Abstract
Sterigmatocystin (STE) is a possible human carcinogenic compound (2B) according to the International Agency for Research on Cancer classification. Structurally, STE is a precursor to aflatoxins, sharing a similar polyketide-derived biosynthetic pathway, which underscores its toxicological relevance. It has been reported to occur in a variety of foodstuffs including cereals and cereal-based products, spices, cheese, and nuts, among others. STE poses a substantial challenge to food safety and addressing this issue requires a comprehensive strategy encompassing prevention, monitoring, and regulation to protect both human and animal health from its harmful effects. The present paper presents the analytical methodologies for the determination of STE in foodstuffs and the reported levels of STE in food, based on a review of scientific publications from 2021 to 2024. Significative progress has been made in the development of analytical methodologies for STE determination in food; however, further advancements in analytical techniques, standardized protocols, and monitoring are essential to improve risk assessment and guide effective mitigation strategies.
Keywords
Mycotoxins, sterigmatocystin, foods, analytical methods, concentration levelsIntroduction
Mycotoxins are toxic secondary metabolites produced by various fungal species that tend to infest crops, leading to contamination both during growth and after harvest. These naturally occurring toxins are primarily synthesized by molds such as Aspergillus, Fusarium, and Penicillium species, which can contaminate human foods and animal feeds under certain favorable conditions, such as optimal levels of moisture, water activity, and temperature [1, 2]. According to data from the Rapid Alert System for Food and Feed (RASFF), mycotoxins are the most frequently reported toxic substances and therefore represent a significant concern in food safety and public health due to their widespread occurrence and their carcinogenic, genotoxic, and hepatotoxic potential. However, the presence of mycotoxins in food and feed also has substantial economic implications due to crop losses and the costs associated with monitoring and decontamination processes [3].
Among mycotoxins, sterigmatocystin (STE) is a potent mycotoxin produced by certain fungi, particularly those belonging to the genera Aspergillus and Penicillium. The main producers A. versicolor and A. nidulans have garnered significant attention due to their widespread presence in cereals and animal feed [4]. Structurally, STE is a precursor to aflatoxins, the most potent carcinogenic mycotoxins known, sharing a similar polyketide-derived biosynthetic pathway, which underscores its toxicological relevance [2]. Specifically, STE is an intermediate in the biosynthetic pathway of aflatoxin B1 (AFB1) and AFG1 (Figure 1). In aflatogenic fungal species, STE is quickly converted into O-methylsterigmatocystin (OMST), the direct precursor of AFB1 and AFG1. Consequently, STE rarely accumulates, but certain species, such as A. nidulans and A. versicolor, appear unable to convert STE into OMST. As a result, substrates colonized by these fungi can contain high levels of STE [5].
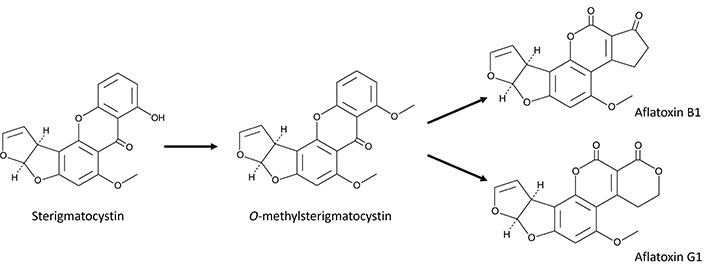
Scheme showing the conversion of sterigmatocystin and O-methylsterigmatocystin to aflatoxins B1 and G1
Understanding the mechanisms behind the conversion of STE into aflatoxins is essential for identifying the factors influencing aflatoxin production and contamination in agricultural commodities. Detailed knowledge of the enzymatic steps involved in this process can inform the development of strategies to mitigate aflatoxin contamination in food and feed. Additionally, uncovering the regulatory mechanisms of this conversion presents opportunities to create novel biocontrol agents or biotechnological approaches to inhibit aflatoxin biosynthesis in fungal pathogens, thereby improving food safety [5].
STE’s toxicity primarily stems from its ability to form DNA adducts, leading to mutations and carcinogenesis. It inhibits key cellular enzymes and disrupts protein synthesis, resulting in cell death and tissue damage. Studies have shown that STE induces oxidative stress and inflammation, contributing to its toxic effects [6, 7]. Exposure to STE is associated with various adverse health effects. Acute toxicity can result in liver and kidney damage, while chronic exposure is linked to an increased risk of liver cancer. Animal studies have demonstrated teratogenic effects, indicating potential risks to fetal development. According to the International Agency for Research on Cancer classification, STE is a possible human carcinogen (2B) [8].
STE is frequently detected in a variety of foodstuffs, including grains [9, 10], cereal products [11], nuts [12–14], coffee beans [15], cheese [16], and spices, among others, where its presence is often indicative of poor storage conditions and suboptimal agricultural practices [17]. Once contaminated, these products pose a significant risk to human and animal health if consumed.
The maximum levels of STE are not regulated within the European Union. Before their accession to the European Union, the Czech Republic and Slovakia had established STE limits of 5 μg/kg for certain cereals and milk [18]. Due to the lack of official STE control programs, there are no reliable assessments of human and animal dietary exposure [19]. More occurrence data on STE in food and feed across European countries need to be collected to allow assessment of dietary exposure.
Overall, STE poses a substantial challenge to food safety. Addressing this issue requires a comprehensive strategy encompassing prevention, monitoring, and regulation to protect both human and animal health from its harmful effects.
The present paper presents the analytical methodologies for the determination of STE in foodstuffs and the reported levels in food, based on a review of scientific publications from 2021 to 2024.
