Abstract
Aim:
The clinical symptoms of autoantibody (AAb)-mediated autoimmune diseases (AID) usually correlate with the AAb-titer. Immunoglobulins (Igs) of the IgG type are actively recycled by the neonatal crystallizable fragment receptor (FcRn). The most common Ig type of AAb is IgG. This explorative study evaluates the safety and tolerability of a fully human anti-FcRn monoclonal antibody (mAb) in patients with thyroid autoimmunity (TA).
Methods:
Adverse events (AEs) and serious AEs (SAEs) were documented and coded according to the standardized Medical Dictionary for Regulatory Activities (MedDRA). AEs were followed up, and seriousness, as defined by the International Conference on Harmonization of Technical Requirements for Registration of Pharmaceuticals for Human Use (ICH)-guideline E6, was documented. All AEs were analyzed for a possible underlying cause, and if not identified, were graded as side effects (SEs). Additionally, safety-relevant serological parameters (liver function and blood cell counts) were evaluated. Furthermore, laboratory parameters influenced by other anti-FcRn agents in clinical studies were considered.
Results:
Of 31 patients with TA, 19 were administered the anti-FcRn mAb subcutaneously once weekly for 12 weeks, while 12 were on placebo. Compared to placebo, there was no increased occurrence of AE and/or SE in the mAb group. mAb treatment increased total, low-density lipoprotein (LDL), and high-density lipoprotein (HDL) cholesterol. A mAb treatment-induced transient decrease in serum albumin strongly correlated with an increase in total cholesterol (r = –0.893, P = 0.012). Overall compared to placebo, there were no significant changes in blood cell counts, complement factors, or liver enzymes. Serological changes were transient and spontaneously normalized after treatment completion. Two SAEs were deemed no-drug induced (dysthyroid optic neuropathy and a post-COVID infection associated autoimmune encephalomyelitis).
Conclusions:
The anti-FcRn mAb is a safe and well-tolerated therapy for AAb-mediated AID.
Keywords
Anti-FcRn monoclonal antibody, drug safety, adverse events, side effects, thyroid autoimmunityIntroduction
The half-life of immunoglobulin (Ig) G and human serum albumin (HSA) is approximately 21 days, with IgG being the most common Ig in circulation [1–3]. The relevant half-life is due to a specific receptor-mediated recycling mechanism. The neonatal crystallizable fragment receptor (FcRn) was first described in 1964 [4]. FcRn is a major histocompatibility complex I (MHC-I)-like heterodimer consisting of a non-covalently linked α and β2m subunit. The presence and function of FcRn have been extensively characterized over the past decades [5–8]. Three essential functions of FcRn have been described: (a) transport of IgG across membrane barriers, (b) uptake and presentation of IgG-bound antigens to immune cells, and (c) protection of IgG and albumin from excessive degradation (recycling loop). FcRn’s specificity for its ligands is high; no other Igs or proteins bind to the receptor. The transport and recycling mechanisms are strictly pH-dependent [9–11]. By extending the half-life of IgG, FcRn contributes to maintaining immune defense in healthy organisms and significantly impacts the onset and course of autoimmune diseases (AID). The severity of IgG-mediated AID, such as thyroid autoimmunity (TA) or myasthenia gravis (MG), strongly correlates with the titer of pertaining autoantibodies (AAb) [12–16]. The receptor-mediated recycling loop of AAb can significantly influence the onset and progression of AAb-mediated AID. For IgG-mediated AID, e.g., TA, currently only unspecific and symptomatic treatment is available [17, 18]. In contrast to disease-targeted therapy, non-specific treatments often cause side effects (SEs). As early as the 1990s, it was shown that mice administered AAb developed AID. Knock-out animals with an FcRn deficiency did not develop AID or a milder form [19–21]. This led to considering FcRn as a potential target for treating AAb-mediated AID. In recent years, both anti-FcRn-Ab and engineered Fc targeting FcRn have been developed for the therapy of IgG-mediated AID [22–28]. However, the use of anti-FcRn-Ab in patients with TA was reported in one single study only [29, 30].
Hence, this exploratory monocentral study evaluates the safety of a novel anti-FcRn monoclonal antibody (mAb) in patients with TA who participated in a randomized, placebo-controlled trial [29, 30]. The mAb binds with high affinity to the FcRn at acid and neutral pH. Further, the Fc has been engineered, reducing effector functions, that is, antibody-dependent cell-mediated cytotoxicity and complement-dependent cytotoxicity.
Materials and methods
Study design and criteria of eligibility
The clinical trial has been approved by the local independent Ethics Committee (IEC) of Rhineland Palatinate (IEC no. 2019-14297-AMG; approved March 11, 2020) and performed according to the Declaration of Helsinki and the Guidelines for Good Clinical Practice (GCP). All patients have given their written informed consent prior to screening for eligibility. Patients with TA randomly received either the anti-FcRn mAb (treatment group) or placebo for 12 weeks. This manuscript is a presentation of data from a single center. The analyses are exploratory and do not include the prospectively defined endpoints of the main multicenter trial [29].
Patients aged 18 years and older were considered eligible for participation if they had active TA within nine months of screening, euthyroidism or mild thyroid dysfunction at baseline, and were on a stable medical regimen. Exclusion criteria included prior treatment with steroids within three weeks prior to screening or other immunosuppressive medications within the past nine months before baseline. Patients with acute or chronic viral hepatitis, relevant malignancies, or chronic renal failure were excluded. Patients with IgG levels < 6 g/L, serum albumin levels < 3.5 g/L, absolute neutrophil counts < 1,500 cells/mm3, or elevated (> 1.5-fold) liver enzymes at screening did not meet eligibility criteria. A comprehensive list of inclusion and exclusion criteria is available in the Supplementary material.
