Abstract
Seafood is both nutritionally and economically significant, with bivalve molluscs being particularly valuable for monitoring environmental pollutants due to their filter-feeding nature and ability to bioaccumulate pollutants. While not often linked to food poisoning, these molluscs can occasionally introduce health risks, highlighting the need for vigilant monitoring. This review provides a thorough analysis of pollutants—including persistent and emerging pollutants, as well as marine toxins—found in bivalve molluscs between 2019 and 2024. Among the studied pollutants, plasticizers and alkaloids are the most frequently analyzed, with liquid and gas chromatography (GC) tandem mass spectrometry (MS) the predominant methods, although novel approaches to determine these compounds, such as sensors, have also emerged in recent years. However, many studies are focused on establishing pollutant content without addressing bioaccumulation (BA) factors, and a lack of standardization in species and sampling locations complicates comparisons between the different published works. Despite some studies linking human activity and algal blooms to BA dynamics, more comprehensive research is needed. Additionally, limited data on the depuration capacity of molluscs underscores the need for further investigation. Although pollutant levels generally remain within legal limits, many substances remain unregulated. Environmental factors also play a critical role in influencing BA, emphasizing the need for future studies to focus on BA factors to better understand these complex dynamics.
Keywords
Bioaccumulation, bivalve molluscs, environmental pollutants, marine toxins, filter-feeding organismsIntroduction
Seafood, which predominantly includes fish, shellfish (such as crustaceans, molluscs, or echinoderms), and seaweeds, plays a crucial role in the food industry due to its high nutritional value, its wide use from a culinary point of view, and its potential role in the functional food and nutraceuticals industry. Focusing on shellfish, they are usually rich in digestible proteins, essential amino acids, bioactive peptides, long-chain polyunsaturated fatty acids, carotenoids, vitamins (mainly B12), and minerals [1]. As a consequence, its consumption offers numerous health benefits to consumers. However, their occurrence in diverse habitats, their filter-feeding nature, and poor farming and handling practices can occasionally introduce health risks to consumers [2]. These risks include the presence of pathogenic organisms, parasites, biotoxins, industrial and environmental pollutants, heavy metals, process-related additives like antibiotics and bisulfite, and allergy-causing compounds [1]. Therefore, it is necessary that health organizations strictly monitor the safety of shellfish and other seafood. In this regard, bivalve molluscs (such as oysters, clams, and mussels, among others) have been most widely used as model organisms and/or bioindicators to monitor the presence, toxicity, and also the bioaccumulation (BA) of pollutants due to several unique attributes. Their sedentary and filter-feeding nature, their easy sampling, their wide distribution in natural habitats, and their resistance to different environmental conditions can cause the uptake and BA of diverse substances from their ecosystem over a relatively long life span [3, 4].
Pollutants are likely to end up in aquatic environments (and subsequently in seafood), making their continuous release a great problem since this release gradually increases their concentration in the environment [5]. Due to their efficient diffusion in water and oceanic hydrodynamic movement, these pollutants can be found far away from their source and accumulate in diverse marine habitats [6]. These pollutants mainly include compounds that come from human activities and from biological sources, such as marine toxins [7].
Pollutants coming from human activities include persistent organic pollutants (POPs) [8–12], endocrine-disrupting chemicals (EDCs) [13–18], pharmaceuticals and personal care products (PPCPs) [18–21], polycyclic aromatic hydrocarbons (PAHs) [8, 22], heavy metals [23], pesticides (such as organochlorines, organophosphates, and carbamates, and more recently pyrethroids and neonicotinoids) [24, 25], surfactants [26] and microplastics (MPs) [27], among others.
POPs are compounds that resist environmental degradation through chemical, biological, and photolytic processes [8, 12]. EDCs can be harmful to exposed organisms, potentially causing adverse effects on fertility and fecundity in wildlife, leading to the masculinization of females, feminization of males, and disruptions in fish spawning timing [13–18]. PPCPs include different types of drugs found in both veterinary and human health products, fragrances, disinfectants, insect repellants, sunscreen UV filters, soaps and detergents, etc. [18–21]. More info about these three types of pollutants is included in Table 1.
Summary of pollutants coming from human activities
Family | Examples | Other information |
---|---|---|
Persistent organic pollutants (POPs) | Polychlorinated biphenyls (PCBs) | Widely used due to their insulating properties as dielectrics in transformers and capacitors and lubricants in turbines and pumps |
Polybromodiphenyl ethers (PBDEs) | Mainly used as flame retardants in plastics and textiles | |
Polychlorinated dibenzo-p-dioxins (PCDDs) | Carcinogenic dioxins | |
Dioxin-like PCBs (DL-PCBs) | ||
Organochlorine pesticides (OCPs) | Toxicity, bioaccumulation (BA), mutagenicity, and teratogenicity | |
Endocrine-disrupting chemicals (EDCs) | Hormones | Naturally produced hormones such as testosterone, estrone, and 17b-estradiol |
Bisphenols | Bisphenol A (BPA) and its analogues, are mainly used as precursors of epoxy resins and polycarbonate plastics for food packaging coating, thermal papers, household appliances, and adhesives | |
Phthalates | Phthalate esters (PAEs), are widely used as plasticizers in different types of products such as food packaging, personal care products, polyvinylchloride resins, construction materials, pesticide carriers and cosmetics, electronic equipment, and insect repellents, etc. | |
Alkylphenols | Obtained after the degradation of alkylphenol ethoxylates, which are used in the production of plasticizers and emulsifiers, or as nonionic surfactants | |
Per- and polyfluoroalkyl substances (PFASs) | Utilized in a variety of applications including water-repellent coatings, stain-resistant fabrics, and firefighting foams | |
Pharmaceuticals and personal care products (PPCPs) | Flame retardant compounds | Tris(2-butoxyethyl) phosphate (TBEP) |
Anti-inflammatory drugs | Diclofenac or ibuprofen | |
Antibiotics | Macrolides, sulfonamides and quinolones |
Pollutants like those previously mentioned are not only problematic in the environment, but they also pose severe health risks. PAHs, for instance, are known carcinogens, while per- and polyfluoroalkyl substances (PFASs) are associated with a range of health issues, including endocrine disruption and immune system suppression [28–30]. Understanding the levels of these pollutants in bivalves helps in assessing potential risks to human health, while understanding their BA patterns (including factors influencing their uptake and elimination), allows for a more comprehensive environmental risk assessment. This knowledge is crucial for creating effective environmental policies and safeguarding aquatic ecosystems.
Other important pollutants are MPs, which are ubiquitous in marine environments and can serve as significant vectors for the accumulation and transport of environmental pollutants. This phenomenon enhances the complexity of assessing pollution impacts in marine ecosystems. Due to their small size, MPs have a high surface area relative to their volume. This characteristic facilitates the adsorption of pollutants from the surrounding environment, apart from those that can be already in their structure, such as bisphenols and phthalates. The surrounding pollutants, which are often present in trace amounts in seawater, can become concentrated on the surfaces of MPs [31]. Therefore, marine organisms, particularly filter-feeders and sediment feeders, can ingest MPs along with their natural food sources [32]. Once ingested, MPs and their associated pollutants can accumulate in the tissues of marine organisms. Hence, effective environmental monitoring and management strategies must address both MPs and the pollutants they carry to mitigate their effects on marine biodiversity and human health [33, 34].