Regulations
On April 25, 2023, the European Commission introduced a new regulation focused on establishing maximum limits for certain contaminants in foodstuffs, such as mycotoxins, including aflatoxins, ochratoxin A, patulin, deoxynivalenol, zearalenone, fumonisins, citrinin, ergot sclerotia, and ergot alkaloids [20]. Before joining the European Union, the Czech Republic and Slovakia established STE limits of 5 μg/kg for certain cereals and milk [18, 21]. However, the maximum limits of STE are not yet regulated within the European Union. The Joint FAO/WHO Expert Committee on Food Additives (JECFA) has also evaluated STE, but there are no specific regulatory limits set by JECFA [22].
The European Food Safety Authority (EFSA) in Europe delivered a scientific opinion on the risk to public health related to the presence of STE in food and feed [19]. However, the EFSA Panel on contaminants in the food chain (CONTAM Panel) concluded that the available occurrence data were too limited to carry out a reliable human and animal dietary exposure assessment and reinforced the need for more occurrence data on STE in food and feed. Regarding the performance criteria of the analytical methods, a limit of quantification (LOQ) of less than 1.5 μg/kg should be applied [19].
Analytical methodologies for the determination of STE in food
Advanced detection methods are required to monitor and quantify STE levels in agricultural products, considering the LOQ set by EFSA. Current analytical methodologies for determining STE concentration levels in food have advanced significantly, enhancing selectivity, sensitivity, and accuracy. Table 1 summarizes the procedure and the analytical performance of the methodologies developed for the determination of STE in food from 2021 to 2024 (Scopus database, Elsevier).
Analytical performance of methodologies developed for the determination of sterigmatocystin (STE) in food from 2021 to 2024
Sample | Extraction | Determination | LOD/LOQ(μg/kg or µg/L) | Recovery (%) | Concentration levels (μg/kg) (number of samples, detection rate) | Ref | ||
---|---|---|---|---|---|---|---|---|
Brown rice, wheat | 25 g sample, 100 mL ACN:water (17:3, v/v)SPE (Horiba Aflaking IAC)Dried and reconstituted | LC-MS/MSInertSustain C18 (150 mm × 2.1 mm, 3 µm)2 mM NH4Ac in water/2 mM NH4Ac in ACN | 0.02–0.03/0.05–0.09 | 86–102 | Brown rice 0.35–5.70Polished rice 0.02–0.30Wheat 0.05–2.20Bread 0.02–0.20Baked sweets 0.01–0.20Noodles 0.01–0.80 | [23] | ||
Rice, maize, soybean | 5 g sample, 25 mL MeOH:water (7:3, v/v)30 min shakingSPE (HMON@MIP)TFA + hexane derivatizationDried and reconstituted | LC-FDSymmetry C18 (250 mm × 4.6 mm, 5 µm)ACN:water (32:68, v/v) | - | 81–95 | - | [24] | ||
Chilli, pepper | 50 g sample, 10% KCl in ACNLLE (2x hexane)LLE (hexane:CHCl3, 1:1 v/v)Dried and reconstituted | GO-FAM-FRET | 24/132 | 71–89 | - | [25] | ||
Soaked rice, steamed rice, fermented rice, fermented wine | QuEChERS | LC-MS/MSAcquity UPLC HSS T3 (100 mm × 2.1 mm, 1.8 μm)0.1% FA + 2 mM AF in water/0.1% FA + 2 mM AF in ACN | 0.01–0.07/0.03–0.25 | 73–119 | - | [26] | ||
Wheat | 10 g sample, 25 mL ACN:water (9:1, v/v), 1 g MgSO4, 1 g NaCl30 min shacking20 min centrifugationMSPE (Fe3O4-MIP) elution 5 mL MeOH:TEA (9:1, v/v)20 min shakingDried and reconstituted | LC-DAD | 0.63/- | 88–97 | 3.4–4.5 | [27] | ||
Cereals, nuts, vegetables, oil, noodle, paste, seasoned food, instant food | 5 g sample, 20 mL 0.1% FA in ACN:water (1:1, v/v)30 min shaking10 min centrifugationSPE (Isolute Myco) elution 2 mL 0.1% FA in ACN + 4 mL MeOHDried and reconstituted | LC-MS/MSImtakt Cadenza C18 (100 mm × 2 mm, 3 μm)0.1% FA + 5 mM AF in water/0.1% FA + 5 mM AF in MeOH | 0.4–0.9/1.2–2.8 | 69–112 | Processed foods 0.08–1.93 (n = 522, 4.2%)Agricultural products 0.08–10.07 (n = 613, 3.9%) | [28] | ||
Black, green, and Oolong teas | QuEChERS5.0 g sample, ACN:water (75:25, v/v)30 min UAE, 1 g NaCl, 1 g MgSO45 min centrifugationdSPE (C18)5 min centrifugation | LC-MS/MSShim-pack XR-ODS III (75 mm × 2.0 mm, 1.6 µm)0.1% FA + 5 mM AF in water/0.1% FA + 5 mM AF in ACN | 0.04–0.12/0.13–0.40 | 101–118 | 0.13–0.48 (n = 126, 13.5%) | [29] | ||