Documentation of adverse events and SEs
All recorded adverse events (AEs) were assigned codes using the Medical Dictionary for Regulatory Activities (MedDRA). MedDRA is a medical terminology developed by the International Conference on Harmonization of Technical Requirements for Registration of Pharmaceuticals for Human Use (ICH). The use of MedDRA is strongly recommended to accurately and transparently describe the safety profile and quantify potential AE and SEs during drug therapy or clinical trials. The English version 24.1 of MedDRA was utilized, and the relevant guidelines were followed. The severity of all AE was assessed and categorized as mild, moderate, or severe, as follows: mild (no interference with daily activities), moderate (interference with daily activities), and severe (prevention of daily activities). Follow-up was conducted for all AE, and the final outcome was documented. Each AE was evaluated for its relationship to drug intake and seriousness, based on the criteria outlined in the ICH guideline-E6 for GCP.
Demographic and clinical data
Forty patients have been screened for eligibility. Of the 31 randomized patients, 19 received the mAb and 12 placebo. Of the remaining nine, six patients were excluded because they did not meet the inclusion criteria, while three patients were not randomized due to the early termination of the trial. One patient in the treatment group withdrew from the study after week four. Complete demographic, clinical, and serological data are offered in Table 1. With very few exceptions (e.g., weight), no significant differences were noted between the groups at baseline.
Demographic and serological data
Study group | mAb (n = 19) | Placebo (n = 12) | P-value |
---|---|---|---|
Gender, n (%) | > 0.999 | ||
Female | 13 (68.42) | 9 (75) | |
Male | 6 (31.58) | 3 (25) | |
Age (years), mean ± SD | 48.65 ± 11.02 | 48.08 ± 12.04 | 0.670 |
Weight (kg), mean ± SD | 78.15 ± 15.22 | 70.90 ± 13.44 | 0.044 (*) |
Height (m), mean ± SD | 1.73 ± 0.06 | 1.68 ± 0.11 | 0.154 |
Smoking habits | > 0.999 | ||
Non-smoker, n (%) | 15 (78.95) | 10 (83.33) | |
Smoker, n (%) | 4 (21.05) | 2 (16.67) | |
Duration of thyroid autoimmunity (month), mean ± SD | 4.37 ± 2.34 | 5.25 ± 2.14 | 0.269 |
Serology (baseline), mean ± SD | |||
Serum albumin (32–55 g/L) | 46.74 ± 2.45 | 47.92 ± 2.50 | 0.335 |
Cholesterol (0–119 mg/dL) | 204.10 ± 52.95 | 206.90 ± 33.43 | 0.727 |
HDL (< 40 mg/dL) | 62.32 ± 16.27 | 61.58 ± 15.08 | 0.696 |
LDL (0–129 mg/dL) | 123.80 ± 46.89 | 125.70 ± 32.11 | 0.712 |
TG (0–199 mg/dL) | 90.58 ± 59.12 | 100.80 ± 44.05 | 0.174 |
ASAT (0–41 U/L) | 17.79 ± 4.54 | 17.50 ± 3.26 | 0.944 |
ALAT (0–45 U/L) | 18.68 ± 10.27 | 14.92 ± 6.63 | 0.263 |
AP (35–104 U/L) | 87.11 ± 29.52 | 78.17 ± 18.30 | 0.453 |
γGT (0–65 U/L) | 25.00 ± 26.06 | 15.17 ± 5.11 | 0.772 |
Lymphocytes (abs.) (1–4 × 10E9/L) | 1.90 ± 0.59 | 2.06 ± 0.67 | 0.787 |
Monocytes (abs.) (0.1–0.9 × 10E9/L) | 0.38 ± 0.22 | 0.35 ± 0.07 | 0.745 |
Platelets (150–350 × 10E9/L) | 260.40 ± 64.28 | 266.80 ± 58.58 | 0.735 |
WBC (4–10.7 × 10E9/L) | 6.41 ± 1.75 | 6.31 ± 1.55 | 0.897 |
CH50 (79–187 uEq/mL) | 111.90 ± 34.07 | 115.80 ± 25.88 | 0.757 |
C3c (0.9–1.8 g/L) | 1.16 ± 0.17 | 1.17 ± 0.19 | 0.895 |
* Significant difference. n: number of patients/events. γGT: gamma-glutamyltransferase; abs.: absolute number; ALAT: alanine aminotransferase; AP: alkaline phosphatase; ASAT: aspartate aminotransferase; C3c: complement factor 3c; CH50: complement factor CH50; HDL: high-density lipoprotein; LDL: low-density lipoprotein; mAb: monoclonal antibody; SD: standard deviation; TG: triglyceride; WBC: white blood cell
Serological parameters
The collection of serological parameters was carried out at predefined visits. This exploratory study focused on typical safety-related parameters (liver function and blood cell titers). Furthermore, parameters that were influenced by other anti-FcRn mAb or Fc in clinical trials were considered (serum albumin, complement system factors, and blood lipid levels). To evaluate a potential influence on these laboratory parameters by the mAb, the lab parameters were analyzed on day 1 (D1, baseline), D50, D78, and D134 (end of study).