In addition to these pollutants coming from human sources, marine organisms are also affected by specific climatic and hydrographic conditions, including the continuous rise of seawater temperatures, changes in water salinity, shifts in nutrient-rich currents, and acidification due to the increase of CO2 levels [5]. These factors could increase the frequency of high-density algae clouds, also called algal blooms, leading to nutrient depletion and the production of phycotoxins (natural/marine toxins) [7]. Furthermore, these factors can also contribute to a rise in pathogens such as Vibrio spp., as well as the physiological state of the bivalve and its predisposition to bacterial infections [5, 35], affect the transfer of pollutants through trophic webs, and alter the toxicity of pollutants within marine organisms [36].
Marine toxins have attracted researchers’ attention due to their role in human intoxication and the significant socioeconomic impacts resulting from such incidents. Its ingestion can induce different types of symptoms, starting from gastrointestinal disorders, although they can also cause neurological problems and even death [23]. Toxins produced by algae are estimated to cause around 60,000 human intoxications worldwide each year [37]. The most common intoxications linked to toxin-contaminated bivalves are amnesic shellfish poisoning (ASP), diarrheic shellfish poisoning (DSP), and paralytic shellfish poisoning (PSP), while azaspiracid poisoning (AZP) and neurotoxic shellfish poisoning (NSP) are less frequent and appear to be more endemic [38]. According to the chemical properties, marine toxins can be divided into two groups: hydrophilic and lipophilic toxins. The hydrophilic ones, characterized by a molecular weight (MW) below 500 Da, are mainly associated with ASP and PSP syndromes, while lipophilic toxins, with a MW, comprised between 600 Da and 2,000 Da, are responsible for NSP, DSP, and AZP [7].
The main hydrophilic toxins are domoic acid (DA) and saxitoxin (STX) and its analogues. DA is the main toxin responsible for ASP; the diatom Pseudo-nitzschia pungens is one of the important species responsible for DA production. Instead, STXs are responsible for PSP, mainly produced from dinoflagellates of the Alexandrium genus. The main lipophilic toxins are: (i) brevetoxins, produced by the algae, Karenia ssp., and responsible for NSP; (ii) okadaic acid (OA) and dinophysistoxins (DTXs), produced by the Dinophysis genus, that causes DSP; (iii) azaspiracids (AZAs), that cause of AZP, are produced from the dinoflagellate Azadinium spinosum; and (iv) other potentially toxic microalgal metabolites such as pectenotoxins (PTXs) and yessotoxins (YTXs) [7, 38]. Therefore, the analysis of marine toxins in bivalves is a crucial aspect to ensure seafood safety and public health, given the potential risks posed by harmful algal blooms and other marine pollutants. It is important to evaluate the presence of these pollutants in bivalves to achieve a deep understanding of their BA.
In this regard and given the wide variability of organic pollutants (from human and biological sources) found in both bivalves and in oceanic waters and sediments, it is necessary to define different biomarkers that describe the effects of pollution in aquatic organisms, including the BA process, for both, public health and for better management of ocean and coastal resources. Hence, a comprehensive evaluation of recent findings from the last five years (2019–2024) has been compiled in this review. The selection of this period was made to obtain a more realistic view of the current state of this scientific field, particularly concerning BA, considering the current contamination issues. The review provides valuable insights into analytical methodologies, bioparameters, the type of bivalves evaluated, and the trends and variations in human-derived pollutants and toxin levels, algal blooms, and environmental conditions. Additionally, information about the depuration capacity of bivalves, the compliance with legal limits, and a critical point of view of the obtained data will be done.
Analyzed organic pollutants and used analytical techniques
The types of organic pollutants examined in the period 2019–2024 cover a broad spectrum of harmful substances from human and biological sources (see Tables 2 and 3). Concretely, 61% of the 18 published studies in the period 2019–2024 belong to pollutants from human sources, while 39% to marine toxins. Examined human source pollutants include pesticides [11, 39], hormones [40], plasticizers such as BPs [39–46] and phthalates [43], PPCPs [39, 40], PAHs [44, 47], and PFASs [48, 49]. It needs to be remarked that 63% of the works in this part are related to the BA of plasticizers in different molluscs, being, therefore, the most analyzed family. Each article within the reviewed ones provides a detailed examination of different pollutant classes and their prevalence in aquatic environments regarding the BA in bivalves. Additionally, when considering environmental pollutants, we must not overlook MPs, as they can pose a significant and complex problem in the marine environment. Regarding marine toxins, alkaloids [50–53] and polyketides [51, 54] have been the most studied compounds (85% of the published works), being STXs and OA the most explored ones. Nevertheless, kainoids, such as DA [55], or cyclic amines, such as spirolide derivatives [56] have also been evaluated. Both types or organic pollutants are well represented by the different families determined, although other families, such as flame retardants or antibiotics for pollutants coming from human sources, or ciguatoxins and palytoxins for pollutants coming from biological sources, have been not explored in the last years. These founds remark on the importance of expanding these analyses to obtain a holistic overview of the BA of organic pollutants in bivalve species.