Wheat | 5 g sample, 20 mL ACN:water (8:2, v/v)10 min UAE5 min centrifugationMSPE (MHNTs@MIP) elution 5.3 mL EtOH:HAc (9:1, v/v)Dried and reconstituted | LC-DADHedera ODS-2 (250 mm × 4.6 mm)MeOH:water (60:40 v/v) | 1.1/3.5 | 89–103 | - | [30] | ||
Roasted coffee bean, black pepper | 10 g sample, 40 mL MeOH:water (8:2, v/v)5 min shaking2 min centrifugationSPE (Envi-carb SPE) elution 6 mL tolueneHexane cleaningSPE (IAC) elution 2 mL ACN | LC-MS/MSAcquity CSH C18 (150 mm × 2.1 mm, 1.7 μm)0.05 M FA and AF in MeOH:water (8:2, v/v)/water | 0.03/0.10 | 92–105 | 0.08–0.87 (n = 18, 22%) | [31] | ||
Rice, wheat | 5 g sample, 10 mL ACN:water (8:2, v/v)10 min UAE5 min centrifugationDried and redissolved in 10 mL water15 min dSPE [SiO2@mPMO-IL(im)2] elution 2 mL MeOH5 min centrifugationDried and reconstituted | LC-MS/MSODS (250 mm × 4.6 mm, 5 μm)ACN:MeOH:water (22:22:55, v/v/v)/ACN:MeOH:water (35:35:30, v/v/v) | 0.9–1.5/3.0–4.5 | 92–102 | - | [32] | ||
Coix seed | 5 g sample, 20 mL 0.1% FA in ACN:water (7:3, v/v)30 min mechanically shaking5 min centrifugation | SIDA-UHPLC-MS/MS | 0.03/0.10 | 83–88 | LOQ–23 (n = 60, 83%) | [33] | ||
Mango, litchi, longan, and their products | 2 g sample, 10 mL 1% HAc in ACN:water (8:2, v/v)10 min UAE5 min centrifugationdSPE (PSA, C18)10 min vortex shaking5 min centrifugationDried and reconstituted tube | LC-MS/MSAcquity BEH C18 (100 mm × 2.1 mm, 1.7 μm)Water/0.2% FA in ACN | 0.01/0.04 | 84–116 | - | [34] | ||
Honey | 1.5 g sample, 3 mL water, 2.5 mL ACN, 1 g MgSO4, 0.25 g NaCl, 0.25 g Na3Cit, 0.125 g Na2HCit1 min hand shaking10 min centrifugation2 min dSPE (MgSO4)10 min centrifugationDried and reconstituted | LC-MS/MSEclipse Plus C18 RRHT (100 mm × 2.1 mm, 1.8 µm)0.2 M NH4HCO3 in water/0.2 M NH4HCO3 in ACN | 0.3/1.0 | 101–103 | 0.4–18.7 (n = 57, 3.5%) | [35] | ||
Pale lager beer | 25 mL sample, pH adjustment to 7.4SPE (11+Myco MS-PREP IAC) elution 2 mL MeOHDried and reconstituted | LC-MS/MSAcquity UPLC BEH C18 (100 mm × 2.1 mm, 1.7 µm)1 mM NH4Ac + 0.5% HAc + 0.1% FA in water/0.5% HAc + 0.1% FA in MeOH | - | 27 | - | [36] | ||
Rice | 5 g sample, 10 mL ACN:water (8:2, v/v)10 min UAE5 min centrifugationDried and reconstituted in 10 mL water25 min MSPE (Fe3O4/ZIFs) elution 2 mL 10% FA in ACNDried and reconstituted | LC-DADODS column (250 mm × 4.6 mm, 5 μm)Water/0.05% H3PO4 in ACN | 0.4/1.2 | 79–95 | 1.2–2.2 (n = 56, 3.6%) | [37] | ||
Cocoa beans | 7.5 g sample, 18 mL 5% HAc in ACN:water (7:3, v/v), 3 g NaCl60 min shaking15 min freezing, –70°C10 min centrifugation | LC-MS/MSAcquity UPLC BEH C18 (100 mm × 2.1 mm, 1.7 μm)0.1% FA + 5 mM AF + 2% MeOH in water/0.1% FA in ACN | 3/10 | 97–109 | 10–11 (n = 135, 1.5%) | [38] | ||
Spice, herb | - | LC-MS/MS | - | - | 0.4–7.8 (n = 155, 4%) | [39] | ||
Rice, wheat | 5 g sample, 10 mL 10% FA in water, 10 mL ACN, 4 g MgSO4, 1 g NaCl, 1 g Na3Cit, 0.5 g Na2HCit5 min shaking5 min centrifugationSPE (Oasis Prime HLB) | LC-MS/MSAcquity HSS T3 C18 (100 mm × 2.1 mm, 1.8 μm)1% HAc + 5 mM NH4Ac in water/1% HAc + 5 mM NH4Ac in MeOH | 2/- | - | - | [40] | ||
Arecae semen | 2 g sample, 15 mL 0.2% FA in ACN:water (84:16, v/v)10 min UAE10 min centrifugationS-µSPE (MycoSpin 400)Dried and reconstituted | LC-MS/MSAcquity UPLC BEH C18 (100 mm × 2.1 mm, 1.7 µm)0.1% FA in MeOH/2 mM AF in water | 0.3/1.0 | 94–105 | LOQ–2.2 (n = 20, 15%) | [41] | ||
Plant-based milk alternatives | No sample treatment | ELISA | 2/- (ELISA) | - | Soy (n = 7, 14%)Almond (n = 7, 0%)Oat (n = 14, 14%)Others (n = 26, 8%) | [42] | ||
10 mL sample | LLE (EtAc)Dried and reconstituted in PBSSPE (VICAM AflaTest WB SR+ IAC) elution 3 mL MeOH | LC-MS/MS | Phenomenex C18 (100 mm × 3.0 mm, 5.0 µm)0.1% FA + 300 mg/L AF in water/0.1% FA + 300 mg/L AF in MeOH | |||||