Statistical analysis
All serological results obtained at the four visits (D1, D50, D78, and D134) were analyzed. The course has been plotted and the area under the curve (AUC) was calculated for each parameter. To document trends between active treatment and follow-up phases, the delta between each evaluation visit was calculated. Each parameter has been evaluated (a) within the study group and (b) between the treatment groups. Analysis was performed with a type I error of 0.05 (α = 0.05) and a 95% confidence interval (95% CI). Obtained data were tested for statistical significance using either the Wilcoxon test or the Mann-Whitney-U test (group internal tests and group comparison, respectively). Parameters were tested for various correlations using Spearman’s correlation test. Correlations were rated according to Cohen’s criteria as minor (0.1 < r < 0.3), moderate (0.3 < r < 0.5), or high (r > 0.5). Analyses were performed with the statistical software GraphPad Prism (version 9.3, GraphPad Software Inc.). Furthermore, the estimated effect of the time and baseline value has been investigated using a mixed linear model (IBM SPSS Statistics, version 27, IBM Inc.) during the active treatment phase. Estimated effect for the treatment duration, considering the baseline values, analysis was performed with a type I error of 0.05, and results are presented as an estimate with 95% CI. In addition, the estimated effect of the treatment arm considering the baseline values was evaluated.
Results
Safety
A total number of 61 AEs were reported by 26 patients (83.87%) in both groups. The most frequent AEs were: nervous system disorders (n = 22), gastrointestinal disorders (n = 7), infections and infestations (n = 7), as well as musculoskeletal and connective tissue disorders (n = 5) (Table 2). The majority of the occurred AE were transient and mild or moderate (62.30% and 29.51%, respectively). No injection site AE occurred during or after mAb administration. Of the observed AE, 28/61 (45.90%) were drug-related SE: 10/23 (43.48%) and 18/38 (47.37%) in the placebo and mAb groups, respectively (Table 3). Occurred SE were: nervous system disorders (lethargy, n = 12), gastrointestinal disorders (diarrhea, n = 3; nausea, n = 3), general disorders and administration site conditions (ankle edema, n = 1; erythema, n = 1; limb edema, n = 1), infections and infestations (herpes simplex infection, n = 2; shingles, n = 1), musculoskeletal and connective tissue disorders (arthralgia, n = 2), and psychiatric disorders (insomnia, n = 2). All SEs were transient and either mild (67.86%) or moderate (32.14%).
MedDRA-coded adverse events
Adverse event (MedDRA SOC & LLT) | Total number (n) | mAb (n) | Placebo (n) |
---|---|---|---|
Cardiac disorders | 1 | ||
Palpitations | 1 | ||
Ear and labyrinth disorders | 1 | ||
Ear noises | 1 | ||
Eye disorders | 3 | ||
Deterioration of visual acuity | 2 | ||
Optic neuropathy# | 1 | ||
Gastrointestinal disorders | 7 | ||
Aphthae | 1 | ||
Diarrhea | 1 | 2 | |
Nausea | 2 | 1 | |
General disorders and administration site conditions | 4 | ||
Ankle edema | 2 | ||
Erythema | 1 | ||
Limb edema | 1 | ||
Infections and infestations | 7 | ||
Herpes simplex infection | 1 | 1 | |
Hordeolum | 1 | ||
Inguinal abscess | 1 | ||
SARS-CoV-2 infection | 1 | ||
Shingles | 1 | ||
Vaginal mycosis | 1 | ||
Injury, poisoning, and procedural complications | 1 | ||
Sprain | 1 | ||
Investigations | 2 | ||
Increased intraocular pressure | 1 | 1 | |
Musculoskeletal and connective tissue disorders | 5 | ||
Arthralgia | 1 | 2 | |
Groin pain | 1 | ||
Myalgia | 1 | ||
Nervous system disorders | 22 | ||
Autoimmune encephalomyelitis# | 1 | ||
Dizziness | 1 | ||
Headache | 6 | ||
Lethargy | 8 | 4 | |
Paresis | 1 | ||
Paresthesia | 1 | ||
Psychiatric disorders | 2 | ||
Insomnia | 1 | 1 | |
Reproductive system and breast disorders | 3 | ||
Irregular menstruation | 3 | ||
Skin and subcutaneous tissue disorders | 1 | ||
Neurodermatitis | 1 | ||
Vascular disorders | 2 | ||
Hypertension | 1 | 1 | |
Total number of adverse events | 38 | 23 | |
Total number of patients with adverse events (%) | 15/19 (78.95) | 11/12 (91.67) | |
Total number of patients with serious adverse events (%) | 2/19 (10.52) | 0/12 (0.00) |
# Adverse event classified as serious adverse event. n: number of patients/events. LLT: lower limit term; mAb: monoclonal antibody; MedDRA: Medical Dictionary for Regulatory Activities; SOC: system organ class
MedDRA-coded drug-related side effects
Drug-related side effect (MedDRA SOC & LLT) | Total number (n) | mAb (n) | Placebo (n) |
---|---|---|---|
Gastrointestinal disorders | 6 | ||
Diarrhea | 1 | 2 | |
Nausea | 2 | 1 | |
General disorders and administration site conditions | 3 | ||
Ankle edema | 1 | ||
Erythema | 1 | ||
Limb edema | 1 | ||
Infections and infestations | 3 | ||
Herpes simplex infection | 1 | 1 | |
Shingles | 1 | ||
Musculoskeletal and connective tissue disorders | 2 | ||
Arthralgia | 1 | 1 | |
Nervous system disorders | 12 | ||
Lethargy | 8 | 4 | |
Psychiatric disorders | 2 | ||
Insomnia | 1 | 1 | |
Total number of drug-related side effects | 18 | 10 | |
Total number of patients with drug-related side effects (%) | 12/19 (63.16) | 8/12 (66.67) | |
Total number of patients with serious drug-related side effects (%) | 0/19 (0.00) | 0/12 (0.00) |
n: number of patients/events. LLT: lower limit term; mAb: monoclonal antibody; MedDRA: Medical Dictionary for Regulatory Activities; SOC: system organ class
Two serious AEs (SAEs) occurred in the treatment group. One patient reported deterioration of visual acuity at visit D78. Orbital MRI confirmed dysthyroid optic neuropathy (DON). At the time of diagnosis, the patient had received 12 out of 12 doses of the mAb. The SAE was deemed as “not drug-related”. According to the European guidelines for thyroid eye disease (TED), the patient was administered intravenous glucocorticoids every second day [single dose of 0.75 g methylprednisolone (MP) per infusion, cumulative dose of 7.5 g]. Intravenous steroids led to significant clinical improvement and the patient was discharged after two weeks.
