EW9 line; Dr. A. Sprocati (ENEA) for providing the S. cerevisiae M861 strain; Dr. G. Marconi (ENEA library service) for his constant assistance and Mr. Ian Pace for reviewing the English of the text. The authors thank the non-profit organization AaIS (Bracciano IT) for providing field areas and technical assistance during the field experiments. The authors are grateful to Dr. Claudia Zoani (ENEA) for her encouragement to submit the current manuscript.
Author contributions
PG: Conceptualization, Methodology, Investigation, Data curation, Writing—original draft, Writing—review & editing, Supervision. KA: Conceptualization, Methodology, Investigation, Writing—review & editing. CC: Methodology, Investigation, Data curation, Writing—original draft, Writing—review & editing. AL: Methodology, Investigation, Writing—review & editing. LG, FN, GM, O Maccioni, O Marconi, GDF, and SF: Investigation, Writing—review & editing. All authors read and approved the submitted version. All authors approved the current manuscript.
Conflicts of interest
The authors declare that they have no conflicts of interest.
Ethical approval
Not applicable.
Consent to participate
Not applicable.
Consent to publication
Not applicable.
Availability of data and materials
The raw data supporting the conclusions of this manuscript will be made available upon request from the corresponding author (patriziagaleffi@gmail.com), without undue reservation, to any qualified researchers.
Funding
Not applicable.
Copyright
© The Author(s) 2024.