Bioaccumulation of pollutants from human sources in bivalve molluscs, type of pollutant, evaluated species, collection place, and calculated biota-sediment accumulation factor (BSAF) and bioconcentration factor (BCF) (when possible) in the period 2019–2024
Type | Pollutant | Evaluated species(sample size) | Location | BA (ng g−1 dw) | BSAF | BCF | Ref. |
---|---|---|---|---|---|---|---|
Pesticides | alpha–BHC | Mytilus edulis (16 mussels) | Martray, France | 0.31a | – | – | [11] |
Charente, France | 0.26a | – | – | ||||
Seudre, France | 0.24a | – | – | ||||
Magallana gigas (21 oysters) | Martray, France | 0.6a | – | – | |||
Charente, France | 0.62a | – | – | ||||
Seudre, France | 0.72a | – | – | ||||
Atrazine | Cerastoderma edule (N.I.Nb of cockles) | Ebro Delta, Spain | 2.64 | – | – | [39] | |
Metolachlor | Crassostrea gigas (N.I.Nb of oysters) | 1.15 | – | – | |||
Herbicides | Bentazone | Crassostrea gigas (N.I.Nb of oysters) | 1.56 (control June) | – | – | ||
0.14 (dead June) | – | – | |||||
0.61 (control Nov) | – | – | |||||
1.24 8 (dead Oct) | – | – | |||||
Cerastoderma edule (N.I.Nb of cockles) | 0.35 (control Dec) | – | – | ||||
5.11 (dead sept) | – | – | |||||
Insecticides | Acetamiprid | Crassostrea gigas (N.I.Nb of oysters) | 3.68 (control May) | – | – | ||
6.71 (dead May) | – | – | |||||
9.51 (control June) | – | – | |||||
Cerastoderma edule (N.I.Nb of cockles) | 3.5 (dead Sept) | – | – | ||||
Hormones | Testosterone | Dreissena bugensis (30 mussels) | Lake Mead Marina, NV, USA | 6.3 | – | – | [40] |
Boulder Island, NV, USA | 12 | – | – | ||||
Las Vegas Bay, NV, USA | 20 | – | – | ||||
EDCs | BPA | Mytilus galloprovincialis (N.I.Nb of mussels in Naples Bay and 20 mussels in Slovenian Sea) | Naples Bay (area A), Italy | 50 | – | – | [41] |
Naples Bay (area B), Italy | 100 | – | – | ||||
Slovenian sea | 0.18 | – | 58 | [42] | |||
Arca noae (15 mussels) | Cabrera Marine Protected Area, Balearic Island, Spain | 0.29a | – | – | [43] | ||
Cerastoderma edule (N.I.Nb of cockles) | Ebro delta, Spain | 4277.4 | – | – | [39] | ||
Dreissena bugensis (30 mussels) | Lake Mead Marina, NV, USA | 47 | – | 39.2 | [40] | ||
Saccostrea sp. (> 30 ostreidae) | Bandar Abbas Coast, Persian Gulf | 340.16 | 0.43 | – | [44] | ||
Rangia cuneata (96 clams) | Vistula Lagoon (Southern Baltic Sea) | 46–288 | 8.68–53.33 | 0.13–1.64 | [46] | ||
BPF | Arca noae (15 mussels) | Cabrera Marine Protected Area, Balearic Island, Spain | 0.29a | – | – | [43] | |
BPS | 0.25a | – | – | ||||
4-t-OP | Rangia cuneata (15 clams) | Vistula Lagoon (Southern Baltic Sea) | 40–111 | 100–185 | 5.13–13.54 | [46] | |
4-NP | 172–528 | 156.36–352 | 7.48–20.30 | ||||
1H–Benzotriazole | Cerastoderma edule (N.I.Nb of cockles) | Ebro Delta, Spain | 2.43 (dead Sept) | – | – | [41] | |
Caffeine | Cerastoderma edule (N.I.Nb of cockles) | 99.85 (dead Sept) | – | – | |||
Ethylparaben | Cerastoderma edule (N.I.Nb of cockles) | 3.56 (control Dec) | – | – | |||
Methylparaben | Crassostrea gigas (N.I.Nb of oysters) | 1.10 (control Nov) | – | – | |||
0.29 (dead Oct) | – | – | |||||
Cerastoderma edule (N.I.Nb of cockles) | 6.69 (control Dec) | – | – | ||||
Propylparaben | Cerastoderma edule (N.I.Nb of cockles) | 0.56 (control Dec) | – | – | |||
Phthalates | DEP | Mytilus galloprovincialis (15 mussels) | Port d’Andratx, Mallorca, Spain | 1.8 | – | – | [43] |
Arca noae (15 mussels) | Cabrera Marine Protected Area, Balearic Island, Spain | 0.54a | – | – | |||
DEHP | 2.58a | – | – | ||||
DBP | 0.78a | – | – | ||||
PPCPs | Triclosan | Dreissena bugensis (30 mussels) | Boulder Island, NV, USA | 24 | – | – | [40] |
Las Vegas Bay, NV, USA | 28 | – | 4.6 | ||||
PAHs | Naphtalene | Saccostrea sp. (Ostreidae)1,2,Circenita callipyga (Veneridae)1, Barbatia helblingii (Arcidae)1, Solen brevis (Solenidae)2, Amiantis umbonella (Veneridae)2, Telescopium telescopium (Potamididae)2(> 30 individuals for each species) | Bandar Lengeh1 and Bandar Abbas2 Coast, Persian Gulf | 2.6–5.10 | 0.60–1.50 | – | [44] |
Acenaphtylene | 0.30–3.20 | 0.50–0.56 | – | ||||
Acenaphthene | 0.40–1.80 | 0.06–0.80 | – | ||||
Fluorene | 1.00–1.60 | 0.20–1.70 | – | ||||
Phenanthrene | 0.60–1.00 | 0.21–2.00 | – | ||||
Anthracene | ND–0.3 | – | – | ||||
Fluoranthene | 0.30–1.40 | 0.18–0.60 | – | ||||
Pyrene | 0.10–2.90 | 0.25–0.30 | – | ||||
Sum (∑) PAHs | Perna perna (N.I.Nb of mussels) | Santos Estuary, Brazil | 96.94–988.76 | – | – | [47] | |
Crassostrea rhizophorae(N.I.Nb of oysters) | 88.38–138.62 | – | – | ||||
Fluorochemicals | ΣPFASs | Crassostrea rhizophorae (14 oysters) | Subaé estuary, Brazil | 0.28a | – | – | [48] |
Mytilus edulis (N.I.Nb of mussels) | Korean coasts | 1.55 | 5.34 | 0.72 | [49] |
EDCs: endocrine-disrupting chemicals; BA: bioaccumulation; a expressed per gram of wet weight; N.I.Nb: not indicated number; alpha–BHC: α-benzohexachloride; BPF: bisphenol F; BPS: bisphenol S; 4-t-OP: 4-tert-octylphenol; 4-NP: 4-nonylphenol; DEP: diethyl phthalate; DEHP: bis(2-ethylhexyl) phthalate; DBP: dibutyl phthalate; PPCPs: pharmaceuticals and personal care products; PAHs: polycyclic aromatic hydrocarbons; 1 Bandar Lengeh; 2 Bandar Abbas; ND: not detected
Bioaccumulation (BA) of marine toxins (pollutants from biological sources) in bivalve molluscs, main producer genera, evaluated species, collection place, and calculated bioconcentration factor (BCF) (when possible) in the period 2019–2024
Type | Toxin | Producer genera | Evaluated species(sample size) | Location | BA (μg kg−1) | BCF | Ref. |
---|---|---|---|---|---|---|---|
Kainoid | DA | Pseudo-nitzchia australis | Donax trunculus (11 clams) | Northwest coast of Brittany, France | 12 × 103 | - | [55] |
Pecten maximus (5 scallops) | 638.6 × 103 | - | |||||
Aequipecten opercularis (10 scallops) | 22.7 × 103 | - | |||||
Crepidula Fornicata (7 slippersnails) | 48.5 × 103 | - | |||||
Polyketide | OA | Vulcanodinium rugosum | Multiple species (1,150) | Galician Coast, Spain | ~100 | - | [56] |
DTX2 | ~150 | - | |||||
OA | Prorocentrum lima | Mytilus galloprovincialis (372 mussels) | Ria of Vigo, Spain | 2,819.2 | - | [54] | |
DTX1 | 1,107.1 | - | |||||
OA | - | Alectryonella plicatula (3 mussels) | - | 255.2 | - | [51] | |
Polyether | YTX | Vulcanodinium rugosum | Multiple species (1,150) | Galician Coast, Spain | ~90 | - | [56] |
Cyclic imine | PnTXG | Vulcanodinium rugosum | ~50 | - | |||