The second patient developed a SARS-CoV-2 infection at D85. Subsequently, the patient reported worsening visual acuity with visual field defects and tingling paresthesia in the hands and feet. At the onset of the COVID infection, the patient had received 11 of the foreseen 12 mAb injections. Administered oral steroids were not helpful. Orbital MRI revealed significant inflammatory lesions of the central nervous system (CNS). A control cranial MRI confirmed the inflammatory lesions. Based on imaging and present lymphocytic pleocytosis in the cerebrospinal fluid, suspected virus-induced autoimmune encephalomyelitis was diagnosed. Hence, the SAE was deemed as “not drug-related”. During hospitalization, the patient received five repetitive daily doses of 1 g intravenous MP (cumulative dose: 5 g IVMP) and underwent five sessions of plasmapheresis. Unfortunately, the patient did not respond to the above treatments. Hence, rituximab (RTX) 1 g was administered intravenously twice within two weeks, which led to clinical stabilization. However, the SAE persisted beyond the patient’s last visit.
Serology
Serum albumin
On mAb, HSA decreased after the first injection, reaching a nadir at D50 (HSAD1 vs. HSAD50, P < 0.001). HSA levels spontaneously normalized to approximate baseline levels at visit D134 (HSAD1 vs. HSAD134, P > 0.999). The estimated effect of the treatment duration negatively impacted HSA concentration (estimate = –0.114 g/L, 95% CI: –0.153/–0.076, P < 0.001). While deltas between D1–D50 and D1–D78 were not different (P > 0.999), deltas between D1–D134 and D1–D50, as well as D1–D78, were distinct (P = 0.014 and P = 0.023, respectively). No significant differences were observed with placebo. mAb AUC [5,182 (g/L)*d, 95% CI: 4,829/5,536] was lower compared to placebo [6,228 (g/L)*d, 95% CI: 6,032/6,424] (P < 0.001). Considering the baseline values, the estimated effect for the treatment group, demonstrated a negative impact vs. placebo (estimate = –5.560 g/L, 95% CI: –8.566/–2.555, P < 0.001). Notably, the groups differed at visits D50 and D78 (P < 0.001 and P < 0.001, respectively), but no significant variance was observed at visit D134 (P = 0.549). Taken together, compared to placebo, mAb transiently reduced HSA with subsequent normalization at therapy completion (Figure 1).
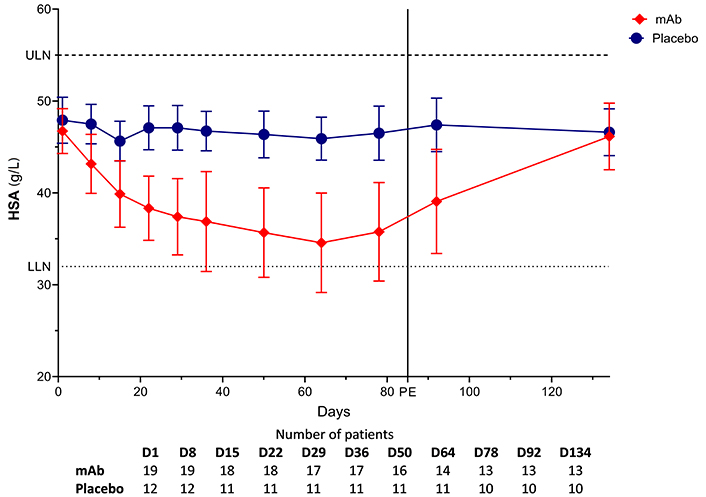
Course of serum albumin. D: day; HSA: human serum albumin; LLN: lower limit of normal (32 g/L; dotted line); mAb: monoclonal antibody; PE: primary endpoint; ULN: upper limit of normal (55 g/L; dashed line)
Lipids
The analyzed blood lipid levels encompass total cholesterol, high-density lipoprotein (HDL), low-density lipoprotein (LDL), and triglycerides (TGs). The anti-FcRn mAb increased serum total cholesterol concentration with a peak at D50 (cholesterolD1 vs. cholesterolD50, P = 0.002). Subsequently, these values spontaneously returned to baseline levels (cholesterolD1 vs. cholesterolD134, P > 0.999). Treatment duration positively affected total cholesterol (estimate = 0.830 mg/dL, 95% CI: 0.521/1.139, P < 0.001). Delta values between D1–D50 and D1–D78 were not different (P = 0.568). In contrast, values between D1–D134 and D1–D50 displayed significant variations (P = 0.003). On placebo, no differences in total cholesterol levels were noted between visits or delta values. The estimate for the treatment duration yielded a negative effect (estimate = –0.037 mg/dL, 95% CI: –0.277/0.203, P = 0.758). mAb AUC [32,364 (mg/dL)*d, 95% CI: 25,933/38,796] and placebo [28,358 (mg/dL)*d, 95% CI: 24,435/32,280] did not differ (P = 0.334). The estimated effect of mAb, considering baseline values, demonstrated a positive effect compared to placebo (estimate = 39.33 mg/dL, 95% CI: 20.437/58.225, P < 0.001). Significant differences were observed at D50 (P = 0.004), but not at D78 and D134 (P = 0.181 and P = 0.111).