Edible oil, soy sauce, bean sauce | 2 g sample, 20 mL ACN:water (8:2, v/v)10 min orbital shaking5 min centrifugationLLE (Hexane)3 min centrifugationSPE (Oasis PRiME HLB)Dried and reconstituted | LC-HRMSAccucore aQ C18 (150 mm × 2.1 mm, 2.6 μm)0.1% FA in water/0.1% FA in MeOH | 0.3/1.0 | 71–104 | Sesame oil LOQ–2.9 (n = 12, 8%) | [43] | ||
Corn, millet, rice, soybean, oats | 5 g sample, 25 mL ACN:water (8:2, v/v)30 min shakingCentrifugationSPE (COF@MIP) elution 5 mL ACNDried and reconstituted | LC-DADWaters Symmetry-C18 (250 mm × 4.6 mm, 5 µm)MeOH:water (80:20, v/v) | 2/8 | 79–98 | - | [44] | ||
Rice bran, maize | 1 g sample, 4 mL 1% FA in ACN:water (8:2, v/v)90 min vortex shaking15 min centrifugation | LC-MS/MSGemini C18 (100 mm × 4.6 mm, 5 μm)5 mM NH4Ac + 1% HAC in water/5 mM NH4Ac + 1% HAC in MeOH | 0.5/2.5 | 92–105 | Rice bran 2.8–272.3 (n = 125, 98%)Maize 0.3–17.9 (n = 125, 43%) | [45] | ||
Dry-cured meat products | QuEChERSSPE defatting (Captiva EMR-Lipid)SPE (Easi-extract sterigmatocystin IAC) | LC-MS/MSGemini (150 mm × 4.6 mm, 5 µm) | 0.02/0.06 | 114 | 0.10–3.93 (n = 250, 4%) | [46] | ||
Licorice | 2 g sample, 20 mL ACN:water (84:16, v/v)30 min UAEQuEChERS (4 g MgSO4, 1 g NaCl, 1 g Na3Cit, 0.5 g Na2HCit)30 min MSPE [Fe3O4@PDA/MIL-101(Cr)]Dried and reconstituted | LC-MS/MSAcquity UPLC BEH C18 (100 mm × 2.1 mm, 1.7 μm)5 mM NH4Ac + 0.1% NH3 in water/5 mM NH4Ac + 0.1% NH3 in MeOH | 0.09/0.30 | 107–116 | - | [47] | ||
Long-ripened Grana cheese | 10 g sample, 50 mL ACN:water (8:2, v/v)60 min rotary shakingFiltration, 2 mL PBSSPE (R-Biopharm-Rhône IAC) elution 6 mL ACNDried and reconstituted | LC-MS/MSBetasil RP-18 (150 mm × 2.1 mm, 5 µm)0.2% FA in water/0.2% FA in ACN | 0.05/0.15 | 87–92 | LOQ–6.9 (n = 107, 94%) | [48] | ||
Pseudostellariae Radix | ACN:water (8:2, v/v)1 h shakingdSPE (PSA + C18 + MgSO4) | LC-MS/MSAcquity HSS T3 (100 mm × 2.1 mm, 1.8 μm)0.1% FA in water/0.1% FA in ACN | - | - | 1.5–69.6 (n = 26, 38%) | [49] | ||
Peanut butter, hazelnut butter, chocolate | 5 g sample, 20 mL JSM FO 970415 min shaking5 min centrifugation | LC-MS/MS | 0.01–0.02/0.05–0.15 | 94–100 | 0.2–2.2 | [50] | ||
Cocoa | 7.5 g sample, 18 mL 0.5% HAc in ACN:water (7:3, v/v), 3 g NaCl60 min shaking15 min frozen at –70°C10 min centrifugation | LC-MS/MSTitan C18 (100 mm × 2.1 mm, 1.9 μm) | - | - | 2.4–3.3 (n = 18, 11%) | [51] | ||
Carob | 1 g sample, 10 mL water, 10 mL 1% HAc in ACN10 min UAE10 min shaking, 1 g NaCl, 4 g MgSO4Dried and reconstituted | LC-MS/MSTitan C18 (100 mm × 2.1 mm, 1.9 μm) | - | - | 0.15–0.18 (n = 22, 14%) | [51] | ||
Cheese | 2.5 g sample, 5 mL 0.1% FA in ACN, 5 mL saturated MgSO430 min shaking7 min centrifugationDefatting, 4 mL heptane5 min shakingDried and reconstituted | LC-MS/MSGemini C18 (100 mm × 3.0 mm, 5.0 μm)0.1% FA + 300 mg/L AF in water/0.1% FA + 300 mg/L AF in MeOH | 0.01/0.04 | 100–106 | 0.08–4.99 (n = 11, 82%) | [52] | ||
Goat, camel, and cow milk | 1 mL sample, 1 mL 1% FA in ACN, 0.4 g MgSO4, 0.1 g NaCl10 min centrifugation | LC-MS/MSAcquity HSS T3 (100 mm × 2.1 mm, 1.8 μm)0.1% HAc + 5 mM NH4Ac in water/0.1% HAc + 5 mM NH4Ac in MeOH | - | - | LOQ–7.7 (n = 135, 14%) | [53] | ||
Cereal-based baby food | 2 g sample, 10 mL JSM FO 970415 min shaking5 min centrifugation | LC-MS/MS | 0.02/0.07 | 100 | 0.02–0.50 (n = 85, 34%) | [54] | ||
Rice, peanut, maize, sorghum | 5 g sample, 20 mL 1% HAc in ACN:water (8:2, v/v) | LC-MS/MS | - | - | Peanut 0.1–30 (n = 53, 40%)Maize 0.1–12 (n = 142, 26%)Rice 0.1–2.2 (n = 23, 48%)Sorghum 0.1–2.5 (n = 24, 12%) | [55] | ||
Hazelnut kernels | 25 g sample, 100 mL MeOH:water (8:2, v/v), 5 g NaCl3 min extractionPBS dilutionSPE (Easi-Extract Sterigmatocystin IAC) elution 1.5 mL ACNDried and reconstituted | LC-FLDODS2 C18-300 (150 mm × 4.6 mm, 3 μm)120 mg/L KBr + 350 µL/L HNO3 in ACN:MeOH:water (10:15:75, v/v) | 1.3/4.2 | 81–87 | 9–101 (n = 30, 5%) | [56] | ||
Herbs, herbal infusions | 1 g herb sample, 5 mL water, 30 min shaking | 5 mL 1% FA in ACN, 2 g MgSO4, 1 g NaCl1 h orbital shaking15 min centrifugationdSPE (C18, Z-sep+)Dried and reconstituted | LC-MS/MSKinetex C18 (150 mm × 4.6 mm, 2.6 μm)5 mM NH4Ac in water:MeOH:HAc (94:5:1, v/v)/water:MeOH:HAc (2:97:1, v/v) | 0.5–20/2.5–40 | 73–101 | 34–147 (n = 58, 19%) | [57] | |
