Abstract
Aim:
The scope of the present study was to elaborate a nitrite electrochemical sensor using multi-walled carbon nanotubes (MWCNTs) modified Pt electrode.
Methods:
The electrocatalytic activity of the proposed sensor was studied by cyclic voltammetry (CV), chronoamperometry (ChAm), and electrochemical impedance spectroscopy (EIS). Then electrochemical detection of nitrite was performed using square wave voltammetry (SWV).
Results:
The MWCNTs/Pt electrode exhibits good electrocatalytic activity for the oxidation of nitrite in neutral pH. Subsequently, the fabricated electrochemical sensor shows a high sensitivity for the NO2– determination and a low limit of detection (LOD) (0.1 µM) in a wide linear concentration range (1.0–1,000 µM).
Conclusions:
This electrochemical sensor has cost-effective, anti-interference capability as well as good stability, reproducibility, and applicability.
Keywords
Multi-walled carbon nanotubes, modified electrode, nitrite ion, electrochemical sensorIntroduction
Nitrite is widely present in daily life and the natural environment, but its overuse and potential toxicity is a serious health threat. It reacts with the blood pigments to form meta-hemoglobin, resulting in oxygen depletion in the tissue and the excess quantity in the blood produces an irreversible oxidation of the hemoglobin [1]. Moreover, the gastric reaction of NO2– with amines and amides generates N-nitrosamine compounds, suspected to be carcinogens [2]. According to World Health Organization (WHO) standards, the maximum allowable content of NO2– in drinking water is 43.48 μM [3]. Therefore, it is imperative to develop sensitive strategies for the detection of NO2– in drinking water and food. In this respect, certain techniques such as spectrophotometry [4], chromatography [5], polarography [6], electrophoresis [7], and flow injection analysis [8] were used for this purpose. Nevertheless, it should be emphasized that these methods involve long analysis times, energy costs, and complicated procedures. Among the suggested techniques, electrochemistry remains an efficient alternative owing to its simplicity and fast response to the operation modes [9–12]. However, it is still a challenge to improve the sensitivity and other properties of the electrochemical nitrite sensors.
In order to improve the electrochemical sensing properties, great efforts have been focused on electrochemical sensors by modifying the electrode with electron transfer mediators. In the last few years, multi-walled carbon nanotubes (MWCNTs) have attracted enormous interest because of their unique structural, mechanical, and electronic properties. Some of these properties include high chemical and thermal stability, high elasticity, high tensile strength, and in some instances, metallic conductivity [13, 14]. The modification of electrode substrates with MWCNT for use in analytical sensing is well documented to result in low limit of detections (LODs), increased sensitivities, reduced overpotentials, and resistance to surface fouling [15, 16]. The best solvents reported for effecting CNT dispersions are N,N-dimethylformamide (DMF) and N-methylpyrrolidone [17]. Further, several strategies were used to build CNT thin films by the layer-by-layer method and the dispersion without chemical functionalization of CNTs on the electrode surface [18, 19].
In the current work, we propose a sensitive and low-cost technique for the analysis of NO2– in an aqueous environment using MWCNTs coated Pt electrode. The proposed sensor was characterized by cyclic voltammetry (CV), electrochemical impedance spectroscopy (EIS), and chronoamperometry (ChAm) methods. The oxidation of nitrite ion was investigated with square wave voltammetry (SWV). In particular, the oxidation of nitrite was found at less positive potentials proving the catalytical effect of MWCNTs/Pt electrode. The effect of possible interferents was examined using 100-fold concentrated solutions of mineral and organic substances. The analytical applicability of the electrode was demonstrated in mineral and source water samples with a high reliability. To the best of our knowledge, this is the first time we have determined nitrite ion using MWCNTs coated Pt electrode. Such an electrode system provided a non-enzymatic sensing platform with good electrocatalytic properties and sensitivity for nitrite detection, a fast response time, and low-cost analysis.
