Abstract
Aim:
Non-healing wounds are one of the most substantial and difficult problems to treat. Wound healing involves a sequence of complex biological processes, but often the oral cavity microbiota adversely affects healing and forms a chronic non-healing wound.
Methods:
In this study, a biologically active membrane (BAM) is present, consisting of decellularized human amniotic membrane and bone marrow stem cells (BMSCs). The efficacy of BAM was evaluated in a model of non-healing oral wounds in rats with streptozotocin (STZ)-induced diabetes mellitus.
Results:
Studies have shown that BAM enhanced the healing of chronic oral wounds in animals with induced diabetes mellitus, reduced scarring, and reduced risk of infection. Paracrine freeze-dried BMSCs stimulated angiogenesis and improved wound conditions.
Conclusions:
BMSCs may lower glucose levels in rats with STZ-induced diabetes mellitus and improve the healing process of chronic diseases. However, more studies are needed to study the paracrine factors of BMSCs and their role in the treatment of non-healing wounds.
Keywords
Chronic non-healing wounds, stem cells, amniotic membrane, oral cavity, diabetes mellitusIntroduction
Chronic, non-healing wounds are a common problem that can occur for a variety of reasons, including chronic systemic disease, circulatory problems, and a weakened immune system. In the USA, it is reported that chronic wounds affect approximately 6.5 million patients [1].
Chronic non-healing wounds are wounds that do not heal for several weeks despite standard treatment [2, 3]. As is known, wound healing involves a sequence of complex biological processes and can often be surrounded by a hostile environment that adversely affects healing and forms a chronic non-healing wound. Pain caused by chronic oral wounds requires constant treatment and care, which is time-consuming for patients and healthcare workers, and increases healthcare costs [4, 5]. Non-healing wounds, as well as oral ulcers, can occur as a result of various systemic diseases. For example, elevated blood glucose levels in diabetes mellitus can lead to the formation of chronic wounds and oral ulcers [6–8]. Dry mouth (xerostomia), caries (including root caries), periapical lesions, gingivitis, periodontal disease, oral candidiasis, burning mouth (especially glossodynia), oral lichen planus (OLP), recurrent aphthous stomatitis in patients with diabetes mellitus can cause the development of chronic oral wounds and complicate the process of their healing [9–14]. Thus, patients with diabetes are at a higher risk of developing non-healing oral wounds.
Currently, various methods are used for the treatment of non-healing oral wounds in patients with diabetes mellitus, including blood glucose control, wound debridement, periodontal surgery (flap surgery or gingivectomy) [15], hyperbaric oxygen therapy, various dressings for wounds, which may be loaded with antimicrobial agents, growth factors, or other substances that promote wound healing [16–21]. Each of these methods has its own advantages and disadvantages. However, our attention was drawn to the human amniotic membrane (HAM), which is effectively used in tissue engineering and regenerative medicine. HAM contains growth factors and cytokines that can stimulate the growth of new tissues and blood vessels, which can lead to faster wound healing. HAM also contains several anti-inflammatory cytokines that can reduce inflammation and create a more conducive environment for wound healing. Additionally, HAM is biocompatible, which will not elicit an immune response when used for wound healing. In recent years, HAM has been reported to be used for promoting anterior auricle reconstruction [22, 23], as an allograft in hand surgery [24], for ocular surface disorders [25–27], for repair of injured common peroneal nerve [28] and others. There are reports of the use of cryo-preserved and decellularized HAM (dHAM) grafts in the therapy of chronic non-healing wounds [29–31]. We have reported the use of HAM in the clinic in a patient for closing post-laryngectomy of pharyngocutaneous fistulas [32]. We also have used HAM in preclinical studies for the treatment of non-healing wounds after radiation therapy [33].
In this study, we are presenting a biologically active membrane (BAM) that we developed, consisting of dHAM and bone marrow stem cells (BMSCs). The efficacy of BAM was evaluated in a model of non-healing oral wounds in rats with induced diabetes mellitus.