13-desm SPXC | Alexandrium ostenfeldii | ~20 | - | ||||
13-19diDesMetSPXC | ~5 | - | |||||
Alkaloid | STXs | A. catenella | Mytilus edulis (61 mussels) | Coastline of Argentina | 40,432 μg STX di-HCl eq/kg | - | [50] |
Aulacomya atra (2 mussels) | 268 µg STX di-HCl eq/kg | - | |||||
Brachidontes rodriguezii (9 mussels) | 224 µg STX di-HCl eq/kg | - | |||||
Zygochlamys patagonica (23 scallops) | 1,348 µg STX di-HCl eq/kg | - | |||||
Aequipecten tehuelche (13 scallops) | 2,884 µg STX di-HCl eq/kg | - | |||||
Mesodesma mactroides (27 clams) | Mean value < MPL | - | |||||
Ameghinomya antiqua (3 clams) | - | ||||||
Donax hanleyanus (13 clams) | - | ||||||
- | Alectryonella plicatula (3 mussels) | - | 365.3 | - | [51] | ||
Gymnodinium catenatum | Cerastoderme edule (40 cockles) | Mira branch, Aveiro lagoon, Portugal | 125–4,721 μg STX di-HCl eq/kg | - | [52] | ||
Mytilus galloprovincialis (10 mussels) | 165–4,013 μg STX di-HCl eq/kg | - | |||||
Solen marginatus (10 shells) | 133–1,176 μg STX di-HCl eq/kg | - | |||||
- | Mussels (N.I.Nb of not indicated species) | Xiapu, Fujian, China | 0.65 | 282.6 | [53] |
DA: domoic acid; OA: okadaic acid; DTX1: dinophysistoxin 1; YTX: yessotoxin; PnTXG: pinnatoxin G; 13-desm SPXC: 13-desmethyl spirolide C; STX: saxitoxin; N.I.Nb: not indicated number; MPL: maximum permitted level
Accurate measurement of organic pollutants from human and biological sources in bivalve tissues requires sophisticated analytical techniques. The analysis of these substances is not an easy task since tedious and elaborate methodologies are necessary in combination with high-cost instruments that require specialized personnel. The methodologies described in the literature for organic pollutants involve various chromatographic and spectrometric techniques to ensure precise detection and quantification of pollutants. Concretely, liquid chromatography and GC (LC and GC, respectively) tandem mass spectrometry (MS) are the most common techniques for non-volatile and volatile compounds (67%), respectively [11, 39, 42–44, 47–49, 55–54]. However, some authors have opted for the use of fluorescence or ultraviolet detection systems combined with LC [42, 46, 50, 52]. In terms of sample preparation methods, the most common option is liquid extraction using an organic solvent that is placed with the bivalve sample and left to extract for a time in an ultrasonic bath or similar [11, 42, 47, 49]. On the other hand, some authors opted for other sample preparation methodologies, such as conventional solid-phase extraction [42, 46, 50], QuEChERS [39], matrix solid-phase dispersion [43], or the common liquid-liquid extraction [44].
Novel trends in analytical chemistry, such as the use of sensors and green analytical techniques have been scarcely explored in the analysis of pollutants in bivalves. Sensors have appeared in the last years as a novel approach to analyze different substances at low-cost and in an accessible way. A sensor is a system that detects and responds to a physical stimulus or change in its environment. It converts this detected information into a measurable signal, typically an electrical or spectrophotometric one, which can be interpreted by another system or displayed for monitoring [57]. Up to date, the use of sensors as an alternative to conventional methodologies has been not explored to obtain BA information for organic pollutants coming from human sources despite some methodologies that have been already developed [58]. In any case, most of the studies based on the use of sensors are more focused on the analysis of these pollutants in water samples than in other matrices [59]. It is not the case of marine toxins, since there are some authors who have developed sensors to analyze them in bivalves [51, 53]. Yin et al. [51] have developed a dual-emissive fluorescent immunoassay for the monitoring of OA and STX in shellfish using an interesting approach that deserves a deeper explanation since they are able to determine two marine toxins in the same sensor. In this work, the authors used graphene quantum dots doped with sulfur and phosphorous in combination with ovalbumin-coated gold nanoparticles for the synchronous monitoring of both toxins. The sensor was used for a competitive fluorescence-linked immunosorbent assay, which allowed the detection of these two marine toxins in fingerprint oysters (Alectryonella plicatula). A detailed explanation of the sensor fabrication and the detection of the marine toxins is shown in Figure 1.
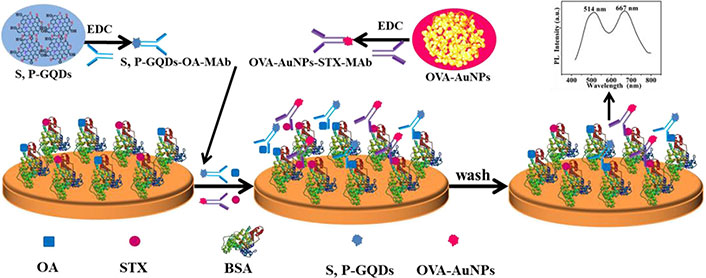
Illustration of the sensor fabrication and the scheme of the competitive fluorescence-linked immunosorbent assay. EDC: endocrine-disrupting chemical; S, P-GQDs: sulfur, phosphorous co-doped graphene quantum dots; MAb: monoclonal antibody; OVA: ovalbumin; AuNPs: gold nanoparticles; STX: saxitoxin; BSA: bovine serum albumin. Reprinted permission from [51], © 2021 Elsevier Ltd
The proposed method showed limits of detections in agreement with the common levels found in molluscs (1.8 μg kg–1 and 0.6 μg kg–1 for OA and STX, respectively), acceptable relative standard deviations (3.3–4.8%), and high specificity compared to other toxins, even from the same family. However, a previous extraction with an organic solvent should be done before the quantification of the analytes [51]. At the end, the sensor was applied to one real sample showing similar results to those found in LC-MS or commercial ELISA methodologies. The development of these determination systems could be a cornerstone in the following years regarding the BA studies on bivalves and other animals that act as marine biomarkers.
BA of organic pollutants in bivalves
The process of BA can be generally defined as the concentration increase of a pollutant in an organism (mainly in tissues and organs), which is exposed to a surrounding environment contaminated with the pollutant. Usually, this parameter is measured by determining the concentration of the pollutant by different analytical techniques in different filter-feeding organisms. However, this parameter is not enough, and inside the BA field, several factors have been defined along the years (Figure 2): (i) bioconcentration factor (BCF); (ii) BA factor (BAF), biota-sediment accumulation factor (BSAF), biomagnification factor (BMF) and trophic magnification factor (TMF) [60].