Materials and methods
Reagents and materials
MWCNTs were supplied from DropSens, HCON(CH3)2 (68%, Sigma-Aldrich), and NaNO2 (98%, Fluka) were of analytical quality. The phosphate-buffered solution (PBS) was elaborated by mixing 0.1 M NaH2PO4 and Na2HPO4 solutions (98%, Sigma-Aldrich). Deionized water was employed for all preparations.
The electrochemical study was carried out at room temperature in a three-electrode arrangement in the standard cell; the data were collected with a potentiostat/galvanostat (model PGZ402, Radiometer Analytical, France) coupled with a PC-computer. A saturated calomel electrode (SCE) (Hg/Hg2Cl2) as a reference, Pt wire as an auxiliary electrode, and MWCNTs/Pt as a working electrode (Ø = 2 mm) were used on the electrochemical cell. The pH was measured with a digital pH meter (model inoLab® pH 7110).
Preparation of modified electrode
The working electrode was mechanically polished to a mirror-like surface with Al2O3 (0.3 µm) slurry on a polishing cloth. After being washed with distilled water, the bare electrode was sonicated (5 min) in ethanol and dried at ambient temperature. A suspension was prepared by dispersing 1 mg MWCNTs in 1 mL DMF under sonication for 30 min. The MWCNT-modified Pt was prepared by casting a known volume (3 µL) of the mentioned suspension on the Pt electrode surface using a micropipette and left to dry at room temperature. The surface was electrochemically cleaned in supporting electrolyte by CV between –0.25 V and 1.5 V for several cycles after each run to remove the adsorbed substances. Then the electrode surface was rinsed with distilled water.
Analytical procedure
MWCNTs/GCE (glassy carbon electrode) was electrochemically activated in PBS solution (0.1 M, pH 7.0) under scanning potential between –0.25 and 1.2 V at 50 mVs–1 until a reproducible cyclic voltammogram was obtained. The sensor was transferred into a 10 mL glass standard cell filled with PBS solution, and aliquots of the stock solution were added. The calibration graph was constructed from the SW voltammograms plotted by successive additions from aliquots of the stock solution to 10 mL of PBS solution (0.1 M, pH 7.0); each concentration was measured in triplicate. All potential were referred to the SCE at an ambient temperature.
Results
Electrooxidation of nitrite on MWCNTs/Pt electrode
Figure 1a illustrates the voltammograms of the bare electrode and MWCNTs/Pt in 0.1 M PBS (pH 7.0) in the absence and the presence of 1.0 mM NO2– at a scan rate of 50 mVs–1. As expected, no signal could appear on the bare Pt and modified electrode in pure 0.1 M PBS at pH 7.0. However, in the addition of 1.0 mM NO2–, an oxidation peak appears at 0.70 V and no reduction peak appears in the reversal scan. The cyclic voltammogram of 1.0 mM NO2– at the bare Pt under identical conditions is given for comparison. As shown, the oxidation peak is obtained at about 0.77 V, and no reduction peak appears in the reversal scan.