1 g infusion sample, 50 mL hot water, 15 min shacking | 5 min centrifugation5 mL ACN, 2 g MgSO4, 1 g NaCl5 min centrifugationDried and reconstituted | |||||||
Malt, beer | 5 g malt sample, 2 g MgSO4, 1 g NaCl, 15 mL ACN:water (75:25, v/v) | 6 min centrifugationd-SPE (MgSO4, C18)Dried and reconstituted | LC-HRMS/MSKinetex Core-Shell F5 100 A (2.6 µm)0.1% HAc + 4 mM NH4Ac in water/0.1% HAc + 4 mM NH4Ac in ACN | 5/12 | 90–97 | LOQ (n = 47, 0%) | [58] | |
5 mL beer sample, 5 mL ACN, 2 g MgSO4, 1 g NaCl | 3 min UAE5 min centrifugationdSPE (MgSO4, C18)Dried and reconstituted | |||||||
Rice bran | 20 g sample, 80 mL MeOH:water (8:2, v/v)15 min UAE5 min centrifugationDLLME (CHCl3/water)5 min centrifugationDried and reconstituted | LC-MS/MSAccucore C18 (100 mm × 2.1 mm, 2.6 μm) | 2.5/5.0 | 99 | LOQ (n = 24, 0%) | [59] | ||
Milled rice | 5 g sample, 20 mL 1% FA in ACN:water (8:2, v/v)90 min shaking | LC-MS/MSSynergi Hydro-RP (100 mm × 3 mm, 2.5 µm)1% FA and 10 mM NH4Ac in water/MeOH | 0.03/0.09 | 80 | LOQ–7 (n = 200, 74%) | [60] | ||
Dairy products | 2 g sample, 8 mL 2% FA in ACN30 min UAE5 min centrifugationSPE (Captiva EMR-lipid)Dried and reconstituted | LC-MS/MSShiseido C18 (100 mm × 2.1 mm, 3 μm)0.1% FA in water/0.1% FA in MeOH | 0.005/0.020 | 73 | LOQ (n = 76, 0%) | [61] | ||
Milling oats | 5 g sample, 20 mL 1% HAc in ACN:water (8:2, v/v)90 min shakingS-µSPE (MycoSpin 400)Dried and reconstituted | LC-MS/MSEclipse Plus C18 (100 mm × 2.1 mm, 1.8 µm)0.1% HAc + 5 mM NH4Ac in water/0.1% HAc + 5 mM NH4Ac in MeOH | -/1 | 95 | 1–7 (n = 281, 2.3%) | [62] | ||
Garlic | 5 g sample, 20 mL 1% HAc in ACN:water (8:2, v/v)Vortex shaking | LC-MS/MSGemini C18 (150 mm × 4.6 mm, 5 μm) | 0.05/0.14 | 90 | 3–32 (n = 36, 100%) | [63] | ||
Coix seed | 5 g sample, 20 mL 1% FA in ACN:water (7:3, v/v)20 min vortex shaking5 min centrifugation | LC-HRMSCORTECS C18 (100 mm × 2.1 mm, 1.6 μm)0.1% FA + 1 mM NH4Ac in water/0.1% FA + 1 mM NH4Ac in MeOH | -/1 | 76–89 | 1–51 (n = 77, 30%) | [64] |
-: not indicated. SPE: solid-phase extraction; LOD: limit of detection; LOQ: limit of quantification; LC: liquid chromatography; MS: mass spectrometry; HMON@MIP: hollow-structured microporous organic networks coated with molecularly imprinted polymers; FD: fluorescence; GO-FAM-FRET: graphene oxide-aptamer-FD resonance energy transfer; QuEChERS: quick, easy, cheap, effective, rugged, and safe; MSPE: magnetic SPE; DAD: diode array; UAE: ultrasound-assisted extraction; dSPE: dispersive SPE; SiO2@mPMO-IL(im)2: ionic liquid-functionalized mesoporous multipod silica; UHPLC: ultra-high-performance LC; PSA: primary secondary amine; ZIFs: zeolitic imidazolate frameworks; COF@MIP: MIPs-coated covalent organic framework nanoflowers; DLLME: dispersive liquid-liquid microextraction; HRMS: high-resolution MS; ELISA: enzyme-linked immunosorbent assay; ACN: acetonitrile; IAC: immunoaffinity column; TFA: trifluoroacetic acid; LLE: liquid-liquid extraction; FA: formic acid; AF: ammonium formiate; TEA: triethylamine; SIDA: stable isotope dilution assay; S-µSPE: spin micro SPE; PBS: phosphate buffer saline; FLD: fluorescence detector; MHNTs@MIP: magnetic halloysite nanotubes coated molecular imprinted polymer
Liquid chromatography (LC) was the most widely employed technique due to its high-resolution power, being coupled with diode array (DAD) [30, 37, 44], fluorescence (FD) [56], or mass spectrometry (MS) [26, 35, 57] detectors. Reverse-phase chromatography with octadecyl silica (C18) stationary phase was the primary type of chromatographic column used for the separation of STE from other mycotoxins and food constituents. Columns with an average particle diameter of 5 μm were typically used in conventional LC [24, 32, 38], while 1.8 μm diameter columns were employed in ultra-high-performance LC (UHPLC) applications [26, 36]. Chromatographic separation was mainly based on reverse phase mechanisms, using columns, such as Acquity UPLC BEH C18 [35], Acquity UPLC HSS T3 [26], and Symmetry-C18 [24] from Waters Corporation (Milford, MA, USA), Gemini C18 [48] from Phenomenex (Torrance, CA, USA), and Zorbax Eclipse Plus C18 [36, 43] from Agilent Technologies (Santa Clara, CA, USA). Regarding the mobile phase, methanol/water and acetonitrile (ACN)/water gradients were employed to achieve the right separation of STE from other sample constituents, with small amounts of formic acid (FA), acetic acid, and/or ammonium buffers added as modifiers.