Materials and methods
Preparation of decellularized and lyophilized HAM
The amniotic membrane was obtained from the human placenta in accordance with our previously described method [34]. Briefly, the study was conducted on 2 full-term placentas from donors who signed an informed consent form and delivered at 37–41 weeks of gestation. This research is permitted by the ethics committee of the Tbilisi State Medical University (Tbilisi, Georgia; #128/02). All donors had normal pregnancies and gave birth to healthy newborns weighing from 2,300 g to 3,900 g. Newly obtained placentas were washed with 0.9% saline and heparin at 37°C under physiological pressure through the umbilical arteries and veins. After washing, the placenta was frozen at –80°C. Frozen placentas were thawed at 40°C and washed with phosphate-buffered saline (PBS, Sigma) overnight by perfusion through the placental arteries. Placental decellularization was perfused with sodium dodecyl sulfate (SDS, Sigma) in distilled water for 72 h, starting with 0.01% SDS for 24 h, then 0.1% SDS for 24 h, and 1% SDS for 24 h. Placentas were then washed with distilled water for 15 min and 1% Triton X-100 (Sigma) for 30 min to remove residual SDS. After decellularization of the placenta, the amniotic membrane was excised, cut into small 3 cm × 3 cm flaps, and treated with 10% fetal bovine serum (FBS, Sigma), 1% streptomycin (Sigma). After that, the amniotic membrane flaps were placed in a lyophilization apparatus (PowerDry PL6000 Freeze Dryers, China). Lyophilized amniotic membranes were stored under serial conditions until use.
Fabrication of BAM from dHAM and BMSCs
Five laboratory Lewis rats of both sexes weighing from 200 g to 250 g were used to obtain BMSCs [33, 34]. The Ethics Committee of the Georgian National Institute of Medical Research approved the animal protocols (permit 22-14). Animals were euthanized by lethal injection of 0.5% sodium thiopental solution (Sigma, St. Louis, MO, USA). The lower limbs were amputated after treatment with a 70% alcohol solution. The thighs, cleared of muscle tissue, were resected in the area of the epiphysis and diaphysis. A needle was inserted into the lumen of the bone canal and the bone marrow was eluted using a syringe with Dulbecco’s modified eagle’s medium (Sigma). The mononuclear fraction was isolated by density gradient centrifugation at 400 g for 30 min at room temperature using Ficoll Paque Plus or Ficoll Paque Premium solution (GE Healthcare Bio-Sciences, Pittsburgh, PA, USA). After washing with PBS, the cells were centrifuged at 200 g for 5 min. Some of the cells were dissolved in 1 mL of PBS and then, they were placed in a Neubauer chamber, and viability was determined using trypan blue (Sigma).
Thereafter, the decellularized and lyophilized HAM was placed in a sterile PBS solution for 30 min. Amniotic membranes washed in PBS solution were foamed into Petri dishes filled with RPMI 1640 medium (Sigma-Aldrich; EMD Millipore, St. Louis, Missouri, USA), and their surface was loaded with 2.5 × 106 mononuclear stem cells. After 15 min, the amniotic membrane was turned over and the same number of cells was seeded on the opposite side. To ensure uniform cell distribution, this process was repeated every 15 min for 1 h. Further, amniotic membranes loaded with BMSCs were cultured in a humidified incubator (37°C, 5% CO2) for 4 days in a medium containing Dulbecco’s modified eagle’s low glucose medium (Sigma-Aldrich; EMD Millipore) supplemented with 10% FBS (Sigma-Aldrich; EMD Millipore) and antibiotics (100 U/mL penicillin G and 0.1 mg/mL streptomycin; Invitrogen, Carlsbad, CA, USA). After incubation, an HAM loaded with BMSCs was used to close a rat oral wound model.
Experiment design
Animals
In total, 50 Lewis laboratory rats (8 weeks old, weight 200–250 g) were used to create a model of a non-healing oral wound. The animals were purchased from the vivarium of the Tbilisi State Medical University (Tbilisi, Georgia). Rats were kept under controlled conditions at 24°C ± 2°C with a 12-h light-dark cycle and with free access to water and food.
The studies were carried out in accordance with the recommendations of the Committee for the Care and Use of Animals of the Tbilisi State Medical University. A recognized institutional review board at the Tbilisi State Medical University approved all animal protocols.
Diabetes induction
Animals acclimatized for 1 week prior to induction of diabetes and were divided into 3 groups. In the animals of the first and second groups, diabetes was induced by intraperitoneal injection of 65 mg/kg streptozotocin (STZ; Sigma, Steinheim, Germany). Only the animals with blood glucose levels ≥ 200 mg/dL were included in the study.