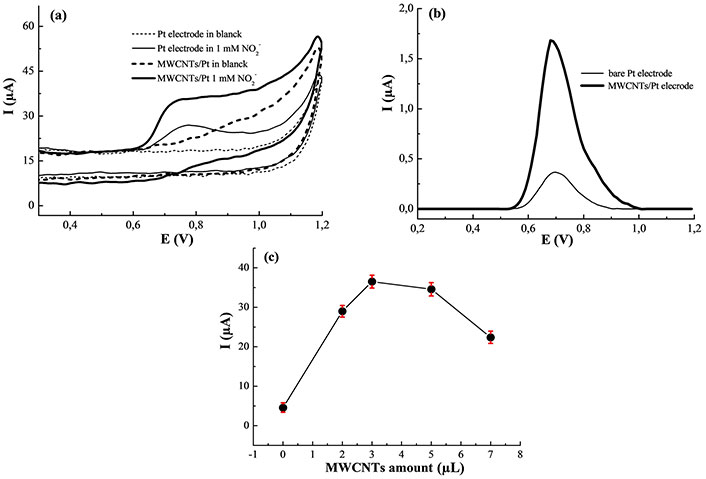
Electrochemical behavior. (a) Cyclic voltammograms of the bare Pt and MWCNTs/Pt in the absence and presence of 1.0 mM NO2– in 0.1 M PBS at pH 7.0; (b) SWV response of the bare Pt and MWCNTs/Pt with 1.0 mM NO2– in 0.1 M PBS at pH 7.0; (c) effect of the MWCNTs amount on the current response of 1 mM NO2–. MWCNTs: multi-walled carbon nanotubes
In addition, when the obtained SWV results were compared (Figure 1b), the MWCNTs/Pt shows a higher response current, which presents an increase of more than four times in the voltammetric response compared to the bare Pt electrode. This indicates that the MWCNTs/Pt improves the electrochemical reaction of NO2– compared with bare Pt electrode. The enhanced signal is probably due to the facile electron transfer with an improved rate and larger active surface area of the modified electrode.
The effect of MWCNT amounts on the peak current response of 1 mM NO2– was examined (Figure 1c). In this regard, different volumes of the suspension were cast on the bare Pt. The best response of NO2– was obtained when a 3 μL drop was used. Therefore, the amount of MWCNTs chosen for the preparation of the sensor was fixed at 3 μL.
Electrochemical characterization of MWCNTs/Pt
The cyclic voltammograms of bare Pt and MWCNTs/Pt electrodes were carried out in 1.0 mM [Fe(CN)6]3–/4– containing 0.1 M KCl at a scan rate of 50 mVs–1 (Figure 2a). A pair of redox peaks were observed at both unmodified and modified electrodes. The peak current at the modified electrode increases dramatically compared with that observed at the bare Pt electrode, suggesting that MWCNTs/Pt exhibits faster electron transfer kinetics compared with the bare Pt electrode. Using the Randles-Sevcik relation [20]:
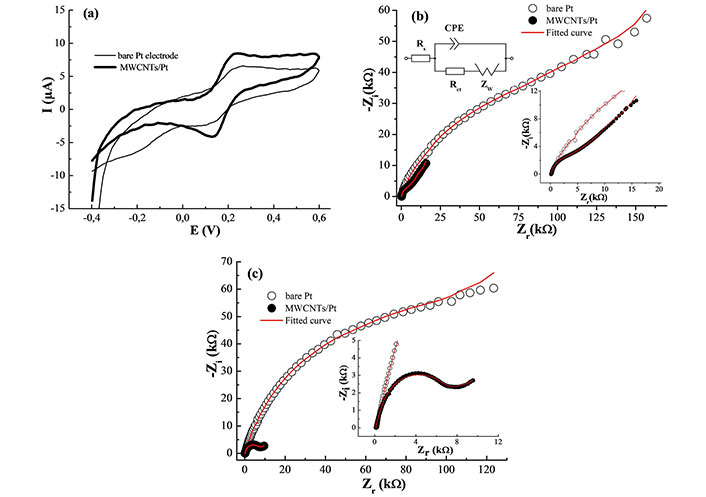
Electrochemical characterization. (a) Cyclic voltammograms; (b) Nyquist plots of bare Pt and MWCNTs/Pt in 0.1 M KCl containing 1.0 mM K3[Fe(CN)6]; (c) Nyquist plots of bare Pt and MWCNTs/Pt in 0.1 M PBS (pH = 7.0) containing 1 mM NO2–. Inset (b): Randles electrical circuit of the redox system. CPE: constant phase element
Where n, Co, A, v, and Do are the number of electrons involved, the substrate concentration (1.0 mM), the active surface area, the scan rate, and the diffusion coefficient of ferrocenium/ferrocene (7.6 × 10–6 cm/s [21]) respectively. The surface area of MWCNTs/Pt and bare Pt electrode are respectively 0.042 and 0.035 cm2. The results clearly show that the electrode modified with MWCNTs film has a larger active surface area, which results in higher sensitivity.