LC coupled to tandem MS (LC-MS/MS) provides the highest selectivity and sensitivity, making it the preferred technique in the currently developed methodologies developed for the identification and quantification of STE at trace levels in complex food matrices. Moreover, LC-MS/MS technique allows the development of multiresidue analysis for the determination of STE and other mycotoxins in a wide variety of food matrices, using electrospray ionization in both positive and negative modes. For example, aflatoxins (B1, B2, M1, M2, P1), ochratoxins (A, B), enniatins (A, A1, B, B1), beauvericin, citrinin, dihydrocitrinone, zearalanol, and alternariol monomethyl ether were detected in camel, cow, and goat milk (36 analyzed mycotoxins) [53]; and 3-acetyl-deoxynivalenol, 15-acetyl-deoxynivalenol, fusarenone-X, patulin, deepoxy-deoxynivalenol, tenuazonic were also detected in fresh and dried mango, litchi and longan fruits, and processed products (44 analyzed mycotoxins) [34].
One of the most relevant and recent innovations in the development of methodologies for the multiresidue analysis of mycotoxins focuses on the use of high-resolution MS (HRMS). This technique allows not only the unambiguous identification of the mycotoxins present in food samples but also allows the non-targeted analysis of the obtained data, enabling the identification of additional compounds. In this sense, LC-HRMS/MS has been applied in the determination of STE in food samples, providing extreme selectivity and high sensitivity. Some recent examples include the simultaneous determination of legislated and emerging mycotoxins in rice and wheat grains [37], malted barley and beer [58], and coix seeds [33], using quadrupole-time of flight MS detectors. Moreover, LC-HRMS/MS approaches enable the identification of emerging mycotoxins and unknown compounds without analytical standards in current and retrospective analyses, especially using Orbitrap mass spectrometers. This includes the determination of STE and other mycotoxins in coix seeds [64], as well as in edible oil, soy sauce, and bean sauce [43].
The use of immunoassays, such as enzyme-linked immunosorbent assays (ELISAs), is a valuable alternative or complementary approach to LC-MS/MS, offering a rapid and cost-effective screening tool suitable for high-throughput analysis. However, it may be less specific and sensitive compared to chromatographic techniques. In the last decades, ELISA has been widely employed for determining mycotoxins in food [65]. Recently, ELISA has been widely employed for the determination of STE, AFB1, ochratoxin A, deoxynivalenol, and T-2/HT-2-toxin in soy, oat, almond, and coconut-based milk alternatives. Significant sample matrix interferences were observed even with a 1:8 dilution, compromising both result accuracy and detection limits [42]. With significant technological advancements in LC-MS instrumentation enabling highly sensitive multitoxin analysis, ELISA is now losing its prominent position. Nevertheless, rapid and cost-effective ELISA tests still hold great potential as a screening tool to reduce the number of samples that need to be analyzed by reference official methodologies.
Moreover, cutting-edge methodologies have been developed, such as the graphene oxide-aptamer-FD resonance energy transfer (GO-FAM-FRET) one-step FD turn-on aptasensor for the one-step detection of STE in chili and pepper, with insignificant interferences from salts and detergents and negligible cross-reactivity with other mycotoxins [25].