On mAb, compared to baseline, serum LDL increased at visits D50 (P = 0.002) and D78 (P = 0.014). LDL levels spontaneously normalized to baseline values after mAb withdrawal (LDLD1 vs. LDLD134, P > 0.999). The estimate for treatment duration showed a positive effect (estimate = 0.555 mg/dL, 95% CI: 0.319/0.790, P < 0.001). Although differences were not observed between D1–D50 and D1–D78 delta values (P > 0.999), differences were evident between D78–D134 and both D1–D50 (P = 0.002) and D1–D78 (P = 0.005). On placebo, no differences were observed at all visits or in the delta values. The estimate for the treatment duration revealed a negative but non-significant effect for placebo (estimate = –0.051 mg/dL, 95% CI: –0.270/0.167, P = 0.639). The AUC for the anti-FcRn mAb [19,243 (mg/dL)*d, 95% CI: 13,623/24,864] and placebo [16,893 (mg/dL)*d, 95% CI: 13,263/20,523] did not differ (P = 0.521). Notably, the estimated effect for the treatment groups concerning baseline values showed a positive effect for mAb (estimate = 23.135 mg/dL, 95% CI: 10.952/35.318, P < 0.001). At none of the considered visits was any registered difference between the groups. However, the calculated delta values D1–D50, D1–D78, and D78–D134 differed between the two groups (P < 0.001, P = 0.017, and P = 0.007, respectively).
Throughout the study, no significant differences in HDL levels were observed. The estimate for the treatment duration revealed a positive mAb effect (estimate = 0.142 mg/dL, 95% CI: 0.045/0.240, P = 0.005) and a negative effect for placebo (estimate = –0.065 mg/dL, 95% CI: –0.015/0.019, P = 0.124). The AUC of mAb [8,711 (mg/dL)*d, 95% CI: 6,511/10,911] and placebo [7,802 (mg/dL)*d, 95% CI: 6,289/9,316] did not differ (P = 0.531). Notably, the estimated effect of the treatment groups, concerning baseline values, demonstrated a positive effect for mAb (estimate = 9.847 mg/dL, 95% CI: 5.494/14.201, P < 0.001). Calculated delta values differed at visits D1–D50 (P = 0.001) and D1–D78 (P = 0.002) between the study groups.
In addition, no significant differences in TG levels were observed within or between the treatment groups.
In summary, an increase in total, LDL, and HDL cholesterol was observed during mAb therapy in contrast to placebo. The observed effect was transient and the values returned to baseline levels after therapy completion. The course of the blood lipids is offered in Figure 2.
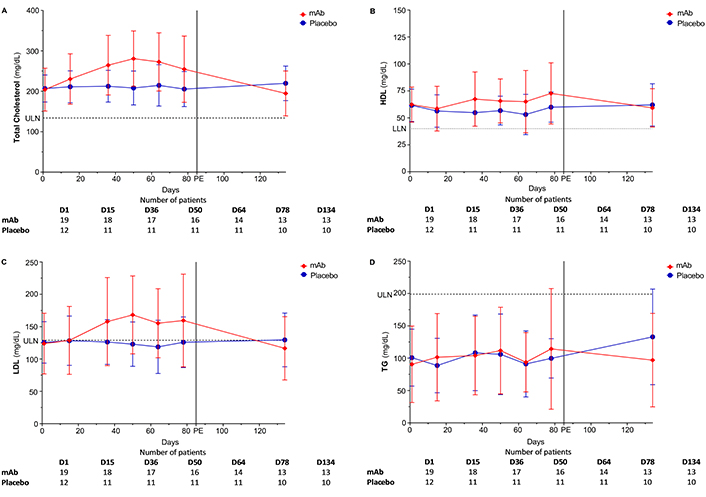
Course of blood lipids. (A) Total cholesterol: LLN = 0 mg/dL, ULN = 119 mg/dL; (B) HDL: LLN < 40 mg/dL; (C) LDL: LLN = 0 mg/dL, ULN = 129 mg/dL; (D) TG: LLN = 0 mg/dL, ULN = 199 mg/dL. D: day; HDL: high-density lipoprotein; LDL: low-density lipoprotein; LLN: lower limit of normal (dotted line); mAb: monoclonal antibody; PE: primary endpoint; TG: triglyceride; ULN: upper limit of normal (dashed line)
Liver function
The analyzed liver values encompass: alanine aminotransferase (ALAT), alkaline phosphatase (AP), aspartate aminotransferase (ASAT), and gamma-glutamyltransferase (γGT).
A mAb-induced AP increase was noted already after the first injection. Compared to D1, AP was higher at D50 (P = 0.018, peak level). Serum AP spontaneously and gradually normalized after treatment completion (APD1 vs. APD134, P > 0.999). The estimation of treatment duration showed a positive effect (estimate = 0.167 U/L, 95% CI: 0.040/0.295, P = 0.011). The delta values D1–D50 and D1–D78 were not different (P > 0.999). In contrast, the delta values between D78–D134 and D1–D50, as well as D1–D78, exhibited relevant differences (P = 0.030 and P = 0.018). On placebo, no effect was observed between individual visits, among the delta values, or for treatment duration (estimate = –0.012 U/L, 95% CI: 0.105/0.080, P = 0.788). The AUC for mAb [12,427 (U/L)*d, 95% CI: 10,087/14,761] and placebo [10,207 (U/L)*d, 95% CI: 8,606/11,808] did not differ (P = 0.153). Compared to the placebo, the mAb estimated effect showed a positive effect, relative to baseline values (estimate = 13.790 U/L, 95% CI: 6.548/21.033, P < 0.001). The two groups differed at visit D50 (P = 0.015) but not at visits D78 (P = 0.237) or D134 (P = 0.552). Delta values further differed at D1–D50 (P < 0.001) and D1–D78 (P = 0.014).