EIS analysis of the bare Pt and MWCNTs/Pt was performed to explain the electron transfer changes between the modified electrode and the solution interface in the presence of 10 mM [Fe(CN)6]3–/4– containing 0.1 M KCl. In the EIS plots (Figure 2b), the high-frequency region of the impedance real part presents the solution resistance (Rs). The semicircular part at high frequency illustrates the electron transfer resistance (Rct) while the straight line at the low-frequency ranges is ascribed to the diffusion of K3Fe(CN)6/K4Fe(CN)6 as the redox probe (Warburg impedance, W) [22–25]. Moreover, a constant phase element (CPE) is introduced to take into account the topological imperfections of the materials caused by the surface roughness of the electrode. The impedance (ZCPE) is characterized by a slight deviation from pure capacitive behavior and is then given by [22, 23]:
Where j = (–1)1/2, ω = 2πf being f the frequency of the applied ac potential,
EIS parameters obtained from the equivalent circuit proposed in Figure 2b
Electrode | Rs, kΩ | Rct, kΩ | Q, µFsα–1 | W, kΩ | α |
---|---|---|---|---|---|
Bare Pt | 166 | 50.70 | 2.55 | 597.8 | 0.70 |
MWCNTs/Pt | 135 | 4.27 | 14.20 | 41.37 | 0.86 |
MWCNTs: multi-walled carbon nanotubes
EIS was also used to investigate the charge transfer property of different electrodes in 0.1 M PBS (pH = 7.0) aqueous solution having 1 mM NO2– (Figure 2c). By fitting the Randles equivalent electrical circuits, the Rct caused by the faradaic reactions at the MWCNTs/electrolyte interface has a smaller semicircle diameter of 8.3 kΩ compared to 141.8 kΩ recorded for the bare Pt electrode. This feature suggests that MWCNTs improve the surface conductivity and hence the electron transfer kinetics of the analyte. Therefore, the MWCNTs/Pt-modified electrode was considered to be used in the application of nitrite electrochemical determination.
Scan rate study
The influence of scan rate on nitrite oxidation at MWCNTs/Pt was examined to investigate the kinetic mechanism of nitrite oxidation behavior. Figure 3a shows a cyclic voltammogram of the NO2– at various sweeping rates (5–300 mVs–1) in 0.1 M PBS solution at pH 7.0 using MWCNTs/Pt. The current responses are increased linearly with the square root of the scan rates (v1/2) (Figure 3b). This supports that the oxidation of NO2– is governed by the diffusion of the analyte at the MWCNTs/Pt electrode.
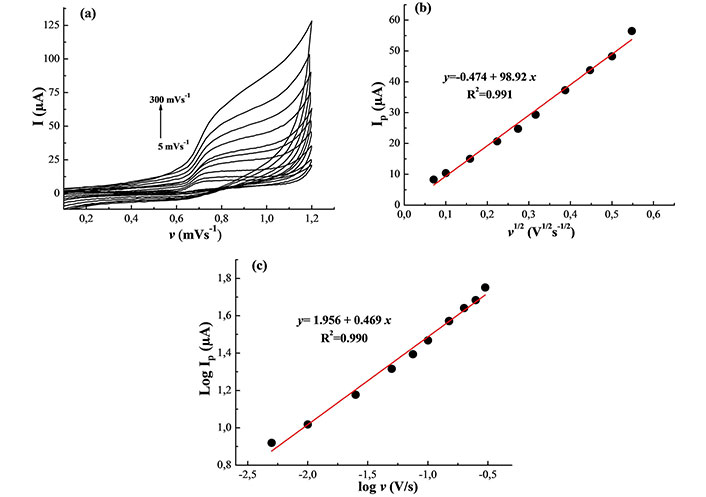
Kinetic of electrode process. (a) Cyclic voltammograms of the MWCNTs/Pt in 0.1 M PBS (pH 7.0) containing 1.0 mM NO2– at different scan rates (5–300 mVs–1); (b) linear relationship between anodic peak current and square root of scan rate; (c) linear relationship between log Ip and logarithmic scan rate
To support these results, the dependence of log Ip on log v was investigated (Figure 3c). Normally, the slope is close to 0.5 for a diffusion-controlled and 1.0 for an adsorption-controlled process [26]. The dependence of log Ip with log v for the nitrite oxidation peak gives a slope of 0.47 with a correlation coefficient close to unity (0.99), corroborating a diffusion-controlled electron transfer process of NO2– on the proposed sensor.