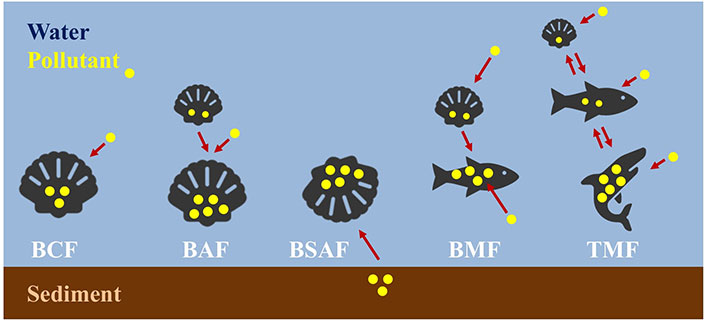
Biaccumulation factors. Scheme of the different bioaccumulation (BA) factors: bioconcentration factor (BCF), BA factor (BAF), biota-sediment accumulation factor (BSAF), biomagnification factor (BMF), and trophic magnification factor (TMF). The arrows indicate the direction in which the pollutants BA
BCF is a value that explains the process by which a chemical substance from the environment is absorbed through several routes (respiratory and dermal), not including the chemical exposure through the diet [61]. Hence, BCF in aquatic organisms mainly proceeds by passive diffusion through gills or epithelial tissues. It is primarily controlled by the physico-chemical properties of the chemicals involved, the physiological disposition of each organism, and the surrounding environmental conditions. Pure BCF can only be measured under controlled laboratory conditions in which dietary intake of the chemical is deliberately not included and is also related to the surrounding water [61]. However, many authors calculate BCF in actual conditions as the concentration of the pollutant in the bivalve divided by the concentration of the pollutant in the surrounding water [62]. Hence, BCF is defined by eq. 1:
where Cpb is the concentration of the pollutant in the organism and Cpw is the concentration of the pollutant in the surrounding water [62]. In this review, the simplified calculation of BCF will be used.
BAF explains the concentration increase of a pollutant in an aquatic organism compared to the global concentration of the pollutant in all surrounding media (water, sediment, diet, etc.) [60]. It can be calculated as BCF, but the denominator (Cpm, see eq. 2) includes in this case the global concentration of the pollutant (water and sediment, and also those obtained from the diet). Some authors also correct Cpb and Cpm by the lipid organism (fL) and global carbon (fC) fractions, respectively, although both eq. 2 and eq. 3 are accepted [62, 63].
Both factors (BCF and BAF) should not be confused, since they are not interchangeable quantities [61]. In any case, it is difficult to evaluate the BAF, since the global concentration of the pollutant studied includes the presence of the pollutant in the dietary intake, which obligates to the measurement of these compounds in the surrounding food.
Another interesting bioparameter related to the accumulation in organisms is the BSAF. BSAF quantifies the BA of sediment-associated pollutants into biota. In the case of lipophilic compounds such as PAHs, the concentration of the pollutant includes all the routes (sediment, water, dietary) in the evaluation. This assumption is explained by the tendency of lipophilic pollutants to aggregate in sediments, where their highest concentration is found. Additionally, this parameter is extremely useful for filter-feeding organisms, such as bivalve molluscs. This can be represented by eq. 4 or eq. 5, depending if the lipid and carbon fractions are taken into account or not [64]:
where Cpb is the chemical concentration in the organism, fL is the lipid fraction of the organism, CS is the chemical concentration in superficial sediment, and FC is the decimal fraction of organic C in the sediment [65]. In general, it is more typical to use eq. 4, but it is important to include information about the fL in the corresponding tables or data [63].
Other bioparameters that can be measured are BMF eq. 6 [66] and TMF. The first one can be defined as the increase in the concentration of a substance in a food chain (not an organism) reaching a maximum value for the highest consumers and can be defined as the ratio of the chemical concentration in a predator organism (Cpred) to that in a prey organism (Cprey).
In the case of TMF, it is increasingly used to quantify biomagnification and represent the average diet-to-consumer transfer of a chemical through food webs. It differs from biomagnification factors, which apply to individual species and can be highly variable between predator-prey combinations. TMF is calculated from the slope of a regression between the chemical concentration and trophic level of organisms in the food web. A deeper explanation of how to calculate the TMF can be found in the literature [67].
The BA of pollutants in bivalves, as mentioned above, is a crucial indicator for assessing the health of aquatic ecosystems. These organisms, which include clams, oysters, and mussels, filter large volumes of water, making them particularly susceptible to the accumulation of toxic substances. Therefore, a summary of the most relevant BA studies of pollutants from human sources is given in Table 2, while the occurrence and BA patterns of marine toxins in bivalves, which are critical for understanding the impacts on marine life and human health are summarized in Table 3.
One significant gap in current research is the insufficient information on the BA factors of pollutants that would enhance our understanding of the potential effects of these compounds on the food web, including both, those from humans or from biological sources. It is crucial to evaluate not only the levels in bivalves but also in the surrounding water and sediments to establish parameters such as BCF, BSAF, and BAF. Interestingly, only one of the articles published during the 2019–2024 period provided data on different BA factors [48], although in some studies (as shown in Tables 2 and 3) it was possible to calculate some of them taking into account the provided data. In this regard, in some of the works, the concentration of pollutants on the water and/or sediments surrounding bivalve habitats is sometimes provided. Hence, crucial parameters such as BCFs and BSAFs are often omitted or not calculated when they can be added to the manuscripts. In this review, Tables 2 and 3 present BCF and BSAF information that has been calculated exclusively for this review, utilizing data available in the reviewed manuscripts that the original authors did not calculate [40, 44, 46, 49]. This lack of comprehensive data hinders our understanding of pollutant dynamics and their potential impacts on marine ecosystems and human health, as it can be understood from the description of the BA factors described before. Despite the low amount of information regarding actual bioparameters, the work conducted by Miranda et al. [48] evaluated TMFs. In this study, the researchers investigated the BA of PFASs (Figure 3) in the Subaé estuarine food web in northeastern Brazil. This comprehensive analysis sheds light on the TMFs of various PFASs, offering critical insights into how these pollutants accumulate and magnify through different trophic levels.
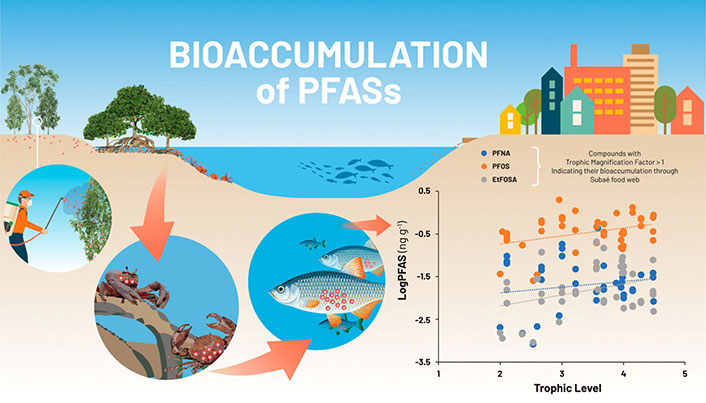
Bioaccumulation of per- and polyfluoroalkyl substances (PFASs) in the food web and calculation of trophic magnification factors (TMFs). PFNA: perfluorononanoate; PFOS: perfluorooctane sulfonate; EtFOS: N-ethyl perfluorooctane sulfonamide. Reprinted permission from [48], © 2020 The Authors
TMFs are calculated to understand the degree of BA and biomagnification of PFASs across different trophic levels within a food web. A TMF greater than 1 indicates that a substance is biomagnifying, while a TMF less than 1 suggests biodilution. In general, TMFs showed values greater than 1, indicating biomagnification across the food web. Nevertheless, some long-chain PFASs, showed biodilution when considering the entire food web. Additionally, the study found significant differences in TMFs when splitting organisms into two groups based on their trophic levels and feeding habits. For instance, lower trophic-level filter-feeding organisms showed higher TMFs for certain compounds compared to higher trophic-level omnivorous organisms. The divergence in TMFs among different PFASs underscores the complexity of BA processes. Therefore, it is important to emphasize the importance of understanding TMFs in assessing the ecological risks associated with pollutant contamination. The identification of specific BA trends of different pollutants will help provide a foundation for better ecological risk assessments and prepare strategies to manage and reduce pollutant exposure in marine ecosystems more adequately.