mAb increased γGT, peaking at D64 (γGTD1 vs. γGTD64, P > 0.999), with values spontaneously normalizing (γGTD1 vs. γGTD134, P > 0.999). The estimated effect for treatment duration, dependent on the baseline value, resulted in a positive effect (estimate = 0.008 U/L, 95% CI: –0.075/0.091, P = 0.850). The delta values between D1–D50 and D1–D78 did not differ (P > 0.999). However, this was the case between D1–D134 and D1–D50, as well as D1–D78 (P = 0.014 and P = 0.201, respectively). On placebo, no differences were noted. The estimated effect for treatment duration showed a negative effect (estimate = –0.022 U/L, 95% CI: –0.046/0.002, P = 0.075). The AUC for the anti-FcRn mAb [2,958 (U/L)*d, 95% CI: 1,577/4,338] was lower compared to placebo [1,835 (U/L)*d, 95% CI: 1,495/2,175], but the two areas did not differ (P = 0.176). The estimated effect of the treatment arm, considering baseline values, indicated a positive effect for the mAb (estimate = 5.456 U/L, 95% CI: –3.616/14.527, P = 0.228). While the groups did not show significant differences at each visit, they differed in the delta values of D1–D50 (P = 0.017) and D1–D78 (P = 0.046).
Finally, no differences were observed in the course of the respective groups or between the groups for ASAT and ALAT.
Taken together, no significant differences were observed between or within the groups for the parameters ASAT, ALAT, and γGT. However, there was a mAb-induced transient and significant increase in AP compared to placebo. The course of each parameter is illustrated in Figure 3.
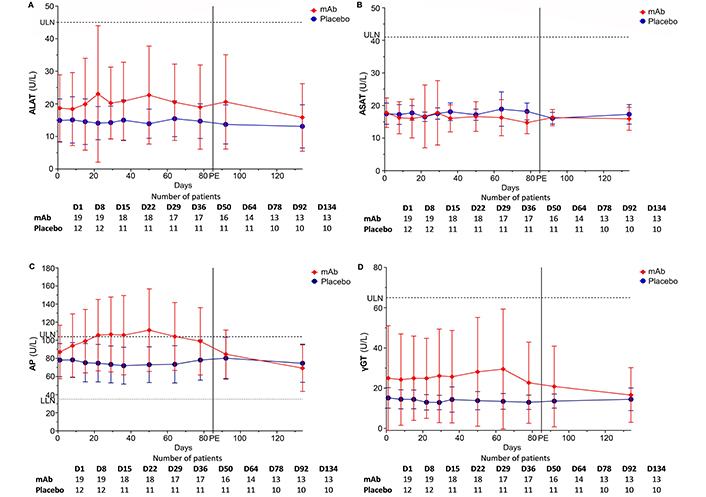
Course of liver enzymes. (A) ALAT: LLN = 0 U/L, ULN = 45 U/L; (B) ASAT: LLN = 0 U/L, ULN = 41 U/L; (C) AP: LLN = 35 U/L, ULN = 104 U/L; (D) γGT: LLN = 0 U/L, ULN = 65 U/L. γGT: gamma-glutamyltransferase; ALAT: alanine aminotransferase; AP: alkaline phosphatase; ASAT: aspartate aminotransferase; D: day; LLN: lower limit of normal (dotted line); mAb: monoclonal antibody; PE: primary endpoint; ULN: upper limit of normal (dashed line)
Blood cell titers & complement factors
No differences were observed between both groups or within each group for blood cell titers (lymphocytes, monocytes, platelets, and white blood cells) or complement factors (CH50 and C3c).
Correlations
The mAb-induced decrease of HSA negatively correlated with increasing levels of total (r = –0.893, P = 0.012), LDL (r = –0.750), and HDL-cholesterol (r = –0.656). Positive correlations between total cholesterol and both HDL (r = 0.571, P = 0.200) and LDL (r = 0.857, P = 0.024) were observed. Serum LDL and HDL cholesterol also correlated (r = 0.786, P = 0.048). In the placebo group, no significant effects were noted: HSA vs. total cholesterol (r = –0.143, P = 0.783), HSA vs. both HDL (r = 0.619, P = 0.138) and LDL (r = 0.143, P = 0.783). Mild negative (r = –0.214, P = 0.662) and positive correlations (r = 0.286, P = 0.556) between total cholesterol and HDL/LDL were observed (Figures 4 and 5). In the placebo group, a moderate positive correlation between LDL and HDL values was registered (r = 0.429, P = 0.354). In both groups, positive correlations between AP and γGT were noted in the mAb (r = 0.755, P = 0.001), and placebo (r = 0.192) groups (Table 4).