Oral wound model and treatment
After 15 days, a rounded 8 mm diameter wound was formed on the gingival surface of the molars in all animals using scissors (the area of the wound was ~50 mm2). In the animals of the first group (n = 15) that had induced diabetes, the gingival defect was closed with a 12 mm diameter BAM, which was fixed with atraumatic sutures (7/0, Ethicon). Animals of the second group (n = 15), which also had induced diabetes with gingival defects, were observed without treatment. Surgical manipulations were performed using microsurgical techniques and an operating microscope (Zeiss, Germany). Animals of the third (control) group (n = 15) were intraperitoneally injected with citrate buffer and a gingival defect was formed. Animals of the third group were observed and received no treatment. The remaining animals (n = 5) served as donors for obtaining BMSCs. All manipulations were performed under anesthesia by intraperitoneal administration of ketamine (60–100 mg/kg) and xylazine (8–10 mg/kg).
In the postoperative period, all animals were observed under standard vivarium conditions. Blood glucose levels were measured daily during the first week after induction, then twice a week until the end of the study. Animals were weighed at various times both before and after diabetes induction.
The rats were euthanized at days 3, 9, 15, 25, and 40, using a combination of intraperitoneal injection of ketamine and xylazine, with subsequent laparotomy, aorta rupture, and exsanguinations. At autopsy, the area of the wound was measured with a caliper and calculated by the formula: S = πd2/4 (S: wound area; d: wound diameter).
Histological examination
The native and BAM samples, including surrounding tissues around the wound, were excised using sterile surgical scissors at various times after the operation and fixed overnight in 10% buffered formalin and then, embedded in paraffin and sectioned at 3 µm. Sections were stained with hematoxylin and eosin and Masson’s trichrome. Histological preparations were analyzed using a light microscope (E100; Nikon Corporation, Tokyo, Japan) and a stereoscopic microscope (MBS-9; Lomo, St. Petersburg, Russia).
Scanning electron microscopy
Scanning electron microscopy was used to assess the structure of the dHAM. To do this, the amniotic membrane, both before and after decellularization, was dehydrated by treating them with an ethanol solution before drying with a Tousimis Samdri-780 Critical Point Dryer (Tousimis Research Corporation, Rockville, MD, USA). After drying was complete, all tissues were lightly gold-coated and visualized with a JEOL JSM-65 10 scanning electron microscope (JEOL Ltd, Tokyo, Japan).
Results
All animals tolerated the operation and postoperative treatment well. The conducted studies showed that compared with the animals of the first and control groups, the animals of the second group had a significant delay in wound healing. Studies have shown that in animals of the control and first groups, the wound healed completely on days 8–10 after modeling, as evidenced by measurements of the area of the wounds (Figure 1). Animals of the second group showed a significant decrease in the rate of wound healing. Blood glucose level after modeling diabetes mellitus is shown in Figure 2. It should also be noted that, in relation to the initial values, the loss of body weight was observed both in the animals of the first and in the animals of the second groups. In the first three days, in most animals, BAM adhered tightly to the surface of the wound. However, in some animals of the first group, suture divergences were noted. Histological studies showed edema and cell infiltration of the wound area on days 2–3 from modeling. At the same time, in the animals of the second group, edema of the surrounding tissues with exudative inflammation was noted. Necrotic tissues with purulent inflammation were determined in the wound. In the following days, the wound took on a chronic course. In the animals of the second group, complete healing of the wound was observed on the 25–30th day from modeling. In the animals of the first group, on the third day, the edges of the membrane were raised, and tender granulation tissue was already determined in the wound. At the same time, the process of BAM delamination was observed. One part of the one that faced BAM oral cavity was rejected, and the other part was involved in the re-epithelialization of the wound. On the fifth day, the formation of new vessels and cellular elements of the gingival epithelium was observed in the wound. On days 10–12, the wound was closed, and the newly formed tissue did not differ from the norm in shape and color (Figure 3). There was also a thickening of the epithelial layer of the gums with mild keratinization. A similar picture was observed in the animals of the third group, but their wound healed on days 8–10.