Therefore, the number of electrons (n) involved in the reaction can be extracted from the relation [27]:
Where Ep is the peak potential, and Ep/2 is the half-peak potential. Thus, αn was found to be 1.0. The coefficient α is equal to 0.5 for an irreversible system. The number of exchanged electrons in the NO2– oxidation is equal to 2.0, involving two electrons in the NO2– oxidation to NO3– in agreement with previous reports on nitrite oxidation [28–32]. Hence, the following reaction is suggested:
Effects of pH
Figure 4 depicts the current responses obtained at different pH values for the electro-oxidation of 1 mM NO2– on MWCNTs/Pt. As can be seen, the current response augments from pH 5.0 to 7.0, reaching a maximal pH of 7.0 and then decreases up to pH 9.0. Such results indicate that the pH domain is small in both acid and alkaline solutions, while the neutral pH is a suitable medium for the electro-catalytic oxidation of NO2– [32]. Hence, subsequent detection of NO2– was performed in PBS solution buffered at pH 7.0.
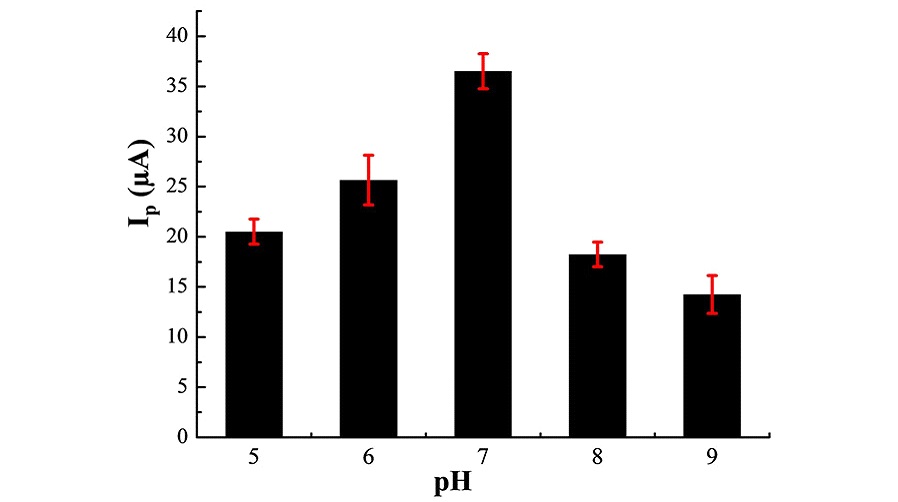
Voltammetric responses of the modified electrode at different pHs in 0.1 M PBS containing 1.0 mM NO2–
Chronoamperometric study
The electrocatalytic oxidation of NO2– on MWCNTs/Pt was also studied by ChAm in 0.1 M PBS (pH 7.0) having 10 µM NO2– at an applied potential of 0.7 V (Figure 5). Plotting the net current against t-1/2 exhibits a linear relation (Figure 5, insert a), with a dominant diffusion-controlled process. By using the slope of this plot, the diffusion coefficient (D) of NO2– can be extracted from the Cottrell relation [33]:
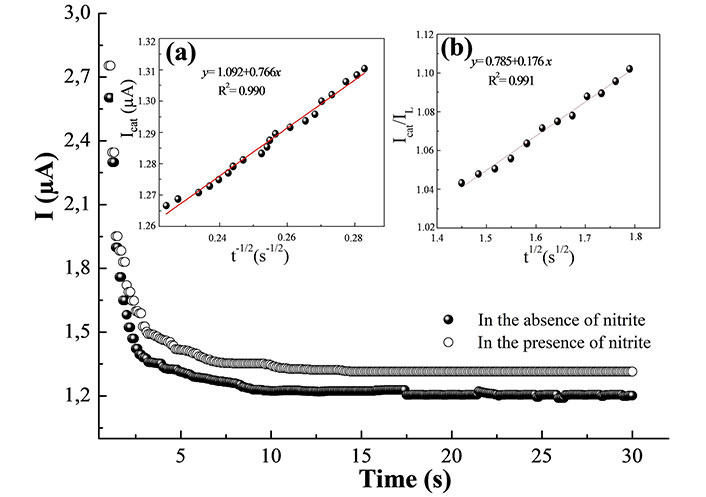
Chronoamperograms were recorded for MWCNTs/Pt in 0.1 M PBS (pH 7.0) in the absence and presence of 10 µM NO2–. Insert (a): dependence of Icat vs. t–1/2; insert (b): dependence of Icat/IL vs. t1/2
Where Co is the bulk concentration. The diffusion coefficient of NO2– is found to be 1.44 × 10–5 cm2/s which is close to formerly reported values [34, 35]. This technique was also used to estimate the catalytic rate constant (k) for the interface reaction between the nitrite and the modified electrode according to equation [36]:
Where Icat and IL are the amperometric currents of the MWCNTs/Pt with and without NO2– respectively, γ (= kCot) is the argument of the error function, and t is the elapsed time (s). When γ > 1.5, erf (γ1/2) is very close to 1 and the simplified equation becomes:
From the slope of the (Icat/IL) versus t1/2 plot (Figure 5, insert b), the value of k was obtained as 1.43 × 104 M–1s–1.
SWV detection of nitrite at MWCNTs/Pt
SWV is a pulse-sensitive method that could attenuate the background noise, allowing a higher sensitivity than the CV for low analyte concentrations [37]. SWV was performed in 0.1 M PBS (pH = 7.0) using a pulse duration time of 0.05 s, pulse amplitude of 25 mV, frequency of 50 Hz, a scan rate of 10 mVs–1, and a step potential of 10 mV. A well-defined nitrite oxidation peak is observed at 0.67 V as shown in Figure 6a. The peak current increases linearly with increasing the NO2– amount in the concentration ranges from 1.0 to 1,000 µM (Figure 6b). The LOD of the fabricated sensor is found as 0.1 µM taking LOD = 3 s/m, where s is the standard deviation of the current of low NO2– concentration and m is the slope of the calibration graph (n = 5) [38]. The LOD is well below the maximum allowable limit for NO2– in potable water (43.48 μM) according to the WHO directives [3]. The relative standard deviations (RSD) were evaluated at 1.5% for five separate voltammograms of 50 μM NO2–. Therefore, our sensor is better suited to assess NO2– concentrations in real water. The analytical parameters of the elaborated electrode are listed in Table 2. The electrocatalytic performance of MWNCNTs/Pt for the oxidation of NO2– was superior or comparable to previously reported results as displayed in Table 3. The improved sensing performance of MWCNTs/Pt can be attributed to their good conductivity and large surface area. These results for the MWCNTs/Pt system reveal a good analytical performance in terms of simplicity and efficiency for the detection of this analyte.