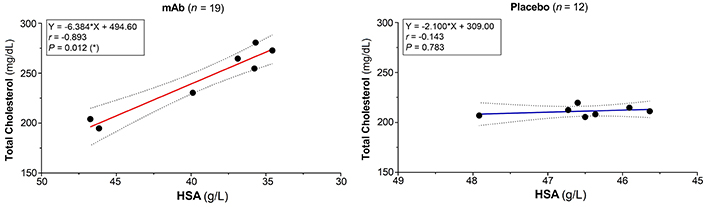
Negative correlation albumin-cholesterol. 95% Confidence interval is shown as dotted lines. * Significant difference. n: number of patients/events; r: Spearman’s correlation coefficient (minor: 0.1 < r < 0.3; moderate: 0.3 < r < 0.5; high: r > 0.5). HSA: human serum albumin; mAb: monoclonal antibody
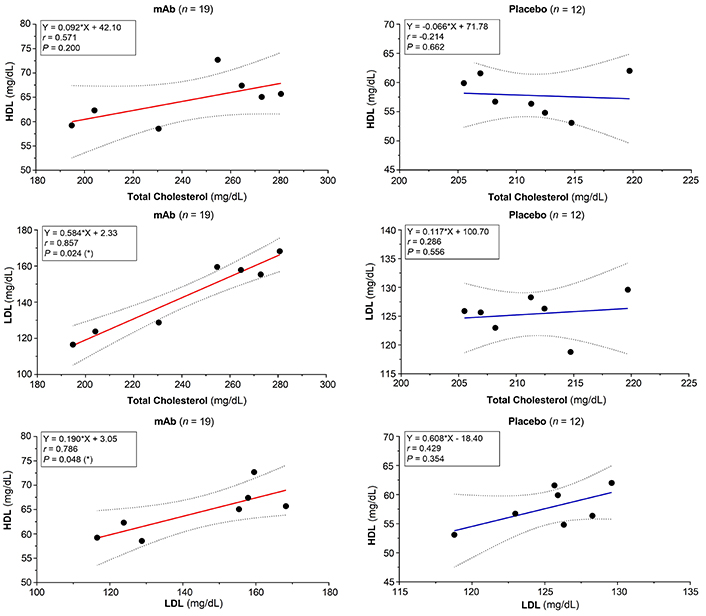
Correlation of blood lipids. 95% Confidence interval is shown as dotted lines. * Significant difference. n: number of patients/events; r: Spearman’s correlation coefficient (minor: 0.1 < r < 0.3; moderate: 0.3 < r < 0.5; high: r > 0.5). HDL: high-density lipoprotein; LDL: low-density lipoprotein; mAb: monoclonal antibody
Spearman’s correlation matrix
Parameter 1 | Parameter 2 | Spearman correlation (r) | Interpretation (Cohn’s criteria) | P-value |
---|---|---|---|---|
Monoclonal antibody | ||||
HSA | Cholesterol total | –0.893 | Negative correlationHigh correlation | 0.012 (*) |
HSA | HDL | –0.656 | Negative correlationHigh correlation | 0.110 |
HSA | LDL | –0.750 | Negative correlationHigh correlation | 0.066 |
Cholesterol total | HDL | 0.571 | Positive correlationHigh correlation | 0.200 |
Cholesterol total | LDL | 0.857 | Positive correlationHigh correlation | 0.024 (*) |
LDL | HDL | 0.786 | Positive correlationHigh correlation | 0.048 (*) |
γGT | AP | 0.755 | Positive correlationHigh correlation | 0.001 (**) |
Placebo | ||||
HSA | Cholesterol total | –0.143 | Negative correlationWeak correlation | 0.783 |
HSA | HDL | 0.619 | Positive correlationHigh correlation | 0.138 |
HSA | LDL | 0.143 | Positive correlationWeak correlation | 0.783 |
Cholesterol total | HDL | –0.214 | Negative correlationWeak correlation | 0.662 |
Cholesterol total | LDL | 0.286 | Positive correlationWeak correlation | 0.556 |
LDL | HDL | 0.429 | Positive correlationModerate correlation | 0.354 |
γGT | AP | 0.192 | Positive correlationWeak correlation | 0.570 |
* Significant difference; ** highly significant difference. n: number of patients/events. γGT: gamma-glutamyltransferase; AP: alkaline phosphatase; HDL: high-density lipoprotein; HSA: human serum albumin; LDL: low-density lipoprotein
Discussion
AID characterized by the presence of autoantibodies usually correlates clinically with the serum titers of these AAb [31–33]. In animal experiments, AAb-induced AID can be triggered by their administration. The majority of AAbs belong to the IgG subgroup. Reducing IgG levels in these experiments resulted in a milder course of the diseases [19–21]. Under physiological conditions, the FcRn serves as an essential enzyme for maintaining IgG titers and a functional immune defense. FcRn selectively extends the half-life of IgG and HSA through a recycling mechanism. Experiments with FcRn-deficient mice have shown that mice lacking FcRn do not develop AAb-dependent AID or, in cases of very high serum titers, exhibit a comparatively milder form [19–21].
TA is characterized by thyroid AAbs, e.g., anti-thyroperoxidase and anti-thyroglobulin antibodies. These AAb, having an IgG phenotype, may lead to thyroid dysfunction and remodeling of the thyroid tissue [34–37]. The fact that selective reduction of IgG AAb can improve AID qualifies the FcRn as a potential target for the treatment of TA. The here-tested fully human mAb exhibits high selectivity for the FcRn and, through Fc modifications, has low cell-mediated cytotoxicity and complement-dependent cytotoxicity. Our exploratory study aimed to assess the safety and tolerability of this anti-FcRn mAb in patients with TA within a randomized, placebo-controlled phase 2 trial [29]. Besides our tested mAb, other substances targeting FcRn are currently undergoing clinical trials [22–28]. To account for a possible group effect of these substances targeting FcRn, we also considered parameters influenced by these substances in other clinical studies (HSA, blood lipid levels, and complement factors).
The mAb-induced decrease in HSA concentration correlating with an elevation of total and LDL cholesterol is clinically known. Patients with analbuminemia (idiopathic hypoalbuminemia) due to a genetic defect are unable to produce HSA or only minimal amounts [38–41]. Clinically, these patients exhibit elevated total and LDL cholesterol with minimally elevated HDL and TG. Symptomatically, patients experience peripheral edema and often fatigue. Similarly, in the nephrotic syndrome, significant loss of blood proteins like HSA occurs due to impaired blood filtration [42–46], resulting in increased total and LDL cholesterol levels, while HDL remains unchanged.