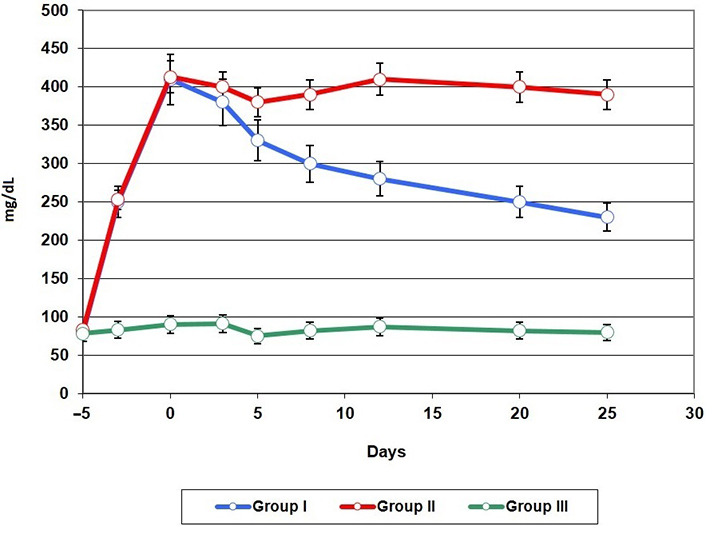
Blood glucose levels after induction of diabetes mellitus and initiation of treatment
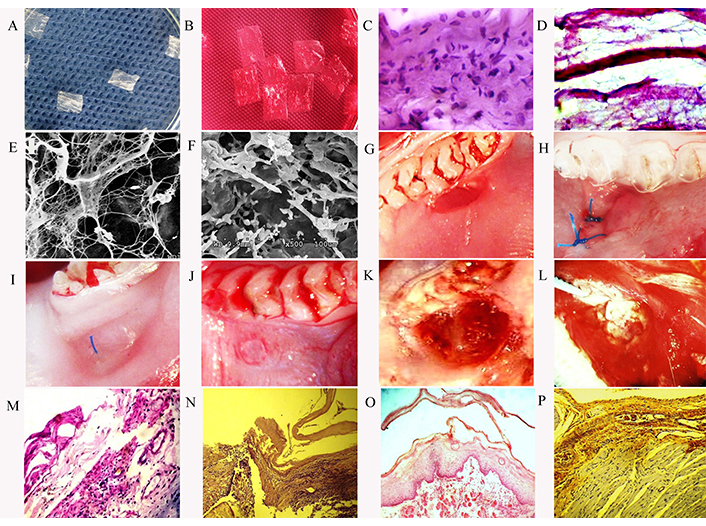
Oral wound model and treatment methods. (A) dHAM; (B) dHAM loaded with BMSCs and placed in Dulbecco’s modified eagle’s low glucose medium; (C) HAM before decellularization. Staining HE, ×400; (D) HAM after decellularization. Staining hematoxylin with eosin ×400; (E) and (F) scanning electron microscopy of dHAM before and after loading of BMSCs; (G) mouth wound model; (H) the wound is covered with BAM. The observation period is 3 days; (I) wound healing process. The observation period is 5 days; (J) BAM is determined in the wound. The observation period is 8 days; (K) and (L) chronic wound of the oral cavity of animals of the second group with induced diabetes mellitus without treatment. The observation period is 8 and 14 days; (M) leukocyte infiltration of the wound with purulent exudate. Chronic wound of the oral cavity of animals of the second group with induced diabetes mellitus without treatment. Stained with HE, ×400. Observation period is 8 days; (N) the wound is covered with BAM. The observation period is 3 days; stained with HE, ×200; (O) process of bundle BAM. The observation period is 5 days; stained with HE, ×200; (P) in animals of the first group, the wound healed without complications. The observation period is 12 days; stained with HE, ×200
Discussion
Non-healed wounds are the result of a complex interaction of biological and environmental factors. A cascade of events involving various signaling pathways, growth factors, and extracellular matrix (ECM) components regulates the healing process. One of the main factors contributing to the development of a long-term non-healing wound is a violation of angiogenesis, which may be associated with a decrease in the level of growth factors such as vascular endothelial growth factor (VEGF) and basic fibroblast growth factor (bFGF). Another important factor is chronic inflammation, which persists in chronic wounds for a long period, leading to increased levels of pro-inflammatory cytokines and matrix metalloproteinases (MMPs). These molecules can degrade the ECM, interfere with cell migration, and inhibit angiogenesis. In addition, a factor contributing to the development of non-healing wounds is violation of the structure of the ECM. ECM provides structural support to cells and tissues and plays an important role in the regulation of cellular behavior. In non-healing wounds, the ECM often changes due to the overproduction of MMPs, which can degrade collagen and other matrix components. When treating a non-healing wound, it is important to understand the involved molecular mechanisms and to consider the underlying factors that contribute to healing impairment. Our studies have shown that compared to animals of the first and control groups, in the animals of the second group, in which the animals with diabetes mellitus received no treatment, there was a significant delay in wound healing. In the animals of the second group, edema of the surrounding tissues was simultaneously noted with exudative inflammation. Necrotic tissues with purulent inflammation were determined in the wound. In the following days, the wound took on a chronic course. On the 25th–30th day from modeling, complete wound healing was observed in the animals of the second group. Studies have also shown that, in animals of the control and first groups, the wounds completely healed on days 8–10 after modeling. In animals of the first group, on the third day, the edges of the membrane were raised, and tender granulation tissue was already determined in the wound. In this case, the process of BAM stratification was observed. One part of the BAM facing the oral cavity was rejected, while the other part participated in the re-epithelialization of the wound. On the fifth day, the formation of new vessels and cellular elements of the gingival epithelium was observed in the wound.