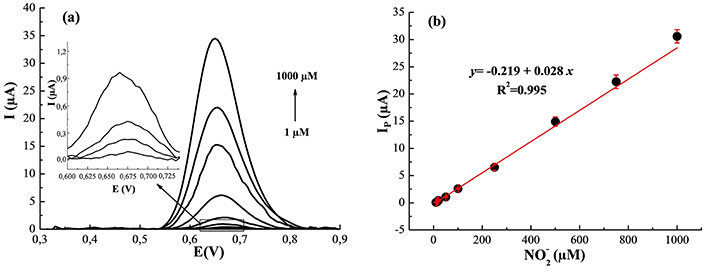
Electroanalytical performance. (a) SWV with varying concentrations (1–1,000 µM) of NO2– using the MWCNTs/Pt in 0.1 M PBS at pH 7.0; (b) analytical curve of Ip vs. NO2– concentration
Analytical parameters of the calibration curve of nitrite on MWCNTs/Pt using the SWV method
Analytical parameters | 0.1 M PBS (pH = 7) |
---|---|
Peak potential, V | 0.67 |
Linearity range, µM | 1.0–1,000 |
Slope, µA/µM | 0.028 |
Intercept, µA | –0.219 |
Correlation coefficient | 0.995 |
SE of slope | 0.0006 |
SE of intercept | 0.001 |
LOD, µM | 0.10 |
LOQ, µM | 0.35 |
Within-day of peak current (RSD %)a | 0.32 |
Within-day of peak potential (RSD %)a | 0.02 |
Between-day of peak current (RSD %)a | 1.89 |
Between-day of peak potential (RSD %)a | 0.10 |
a Obtained from five measurements. PBS: phosphate-buffered solution; SE: standard error; LOD: limit of detection; RSD: relative standard deviation
The performance of the different modified electrodes for the detection of NO2–
Electrode materials | Medium | Potential (V) | LR (µM) | LOD (µM) | Ref. |
---|---|---|---|---|---|
Ag-HNT MoS2/GCE | 0.1 M PBS (pH = 4) | 0.8 V (Ag/AgCl) | 2–425 | 0.7 | [9] |
PEDOT-AuNPs/GCE | 0.1 M PBS (pH = 5) | 0.78 V (SCE) | 3–300 | 0.1 | [10] |
rGO-MoS2/GCE | 0.1 M PBS (pH = 7) | 0.8 V (Ag/AgCl) | 0.2–4,800 | 0.17 | [11] |
Cu/Ag/MWCNTs/GCE | 0.1 M PBS (pH = 7) | 0.85 V (SCE) | 1–1,000 | 0.2 | [12] |
nHAP-PEDOT/GCE | 0.2 M PBS (pH = 8) | 0.78 V (SCE) | 0.25–1.05 | 0.083 | [30] |
Fe2O3-MoS2/GCE | 0.1 M PBS (pH = 4) | 1.0 V (SCE) | 2–6,730 | 1.0 | [31] |
CG-Ppy-CS/GCE | 0.1 M AcHAC (pH = 4) | 0.87 V (Ag/AgCl) | 0.2–1,000 | 0.02 | [32] |
Poly (4-AB/OT)/CPE | 0.1 M PBS (pH = 5) | 0.95 V (Ag/AgCl) | 6–600 | 3.5 | [33] |
a-Fe2O3/CNTs/GC | 0.1 M PBS (pH = 7) | 0.95 V (Ag/AgCl) | 0.5–4,000 | 0.15 | [39] |
MWCNTs/Pt | 0.1 M PBS (pH = 7) | 0.70 V (SCE) | 1–1,000 | 0.1 | This work |
GCE: glassy carbon electrode; PBS: phosphate-buffered solution; CPE: carbon-paste electrode; AuNPs: gold nanoparticles; SCE: saturated calomel electrode; Poly (4-AB/OT): poly(4-aminobenzoic acid/o-toluidine); Ppy: polypyrrole; CG: carboxyl graphene; nHAP: nanosized hydroxyapatite; MWCNTs: multi-walled carbon nanotubes; LR: linear range; LOD: limit of detection; HNT: halloysite nanotube; GC: galssy carbon; PEDOT: poly(3,4-ethylenedioxythiophene); rGO: reduced graphene oxide; CNTs: carbon nanotubes; CS: chitosan; AcHAC: acetic acid-sodium acetate
Reproducibility, stability, and interference analyses of sensor
To test the stability of the MWCNTs/Pt electrode, the current responses of 50 μM NO2– were followed for 5 consecutive days. The oxidation current of NO2– remains practically unchanged with an RSD not exceeding 1.5% for five successive tests, showing clearly that the prepared electrode possesses good stability. Additionally, the reproducibility of the proposed sensor was tested with five different fabricated electrodes in 50 μM NO2–, and the RSD of the current signal was obtained as 2.04%, suggesting the good reproducibility of this electrochemical sensor.