Another surprising gap is regarding that, recent studies have been primarily focused on free or total toxins, overlooking how these toxins are bound to tissues or within cells, which could offer a deeper insight into toxin BA. Additionally, the formation of metabolites derived from these pollutants may also contribute to understanding toxic effects, underscoring the need to assess all potential forms of these toxins in organisms. A more comprehensive approach is necessary to achieve a realistic understanding and control of pollutant-related issues in marine ecosystems since these toxins can enter the food web.
Selection of species for BA studies
The selection of species for BA studies is critical as it reflects the contamination levels and potential health risks associated with seafood consumption. Various bivalve species are used as bioindicators for pollutants due to their filter-feeding habits, which make them excellent indicators of water quality and pollution levels. In this context, Mytilus galloprovincialis, a mussel from the Mediterranean Sea, and Mytilus edulis, from the North Atlantic Ocean, are two of the most typical species used to measure BA in Europe. Both Mytilus, known as the Mediterranean and common mussels, respectively, are appreciated in Mediterranean and North America gastronomies. Generally, these mussels are consumed due to their delicate flavor and nutritional benefits, being a rich source of protein, omega-3 fatty acids, vitamins, and essential minerals. Both mussels are used in a wide variety of dishes such as stews and paellas and are also usually steamed with herbs and garlic. In terms of biomonitoring, these species are widely used to study the BA of EDCs like pesticides [11], BPs [41–43], phthalates [43, 45], PFASs [49] polyketides [54, 56], polyethers [56], cyclic amines [56] and alkaloids [50, 52]. Its widespread distribution in various marine environments makes it a valuable indicator for monitoring contamination levels and assessing environmental health. Other important bivalve although less studied than the previous ones include the Saccostrea sp. and the Ragia cuneata that has been used to evaluate the BA of PAHs [44], phenols [46], and BPs [44–46]. Other studies have focused on different bivalves depending on the region or ocean, but all of them are generally edible, and only a few are rarely consumed. As deduced from Tables 1 and 2, bivalve species selection for evaluating toxin BA is more often influenced by the location of the study than by specific research objectives. This is primarily due to the proximity of researchers to particular habitats and the relative ease of acquiring these animals for analysis. For instance, in the Mediterranean Sea, notable species include Donax d. trunculus (clam), M. galloprovincialis (blue mussel), and Solen marginatus (shell) [54–56]. The North Atlantic Ocean is home of Pecten p. maximus (scallop), Aequipecten a. opercularis (scallop), and Mytilus edulis (blue mussel), as well as Cerastoderma edule (cockles) [50]. In the South Atlantic Ocean, species such as Zygochlamys patagonica (scallop), Aequipecten tehuelche (scallop), Mesodesma mactroides (clam), and Brachidontes rodriguezii (mussel) are prevalent [50]. The Indian Ocean hosts Aulacomya atra (mussel), A. plicatula, and other bivalves [50, 51]. Finally, the Western Atlantic Ocean includes Crepidula fornicata (slippersnail) [55], highlighting that the location of the study determines the analyzed species. Regarding the overlap between species and regions, it is difficult to establish connections due to the variety of pollutants and analyzed species in the period 2019–2024. In this sense, it is interesting to highlight the works performed on M. galloprovincialis for BPA BA in Naples Bay in Italy [41] and the Slovenian Sea [42]. These studies showed that the BA of BPA is up to 500 times lower in the Slovenian Sea than in Naples Bay. These results are highly connected with the contamination levels in these seas, however, the information about the concentration of BPA in sediments and in the surrounding water is not enough to establish connections.
A comprehensive understanding of BA processes and their potential impacts on human health is crucial to conduct studies on a global scale. By examining a diverse range of bivalve species across different geographic regions, researchers can obtain a more complete picture of how pollutants accumulate and how these dynamics may vary, ultimately enhancing our ability to manage and mitigate associated risks.
It is unfortunate that there is no standardization in the choice of bivalve species used for BA studies. The lack of a uniform approach can lead to variability in the results and make it challenging to compare findings across different studies. Consistency in species selection would enhance the reliability and comparability of data, ultimately improving our understanding of pollutant impacts on marine environments and facilitating more effective environmental management practices. It may be worthwhile to standardize the bivalve species that should be selected as the primary choice for BA studies, as well as their potential effects on human health. In this context, considering the availability and world consumption, it would be interesting to use M. galloprovincialis for the Mediterranean, Mytilus edulis for the North Atlantic, North Pacific and Western Atlantic, Perna perna for the South Atlantic, Mytilus trossulus for the Pacific, and Perna viridis for the Indian Ocean as standardized bioindicators in these regions. However, this does not imply a reduction in the number of studies on other species, as they provide valuable insights into how pollutants travel through the food chain. Nonetheless, establishing a standardization for comparisons between regions would indeed be beneficial.
Relationship between sources and BA and depuration studies
The relationship between human activities and BA of pollutants from human sources in bivalves is discussed in some articles [40, 44]. Various anthropogenic sources contribute to the contamination of aquatic environments, leading to the accumulation of harmful substances in bivalves. For example, the study conducted by Bai et al. [40] reveals significant relationships between human pollution and BA in bivalves and other marine species. In Lake Mead, in the USA, human activities, particularly wastewater effluents and recreational activities, introduce EDCs and PPCPs into the water. These pollutants are more persistent in deeper water, affecting bottom-dwelling organisms like quagga mussels, which are used as bioindicators. The pollutants’ presence in these mussels signals potential BA risks for other aquatic species and even humans. Persistent pollutants such as BPA, salicylic acid, and triclosan demonstrate the need for comprehensive monitoring and ecological impact assessments to mitigate risks to marine ecosystems and human health.
An important characteristic of bivalves is depuration, which is the natural process by which bivalves cleanse themselves of accumulated pollutants when placed in toxin-free water. This capacity of bivalves ensures the safety of seafood before entering into the food web. Hence, depuration studies are essential for understanding how bivalves bioaccumulate and release pollutants. However, in the period 2019–2024, BA research often overlooks the critical aspect of these studies, at least for pollutants coming from human sources. On the other hand, depuration studies for marine toxins have been performed in the work developed by Freitas et al. [52], who studied the level of paralytic shellfish toxins (PSTs) in cockles (C. edule), razor shells (S. marginatus) and mussels (M. galloprovincialis). In this work, the authors explored how the levels of PSTs in the different molluscs change along four months, week by week, in Portugal, and how this is related to the algal blooms. The results can be summarized in Figure 4 where the maximum amount of PSTs was found after one week of the algal bloom (1,176–4,721 µg kg–1) and systematically decreased week-by-week until the middle of February. In this second concentration increase, a second small algal bloom occurred. Afterward, the amount of PSTs decreased until reaching a plateau at low levels (125–165 µg kg–1).