It is necessary to note the level of glucose in the blood of animals after treatment. As our studies have shown, after the application of BAM with loaded BMSCs, there was a decrease in the blood glucose level in the animals of the first group. We think that this was due to the paracrine factors of BMSCs. We agree with the authors that mesenchymal stem cells (MSCs) have differentiation potential, immunosuppressive properties, and anti-inflammatory effects, and are considered an ideal candidate cell type for the treatment of diabetes mellitus [35].
The authors report that MSC therapy of STZ-induced diabetic developing rats during an early stage has the capacity of β cell restoration and the control of blood glycemic homeostasis [36].
A preclinical study for the treatment of diabetes mellitus using β-like cells derived from human dental pulp stem cells has also been reported [37]. It is interesting to note that early intervention with MSCs prevented renal injury via immune regulation in diabetic rats, which restored the homeostasis of the immune microenvironment, contributing to the prevention of kidney dysfunction and glomerulosclerosis [38].
Thus, the results of the study showed that BAM improved the healing of chronic oral wounds in animals with induced diabetes mellitus, reduced scarring and risk of infection. Paracrine freeze-dried BMSCs stimulated angiogenesis and improved wound conditions. In addition, BMSCs may lower glucose levels in diabetics and improve the healing process of chronic diseases. However, more studies are needed to study the paracrine factors of BMSCs and their role in the treatment of non-healing wounds.
Abbreviations
BAM: | biologically active membrane |
BMSCs: | bone marrow stem cells |
dHAM: | decellularized human amniotic membrane |
ECM: | extracellular matrix |
HAM: | human amniotic membrane |
MSCs: | mesenchymal stem cells |
PBS: | phosphate-buffered saline |
SDS: | sodium dodecyl sulfate |
STZ: | streptozotocin |
Declarations
Author contributions
LK: Conceptualization, Investigation, Writing—original draft, Project administration, Methodology, Data curation, Validation. ZK: Conceptualization, Investigation, Writing—original draft, Writing—review & editing, Validation. DC: Writing—review & editing, Supervision. MK and TP: Resources, Data curation. All authors read and approved the submitted version.
Conflicts of interest
The authors declare that they have no conflicts of interest.
Ethical approval
Preparation of decellularized and lyophilized human amniotic membrane is approved by ethics committee of the Tbilisi State Medical University (Tbilisi, Georgia; #128/02). The Ethics Committee of the Georgian National Institute of Medical Research approved the animal protocols (Permit 22-14). The study is conducted in accordance with the Declaration of Helsinki.
Consent to participate
Informed consent to participate in the study was obtained from all participants.
Consent to publication
No applicable.
Availability of data and materials
All datasets generated for this study are included in the manuscript.
Funding
Not applicable.
Copyright
© The Author(s) 2023.