In order to investigate the anti-interference property of the modified electrode, the effects of common interfering minerals and organic substances on the response sensor were examined. The electrode response to a 50 µM tested solution of NO2– in 0.1 M PBS (pH 7.0) is 1.26 µA. After adding 100-fold excess amounts of Na+, Cl–, K+, Zn2+, Ba2+, Mn2+, glucose, and ascorbic acid to the tested nitrite solution, no interference effect for the nitrite determination was observed (Table 4). The relative error values for the response were smaller than ± 5%, suggesting that the MWCNTs/Pt sensing electrode has good selectivity toward NO2– detection.
The effects of interfering substances on NO2– sensor
Interfering substances | Current (µA) | Relative error (%) |
---|---|---|
Na+ | 1.21 | –3.96 |
Cl– | 1.25 | –0.78 |
K+ | 1.24 | –1.58 |
Ba2+ | 1.19 | –3.45 |
Mn2+ | 1.31 | +3.96 |
Zn2+ | 1.32 | +4.76 |
Ascorbic acid | 1.27 | +0.79 |
Glucose | 1.21 | –3.90 |
Analysis of nitrite in real samples
To verify the practical applicability of the proposed sensor, NO2– was analyzed on MWCNTs/Pt. Known concentrations of NO2– were added to both mineral and source waters. The recovery study was realized thanks to the standard addition technique and the method can be applied for the NO2– detection in real effluents (Table 5).
Recovery study with the proposed method
Samples | Added (µM) | Found (µM) | Recovery (%) | RSD % (n = 5) | Bias % (n = 5) |
---|---|---|---|---|---|
Mineral water | 50 | 49.58 ± 1.33 | 99.16 | 2.68 | 2.00 |
Source water | 50 | 51.04 ± 0.89 | 102.08 | 1.74 | 5.30 |
RSD: relative standard deviation
Discussion
In summary, a novel sensor for the determination of nitrite was constructed using MWCNTs coated Pt electrode. The MWCNTs/Pt exhibited good electrocatalytic activity towards the oxidation of nitrite. Compared with previous reports for nitrite response, the method for nitrite determination displayed a good LOD of 0.1 μM. The main reason was ascribed to the large surface area and good conductivity of the modified electrode, which improves the electrode reaction kinetic towards NO2–. The results of this study indicate that the developed sensor has great potential for applications in the analysis of real samples including source water and mineral water.
Abbreviations
ChAm: | chronoamperometry |
CV: | cyclic voltammetry |
DMF: | N,N-dimethylformamide |
EIS: | electrochemical impedance spectroscopy |
LOD: | limit of detection |
MWCNTs: | multi-walled carbon nanotubes |
PBS: | phosphate-buffered solution |
RSD: | relative standard deviation |
SCE: | saturated calomel electrode |
SWV: | square wave voltammetry |
Declarations
Author contributions
MD: Conceptualization, Writing—original draft, Writing—review & editing, Investigation, Supervision. NKB: Investigation, Validation, Writing—review & editing. BS: Investigation. MT and SAO: Writing—review & editing. All authors read and approved the submitted version.
Conflicts of interest
The authors declare have no conflict of interest in financial, personal, or other relationships with other people, laboratories, or organizations.
Ethical approval
Not applicable.
Consent to participate
Not applicable.
Consent to publication
Not applicable.
Availability of data and materials
The raw data supporting the conclusions of this manuscript will be made available by the authors, without undue reservation, to any qualified researcher.
Funding
Not applicable.
Copyright
© The Author(s) 2024.