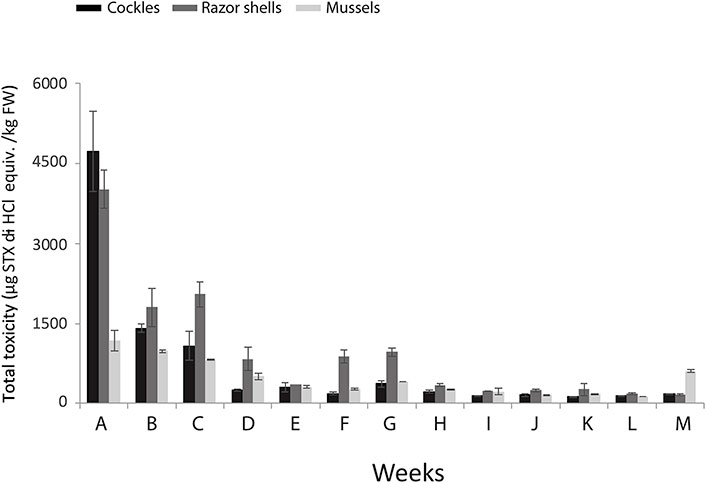
Toxins content. Total amount of paralytic shellfish toxins (PSTs, μg kg–1) in cockles (Cerastoderma edule, black columns), razor shells (Solen marginatus, dark grey columns) and mussels (Mytilus galloprovincialis, light grey columns), collected weekly from 9th January to April 3, 2017 (weeks A to M) in the Mira branch, Aveiro lagoon, Portugal; mean values (n = 3) ± SD. STX: saxitoxin; FW: fish weight. Reprinted permission from [52], © 2020 Elsevier Inc
The findings revealed noteworthy insights, including the activation of antioxidant mechanisms by the species to counteract cellular damage and sustain redox balance. Additionally, at higher toxin concentrations, neurotoxic effects were observed, leading to a reduction in the metabolic activity of bivalves and a decrease in energy expenditure. These physiological changes could significantly impact the overall performance of the bivalves, as their energy reserves were likely diverted towards defensive responses against toxin-induced effects, rather than supporting growth or reproductive functions. Despite these intriguing results, the absence of analysis of the surrounding water makes it challenging to fully understand the dynamics of detoxification and BA, as well as how these processes interconnect. A comprehensive evaluation of the surrounding water’s role would be crucial for a complete understanding of these biological interactions.
This oversight is concerning because understanding depuration in consonance with BA is essential for assessing the full environmental and health impacts of pollutants. Without these studies, it is not possible to accurately evaluate the long-term risks associated with bioaccumulated pollutants and avoid the possibility of developing effective strategies to mitigate their presence in ecosystems and food webs. Consequently, incorporating depuration studies into BA research is crucial for a comprehensive understanding of pollutant dynamics and for safeguarding environmental and public health.
Another important aspect that should not be underestimated in BA studies is the interaction between different pollutants, as they rarely exist in isolation in the environment. In this regard, the interactions among various pollutants have been scarcely studied. Notably, one significant study conducted by Rios-Fuster et al. [43] between 2019 and 2024 addresses this gap in the literature. This study provides a comprehensive analysis of MP ingestion and the accumulation of plasticizers, BPs, and phthalates in bivalves among other marine species collected from Cabrera Marine Protected Area in the western Mediterranean Sea in Spain. In this work, the authors found that sediment-feeding species are particularly vulnerable to MP ingestion due to their direct interaction with sediments containing high concentrations of MPs. Additionally, the results effectively demonstrate the importance of analyzing the BA of both MPs and their associated plasticizers in marine organisms. The differential ingestion rates and accumulation patterns observed among bivalves and other marine species highlight the need for comprehensive pollution assessments that consider the interactions between MPs and chemical pollutants. The observed high negative correlation between the number of MPs and BPA levels highlights the complex interactions between these pollutants, similar to other findings in Mediterranean mussels [68].
Ensuring that the pollutant levels in bivalves comply with legal limits is vital for public health and environmental management. Therefore, various regulatory bodies have established maximum permitted levels (MPLs) for different pollutants in seafood (few pesticides, few PFASs, and PAHs) to protect consumers, such as the European Commission Regulation (EC) No 396/2005 [69] and No 2019/1021 [70]. However, there is a lack of limits in molluscs, specifically for most of the studied pollutants, such as atrazine, metoachlor, testosterone, BPs, phenols, parabens, phthalates, or triclosan. In this sense, some articles have included studies regarding the comparison of the actual levels of pollutants to those recommended as maximum by several government organizations. As an example, studies of mussels farmed in polluted areas like Naples Bay, in Italy [41], have demonstrated that these bivalves can contain BPA levels exceeding the legal limits for human consumption in Italy, posing serious health risks. Similarly, a study of phenol derivatives in bivalves from Vistula Lagoon (Southern Baltic Sea) demonstrated that even if their content was high, it did not surpass the thresholds known to cause adverse effects. However, the potential for synergistic or additive effects of EDCs is still worrying [46]. In another work, it was demonstrated that the environmental risks of PAHSs in the Hormozgan province coastline (Persian Gulf), were deemed low, although it is crucial to recognize that these assessments, such as toxic equivalent quantity, often underestimate long-term and combined effects [44]. Despite the observed low carcinogenic risk from benzo[a]pyrene in bivalves, the cumulative impact of multiple PAHs on human health necessitates stricter regulatory measures [47].
On the other hand, assessing whether the marine toxin levels in bivalves comply with legal limits is vital for ensuring food safety. Some of the reviewed articles provide detailed information on compliance with regulatory standards. In this sense, Rossignoli et al. [56] indicated that, in general, the different bivalves analyzed in Galicia, Spain, are under the EU legal limits of OA (332 μg kg–1, respectively). In the case of STX, 75 μg kg–1 has been suggested by the European Food Safety Authority (EFSA), and the results found by Jin et al. [53] showed that the method is capable of achieving the adequate limits of detection, being the content determined in the analyzed mussels under the maximum allowed concentration. Other indications for seafood established the maximum permitted concentration levels in 160 μg kg–1 and 800 μg kg–1 for OA and STX, respectively [51]. In any case, the studies included in this review showed that the levels of pollutants (Table 3) are low, except after algal blooms where maximum peaks of concentration in bivalves appeared. Therefore, it is important to control these dynamics to avoid undesirable effects, such as possible intoxications in humans after consumption of contaminated bivalves [71, 72].
Environmental conditions
Understanding and considering environmental conditions is crucial when examining BA processes because these factors directly influence the rate and extent to which pollutants accumulate in organisms. Variations in temperature, pH, salinity, and oxygen levels, among others, can affect the bioavailability of pollutants, altering their uptake by organisms. A similar trend can be observed for marine toxins, several articles reviewed in this work delve into the complex relationship between algal blooms, environmental conditions, and their BA in bivalves. Key factors such as pH, temperature, and other abiotic conditions significantly influence toxin dynamics and the extent of their BA in bivalves. For example, it is highly known that algal blooms are the primary sources of marine biotoxins, as previously mentioned. Therefore, the density and duration of these blooms directly correlate with the levels of toxins accumulated in bivalve tissues. The role of environmental conditions on BA is a critical factor that affects the dynamics of algal blooms and bivalve feeding. In this context, high temperatures can enhance the rate of pollutant uptake in bivalves due to the increase in the metabolic rates of both algae and bivalves, leading to more rapid toxin production and pollutant accumulation [54, 73]. Despite the fact that water pH plays a significant role in toxin dynamics by influencing the solubility and stability of toxins, none of the analyzed studies has explored this variable. Additionally, the pH of the seawater surrounding the bivalves was not determined in these studies. Similar trends were observed in the only study that measures salinity [52], although dissolved oxygen was not measured in any of the works. Taking into account that salinity impacts the physiology of both algae and bivalves and oxygen levels in water influence algal bloom sustainability and toxin bioavailability, it is important to provide these data.