Despite the great advancements in analytical methodologies, challenges remain in ensuring consistent and reliable STE detection across various food products. Matrix effect may complicate the accuracy and precision of LC-MS/MS measurements. Thus, efforts to standardize sample preparation protocols and improve extraction efficiencies are ongoing to address these issues. Quick, easy, cheap, effective, rugged, and safe (QuEChERS) based methodologies have been validated for the multianalyte determination of mycotoxins, including STE, in a wide variety of food samples, such as mango, litchi, longan, and their products [34], black, green, and Oolong teas [29], Pseudostellariae Radix [49], and dry-cured meat products [46]. STE extraction is carried out using ACN:water or methanol:water buffers, usually accelerated by using ultrasound-assisted extraction (UAE) [27], followed by a dispersive solid-phase extraction (dSPE) to clean up the extracts, using MgSO4 [35], C18 [29], MgSO4 and C18 [58], MgSO4, C18, and primary secondary amine (PSA) [49], or even specifically dedicated sorbents like an ionic liquid-functionalized mesoporous multipod silica [SiO2@mPMO-IL(im)2] [32].
The use of SPE was frequently employed for a more selective extraction of STE from extracts using immunoaffinity columns (IACs), such as Easi-extract sterigmatocystin (R-Biopharm AG, Pfungstadt, Germany) specific for STE extraction [46, 48, 56], Aflaking (Horiba, Kyoto, Japan) [23] and AflaTest WB SR+ (VICAM, Watertown, MA, USA) [42] specific for aflatoxin related mycotoxins, and Isolute Myco (Biotage, Uppsala, Sweden) [28] and 11+Myco MS-PREP (R-Biopharm AG, Pfungstadt, Germany) [36] for a generic extraction of mycotoxins. Conventional SPE cartridges have also been proposed for clean-up purposes, such as Oasis PRiME HLB (Waters Corporation) [40, 43] and Supelclean Envi-carb (Merck KGaA, Darmstadt, Germany) [31]. Captiva EMR-lipid (Agilent Technologies) SPE columns were employed for lipid removal of fatty samples, such as dry-cured meat [46] and dairy products [61]. Additionally, specifically synthesized solid sorbents were also employed for mycotoxin extraction, such as hollow-structured microporous organic networks coated with molecularly imprinted polymers (HMON@MIP) [24], and MIPs-coated covalent organic framework nanoflowers (COF@MIP) [44] for the specific enrichment of STE and aflatoxins from cereal extracts.
Magnetic SPE (MSPE) has been proposed by many authors to improve the cleaning-up of sample extract in QuEChERS-based methodologies, using Fe3O4-based magnetic sorbents coated with MIPs [27], zeolitic imidazolate frameworks (ZIFs) [37], and polydopamine/metal-organic framework [PDA/MIL-101(Cr)] [47] for the determination of STE in wheat, rice, and licorice samples, respectively. Moreover, magnetic halloysite nanotubes were also proposed as magnetic sorbents coated with a specific MIP for the selective enrichment of STE in wheat samples [30].
Finally, other minority approaches have been employed for the clean-up of samples extracts, such as centrifugation-assisted SPE using selective MycoSpin 400 (Romer Labs, Tulln, Austria) cartridges in survey of mycotoxins made in Arecae semen [41] and milling oats [62], and dispersive liquid-liquid microextraction (DLLME) with chloroform for multi-mycotoxin determination in rice bran [59].
Concentration levels and detection rate of STE in food
Occurrence data of STE in food raises an important issue in food safety due to its carcinogenic potential. Data from scientific publications from the year 2021 to 2024 are shown in Table 1. As can be seen, the presence of STE in foodstuffs has been reported in a limited number of publications. Figure 2 shows the concentration levels (on a logarithmic scale), classified in the food categories cereals and cereal-based products (in green); herbs, seeds, and spices (in orange); and miscellanea (in blue; including cocoa, coffee, cheese, honey, meat products, nuts, garlic, and others). Results show that concentrations of STE in cereal and cereal-based products range from a few μg/kg up to more than 250 μg/kg. The highest level of STE (272.3 μg/kg) was detected in rice bran from Southeast Asia [45], while the lowest levels were detected in cereal products, such as noodles (0.01–0.8 μg/kg) [23], cereal-based baby food (0.02–0.5 μg/kg) [54], and bread (0.02–0.2 μg/kg) [23]. STE was also detected in maize (0.1–17.9 μg/kg) [24, 45, 55], oats (1–7 μg/kg) [62], brown rice (0.35–5.7 μg/kg) [23], white rice (0.02–2.2 μg/kg) [23, 37, 55], and wheat (0.05–2.2 μg/kg) [23]. Overall, the STE concentration levels in cereals and cereal-based products show that the highest levels were found in bran or non-treated cereals, whereas products like polished rice or cereal-based products presented the lowest levels. The comparative analysis of mycotoxin levels in whole cereals versus cereal-based products underscores the importance of food processing and quality control in reducing mycotoxin contamination. While whole cereals are more prone to higher mycotoxin contamination due to direct exposure and favorable conditions for fungal growth, cereal-based products benefit from processes that reduce mycotoxin levels, making them generally safer for consumption.