The significant loss of albumin likely leads to reduced breakdown and increased synthesis of lipids [41, 46, 47]. In vitro studies suggest a pseudoesterase activity for HSA, leading to the breakdown of acetyl coenzyme A (acetyl-CoA) [48]. With reduced HSA, less acetyl-CoA is metabolized, and more cholesterol is produced by the 3-hydroxy-3-methyl-glutaryl-CoA reductase (HMG-CoA reductase) [47, 48]. Due to albumin’s partly hydrophobic structure, it can bind to lipophilic substances such as fatty acids and transport these peripherally. The loss of HSA leads to the redistribution of fatty acids onto lipoproteins, resulting in increased serum LDL and very low-density lipoprotein (VLDL) concentrations [41, 47, 49]. These mechanisms were confirmed in a mouse model with albumin-deficient mice [50]. However, a few studies in animal experiments also observed an increase in ASAT. The fact that FcRn can bind and recycle HSA, similar to IgG, suggests that the mAb-caused inhibition leads to an HSA deficit. Ligands at FcRn have different binding epitopes that do not hinder each other [51–53]. One possible cause for HSA loss by our tested mAb could be that after completing the recycling cycle, FcRn remains longer in a blocked state on the cell surface. The mAb-induced transient increase of γGT and AP suggests a potential mAb effect on the biliary system. However, based on the available data, it is challenging to determine whether the bile disorder was caused by an increase in cholesterol levels.
The two SAEs in the mAb group were deemed as “not drug-related”. First, DON is regarded as an ophthalmic deterioration of TA. Second, there is no evidence (preclinical experiments or clinical studies) that FcRn inhibition may cause CNS inflammation. The expression of FcRn was demonstrated in the blood-brain barrier, but not directly in the CNS [54, 55]. Animal models demonstrated that FcRn plays an important role in the efflux or IgGs from immune-privileged organs like the CNS [56]. However, there are no convincing facts that the post-viral encephalitis was drug-induced. The consulting neurologists and the neurological tests clearly highlighted the most probable viral etiology.
The tested mAb was accompanied by atypical AE, e.g., headaches, nausea, and diarrhea. In other clinical studies using anti-FcRn compounds, AEs were mostly classified as mild to moderate [22–28, 57]. In contrast to previous reports, the difference in safety assessment focuses on blood lipid levels. None of the other studies described whether there was a change in cholesterol or LDL/HDL.
Several limitations of the present exploratory work ought to be discussed: (a) the non-prospective nature of the study, (b) the three treatment arms were combined in comparison with placebo, (c) the relatively small total number of treated and evaluated patients, due to the early termination of the trial. Further, it should also be emphasized that only patients at one center of the multicenter trial are evaluated in our work; with a higher number of included patients, statistical power usually increases while potential bias will decrease, (d) statistical analyses were neither prospectively defined nor controlled for multiplicity, and (e) different doses of the mAb could have varied with dose-dependent effects on serological parameters, e.g., HSA and blood lipid levels. Due to the low number of patients per group, a dose-dependent effect could not be evaluated.
Overall, the administered mAb can be considered safe and well-tolerated. Supportive, temporary therapy with cholesterol-lowering agents (such as statins) during the anti-FcRn mAb treatment might be beneficial. Further studies assessing the safety of the here-tested mAb for treating antibody-mediated AID are warranted.
Abbreviations
95% CI: | 95% confidence interval |
AAb: | autoantibody |
AEs: | adverse events |
AID: | autoimmune diseases |
ALAT: | alanine aminotransferase |
AP: | alkaline phosphatase |
ASAT: | aspartate aminotransferase |
AUC: | area under the curve |
CNS: | central nervous system |
CoA: | coenzyme A |
D1: | day 1 |
DON: | dysthyroid optic neuropathy |
FcRn: | neonatal crystallizable fragment receptor |
GCP: | Good Clinical Practice |
HDL: | high-density lipoprotein |
HSA: | human serum albumin |
ICH: | International Conference on Harmonization of Technical Requirements for Registration of Pharmaceuticals for Human Use |
IEC: | independent Ethics Committee |
IgG: | immunoglobulin G |
LDL: | low-density lipoprotein |
mAb: | monoclonal antibody |
MedDRA: | Medical Dictionary for Regulatory Activities |
MP: | methylprednisolone |
SAE: | serious adverse event |
SEs: | side effects |
TA: | thyroid autoimmunity |
TGs: | triglycerides |
γGT: | gamma-glutamyltransferase |
Supplementary materials
The supplementary material for this article is available at: https://www.explorationpub.com/uploads/Article/file/1003145_sup_1.pdf.
Declarations
Author contributions
JW: Conceptualization, Data curation, Visualization, Writing—original draft, Writing—review & editing. IK: Validation, Writing—review & editing. GJK: Conceptualization, Investigation, Validation, Writing—original draft, Writing—review & editing, Supervision.
Conflicts of interest
GJK consults for Immunovant, Inc., New York City, NY, USA. The other authors declare that they have no conflicts of interest.
Ethical approval
The ASCEND GO 2 study was approved by the leading Ethics Committee (Rhineland Palatinate, 2019-14297-AMG).
Consent to participate
Informed consent to participate in the study was obtained from all participants.
Consent to publication
Not applicable.
Availability of data and materials
The raw data supporting the conclusions of this manuscript will be made available by the authors, without undue reservation, to any qualified researcher.
Funding
The JGU Medical Center receives research-associated funding from Immunovant, Inc., New York City, NY, USA [lmmunovant IMV-02524]. The funders had no role in the design of the submitted study, data collection and analysis, decision to publish, or preparation of the manuscript.
Copyright
© The Author(s) 2024.