A comprehensive understanding of these interactions is vital for assessing environmental risks and implementing effective regulatory strategies to protect both ecosystems and human health. However, most studies generally fail to provide this information comprehensively, making it challenging to fully understand the dynamics of pollutant BA in molluscs. In this sense, it has been demonstrated that factors like salinity and pH affect the sorption/desorption rates of chemicals from MPs to seawater, influencing BA in marine organisms [68]. Hence, addressing these combined effects is crucial for understanding the full impact of plastic pollution on marine ecosystems and for developing effective strategies to mitigate environmental and health risks.
Conclusions
This comprehensive review examines the BA of environmental pollutants and marine toxins in bivalve molluscs, offering significant insights into the current state of research and identifying future directions. The major findings and conclusions drawn from this review are summarized below.
Bivalve molluscs are exposed to a wide variety of pollutants, as evidenced by the numerous articles examined in this review. These pollutants include POPs, EDCs, PPCPs, pesticides, MPs, and various marine toxins, such as alkaloids and polyketides, among others. Among these pollutants, plasticizers have been the most studied ones. The analysis of these compounds in bivalve tissues is predominantly conducted using LC-MS. Despite this, novel trends in analytical chemistry are transforming the landscape with the introduction of other innovative methodologies, such as the use of sensors to measure the concentration of these compounds in bivalves. These sensors facilitate the characterization of important bioparameters. In the future, it is expected to use more sensors that facilitate the analysis of BA increasing the number of publications. This will provide more data and a better understanding of the BA dynamics.
The review also highlights the mechanisms of BA in bivalves, emphasizing the roles of bioparameters such as BCF, BAF, BSAF, BMF, and TMF. Understanding these mechanisms is crucial for comprehending how pollutants transfer and concentrate within marine food webs, ultimately impacting human health through seafood consumption. However, despite the relevance of the reviewed studies, many authors avoid calculating these important bioparameters. This limitation hinders the establishment of correlations or predictions that could better control the presence of these compounds in the food web. Nevertheless, some studies have successfully established significant findings using appropriate bioparameters such as TMF. In this case, it should be noted the importance of incorporating these parameters in future studies to increase the BA knowledge of the scientific community.
The consumption of contaminated bivalves can lead to various health issues, as discussed in the manuscript, including gastrointestinal disorders, neurological problems, and in extreme cases, death. Marine toxins, such as DA and STXs, are particularly concerning due to their acute toxic effects and potential to cause widespread intoxication incidents. Therefore, an adequate study of these harmful substances’ bioparameters is crucial for monitoring pollution levels, assessing environmental health, and ensuring seafood safety. However, achieving this is challenging due to the need for standardized protocols to facilitate comparisons across works and regions. In this regard, the review showed that there is a wide variety of methods and molluscs analyzed even in the same location. Therefore, it is important to establish common protocols, such as using similar organisms for comparison. In this sense, we recommend using specific species for each world region as standardized bioindicators. In any case, we also recommend continuously expanding the analyzed species to obtain valuable insights about BA in the food web.
As shown in this review, environmental conditions such as rising seawater temperatures, changes in salinity, nutrient availability, and ocean acidification significantly influence the BA of pollutants. These factors can also affect the prevalence and toxicity of harmful algal blooms, which produce marine toxins that accumulate in bivalves and pose severe health risks to humans.
Moreover, long-term monitoring data and depuration information are limited, making it challenging to assess trends over time and the effectiveness of pollution control measures. The most problematic aspect is the complex and not fully understood interactions between different pollutants and environmental factors. Hence, more research is needed to elucidate these interactions and their implications for BA and toxicity in bivalves to better assess environmental health and ensure seafood safety.
In the end, all these gaps in the BA studies underscore the urgent need for comprehensive monitoring and evaluation of bioaccumulated pollutants, including BA factors, interactions, synergic effects, etc., in order to protect both environmental and public health.
In terms of future trends in this field, it is important to develop integrated monitoring programs that combine chemical analysis, biological assessment, standardized protocols, and organisms and environmental parameters to provide a holistic understanding of pollution impacts. Additionally, the development of novel in vitro methodologies capable of predicting various bioparameters should be prioritized to obtain more reliable information without ethical concerns. However, the cornerstone of this field should be based on the extensive and thorough evaluation of bioparameters to establish global trends.
Abbreviations
ASP: | amnesic shellfish poisoning |
AZP: | azaspiracid poisoning |
BA: | bioaccumulation |
BAF: | bioaccumulation factor |
BCF: | bioconcentration factor |
BMF: | biomagnification factor |
BPA: | bisphenol A |
BPs: | bisphenols |
BSAF: | biota-sediment accumulation factor |
DA: | domoic acid |
DSP: | diarrheic shellfish poisoning |
EDCs: | endocrine-disrupting chemicals |
GC: | gas chromatography |
LC: | liquid chromatography |
MPs: | microplastics |
MS: | mass spectrometry |
MW: | molecular weight |
NSP: | neurotoxic shellfish poisoning |
OA: | okadaic acid |
PAHs: | polycyclic aromatic hydrocarbons |
PFASs: | per- and polyfluoroalkyl substances |
POPs: | persistent organic pollutants |
PPCPs: | pharmaceuticals and personal care products |
PSP: | paralytic shellfish poisoning |
PST: | paralytic shellfish toxin |
STX: | saxitoxin |
TMF: | trophic magnification factor |
Declarations
Author contributions
COE: Investigation, Writing—original draft. ARC: Investigation, Writing—original draft. EJCC and MJLG: Writing—original draft, Conceptualization, Writing—review & editing, Supervision, Funding acquisition.
Conflicts of interest
The authors declare that they have no conflicts of interest.
Ethical approval
Not applicable.
Consent to participate
Not applicable.
Consent to publication
Not applicable.
Availability of data and materials
Not applicable.
Funding
The authors acknowledge financial support from the Spanish State Research Agency [AEI//10.13039/501100011033/], the Spanish Ministry of Science, Innovation and Universities (MICIU), and the European Union (NextGenerationEU/PRTR) through the project [PID2021-125459OB-I00]. Also, MJ Lerma-García acknowledges the project [CIAICO/2022/183] funded by Generalitat Valenciana. E.J. Carrasco-Correa also acknowledges projects [PID2020-117686RB-C33] and [TED2021-131303B-I00] from AEI and MICIU and [CIGE/2023/069] from Generalitat Valenciana. The funders had no role in study design, data collection and analysis, decision to publish, or preparation of the manuscript.
Copyright
© The Author(s) 2024.