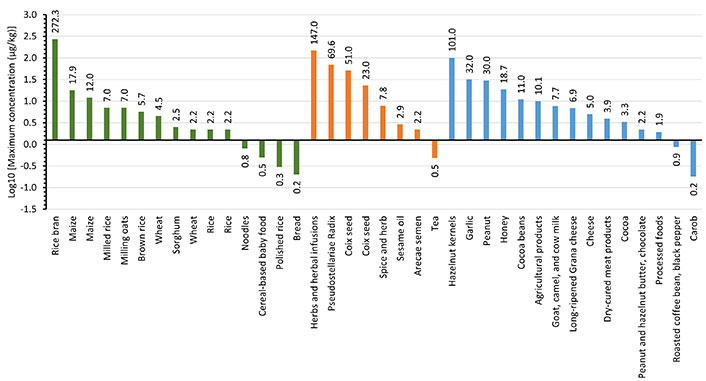
Decimal logarithm of maximum concentration levels of sterigmatocystin in cereals and cereal-based products (green bar), herbs, seeds, and spices (orange bar), and miscellanea (blue bar). The numbers on the bars indicate the concentration (μg/kg)
Regarding the category herbs, seeds, and spices, the highest level of STE (34–147 μg/kg) was found in herbs and herbal infusions [57] and the lowest levels were detected in tea (0.13–0.48 μg/kg) [29]. STE levels were found also in Pseudostellariae Radix (1.5–69.6 μg/kg) [49], coix seed (1–51 μg/kg) [33, 64], sesame oil (LOQ–2.9 μg/kg) [43], and Arecae semen (LOQ–2.2 μg/kg) [41].
In the miscellanea category, data showed that high concentrations of STE were found in nuts such as hazelnut (0.02–101 μg/kg) [50, 56], peanuts (0.1–30 μg/kg) [55], and garlic (3–32 μg/kg) [63]. STE was also found in honey (0.4–18.7 μg/kg) [35], cocoa (2.4–11 μg/kg) [38, 51], milk (LOQ–7.7 μg/kg) [53], cheese (0.08–4.99 μg/kg) [52, 61], meat products (0.1–3.93 μg/kg) [46], coffee (0.08–0.87 μg/kg) [31], and carob (0.15–0.18 μg/kg) [51]. In cheese, contamination occurs particularly on the surface after fungal deterioration during ripening and storage.
On the other hand, the frequency of detection of STE is influenced by food type, geographical region, and the testing methods used. Cereals and grains show the highest prevalence, particularly in regions with conducive climates for fungal growth. As can be observed in Figure 3, no differences were observed in the frequency of detection between food categories (cereal and cereal-based products 2.3–98%, herbs, seeds, and spices 4–83%, and miscellanea 1.5–100%). The highest detection frequency of STE (100%) was found in garlic [63], followed by rice bran (98%) [45], and cheese (82–94%) [48, 52].
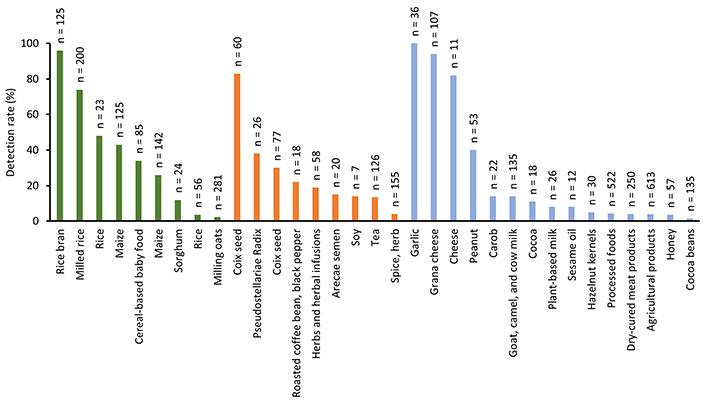
Detection rate of sterigmatocystin in cereals and cereal-based products (green bar), herbs, seeds, and spices (orange bar), and miscellanea (blue bar). Legend shows the number of analyzed samples
Conclusions
The ongoing development and refinement of analytical methodologies are crucial for maintaining the safety of the food supply. Collaborative efforts between researchers, regulatory bodies, and industry stakeholders are essential to enhance detection capabilities, standardize testing protocols, and ensure effective regulatory enforcement. Additionally, continued research into the occurrence, distribution, and toxicity of STE will inform risk assessments and guide the development of more targeted and effective mitigation strategies.
In conclusion, while significant progress has been made in the analytical determination of STE and other mycotoxins in food, continued advancements are necessary to address existing challenges and ensure the safety of the food supply. Enhanced analytical techniques, standardized protocols, and rigorous monitoring are critical components of an integrated approach to managing STE contamination and assessing the risk assessment of dietary exposure in populations. By prioritizing these efforts, we can protect public health and maintain consumer confidence in the safety of our food systems.
Abbreviations
AFB1: | aflatoxin B1 |
EFSA: | European Food Safety Authority |
ELISA: | enzyme-linked immunosorbent assay |
FD: | fluorescence |
HRMS: | high-resolution mass spectrometry |
LC: | liquid chromatography |
LOQ: | limit of quantification |
MS: | mass spectrometry |
SPE: | solid-phase extraction |
STE: | sterigmatocystin |
Declarations
Author contributions
OP: Conceptualization, Data curation, Formal analysis, Funding acquisition, Investigation, Methodology, Supervision, Validation, Visualization, Writing—original draft, Writing—review & editing. FAET: Conceptualization, Data curation, Formal analysis, Funding acquisition, Investigation, Methodology, Supervision, Validation, Visualization, Writing—original draft, Writing—review & editing.
Conflicts of interest
Olga Pardo and Francesc A. Esteve-Turrillas, who are the Guest Editors of Exploration of Foods and Foodomics had no involvement in the decision-making or the review process of this manuscript.
Ethical approval
Not applicable.
Consent to participate
Not applicable.
Consent to publication
Not applicable.
Availability of data and materials
Not applicable.
Funding
This study was funded by the Conselleria d’Educació, Universitats i Ocupació from Generalitat Valenciana project [CIAICO2022/217]. The funders had no role in study design, data collection and analysis, decision to publish, or preparation of the manuscript.
Copyright
© The Author(s) 2024.