Abstract
The control of the immune system, neuroendocrine system, and energy metabolism is essential for the physiological process of male reproduction. The hypothalamic-pituitary-testicular (HPT) axis regulates the generation of gonadal steroid hormones in the testes, which in turn controls spermatogenesis. For the growth and maturation of germ cells, the immune cells and cytokines in the testes offer a safe microenvironment. The cellular reactions and metabolic activities in the testes produce energy and biosynthetic precursors that control the growth of germ cells, as well as testicular immunology and inflammation. Both inflammatory and anti-inflammatory responses depend on immune cell metabolism, which is thought to influence testicular spermatogenesis. The significance of immunometabolism in male reproduction will be underlined in this review.
Keywords
Neuroendocrine, HPT, immunometabolism, spermatogenesis, cytokinesIntroduction
The goals of this brief review are to familiarize the reader with the anatomy and histology of the testes, to survey those immune modulatory factors in semen that may prevent sensitization to sperm in women, and to promote embryo implantation. To review the role of Sertoli cells in the formation of the blood-testes barrier (BTB), in the context of preventing autoimmunity to sperm, and to discuss the role of sperm in the formation of the BTB. The concept of the BTB, also referred to as the Sertoli cell seminiferous epithelium barrier, was initially coined by Chiquoine in 1964 during research on the impact of cadmium toxicity on testicular necrosis [1]. The BTB is a specialized feature within the testes, essential for shielding developing sperm cells from the immune system. The primary constituents of this barrier are Sertoli cells, specialized supporting cells found within the seminiferous tubules of the testes. The BTB is crucial for upholding an immune-privileged setting within the seminiferous tubules, safeguarding against the body’s immune system initiating an autoimmune reaction against sperm cells, which possess distinctive antigens absent elsewhere in the body [2]. The complex regulation of the endocrine and immunological systems coordinates the multi-step process of male reproduction, which begins with the synthesis of germ cells in the testes and the transportation of sperm to the sperm-egg binding site in the fallopian tube [3]. Because meiosis and subsequent cellular differentiation events necessary for spermatogenesis take place after the systemic tolerance is developed, spermatogenesis poses a special challenge to the immune system [4]. Early-stage germ cells (spermatogonia) located outside of BTB can now become autoantigenic foreign entities to the immune system because of all subsequent post-meiotic (round and elongating spermatids) germ cells [5]. Three distinct steps can be distinguished in the sperm-formation process: Spermatogenesis is the process of creating sperm cells that have undergone both the first and second meiotic divisions while maintaining their spherical shape. The initial stage entails the division of diploid germ cells known as spermatogonia, which subsequently undergo mitotic divisions to generate primary spermatocytes, maintaining their diploid status. The subsequent phase involves meiotic divisions that halve the chromosome number, resulting in the formation of haploid cells known as spermatids. Spermiogenesis marks the concluding stage of sperm development, wherein spermatids undergo substantial structural and functional modifications to transform into mature, motile sperm. These resulting mature sperm, referred to as spermatozoa, are discharged into the lumen of the seminiferous tubules and subsequently traverse through the epididymis for additional maturation and storage [6]. Spermiation is the release of spermatids from their association with Sertoli cells into the lumen of the seminiferous tubule [7].
Spermatozoa do not have the capacity to fertilize eggs when they leave the testes and reach the epididymis; instead, they develop this capacity as they move through the epididymis. Although the exact mechanism of this process is yet unknown, it is linked to the development of propulsive motility and changes in the sperm plasma membrane and glycocalyx [8]. If one looks at the histology of testes in cross-section (Figures 1 and 2) they will reveal that the spermatogonia, which are the progenitor (PG) cells from which spermatocytes undergoing meiosis are created, rest on a layer of myoid cells that surround the seminiferous tubule [6]. Sertoli cells rise like “trees of a forest” from the seminiferous tubule’s base to its lumen. They participate in the segregation of spermatids and spermatocytes from the systemic immune system, the endocrine regulation of pituitary gonadotropins, and the process of spermiogenesis [7]. Inhibin, which is largely produced by Sertoli cells, feeds back negatively to the anterior pituitary, regulating the release of follicle-stimulating hormone (FSH) [9]. Sertoli cells facilitate the ascent of primary spermatocytes from the spermatogonia towards the tubular lumen. The Sertoli cell’s only syndrome, which is characterized by the absence of spermatogonia and is linked to azoospermia, is the condition in which their architecture within the seminiferous tubules is most clearly visible. Round spermatids go through cytoplasm loss, the development of a sperm tail that enables cell motility, and the formation of a mid-piece with mitochondria that fuels sperm motility during spermiogenesis is also produced during this process called acrosome. For fertilization to take place, it is crucial that this structure, which is situated over the rostral portion of the spermatozoon head, functions [10]. The acrosome is a specialized granule that includes the multifunctional adhesion molecule vitronectin, the trypsin-like enzyme acrosin, and additional as of yet unknown moieties that play roles in gamete interactions that result in fertilization [11]. Before gaining the capability to fertilize, sperm undergo a process called capacitation, which denotes the physiological alterations occurring in the female reproductive tract, notably in the fallopian tubes, enabling them to acquire the capacity to fertilize an egg. In this process, the sperm glycocalyx is altered, and the plasma membrane cholesterol is lost [12]. The “acrosome reaction” happens when sperm attach to a glycoprotein in the zona pellucida, the membrane that covers the unfertilized egg. Sperm receptors become cross-linked after zona binding, which raises intracellular calcium and encourages the acrosome reaction. The sperm plasma membrane merges with the outer acrosomal membrane during this phase, resulting in fenestrations through which the contents of the acrosome are expelled [13]. The egg goes through activation at this point, completing the second meiotic division, releasing the second polar body, and releasing cortical granules into the perivitelline space, which change the zona pellucida and stop secondary sperm from binding to it and penetrating it [14].
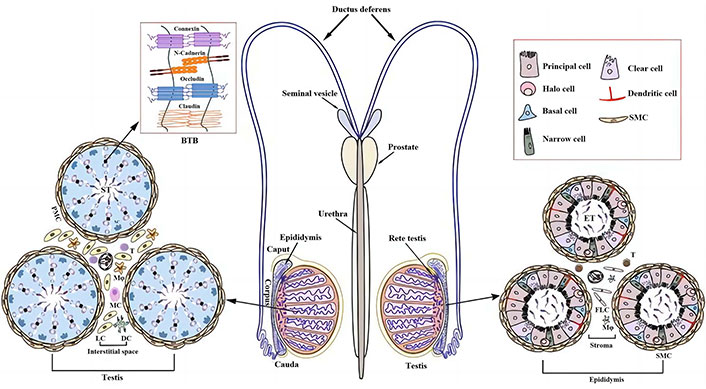
Male reproductive system [15]. BTB: blood-testes barrier; DC: dendritic cell; ST: seminiferous tubules; PMC: peritubular myoid cells; Mφ: macrophage; MC: mast cells; LC: Leydig cells; SMC: smooth muscle cells; ET: epididymal tubules; FLC: fluorochloridone
Note. Adapted with permission from “Infectious and Immunological Aspects of Male Infertility” by Jiang Q, Han D. In: Simoni M, Huhtaniemi IT, editors. Endocrinology of the Testis and Male Reproduction. Cham: Springer International Publishing; 2017. pp. 1051–69 (https://doi.org/10.1007/978-3-319-44441-3_35). © 2017 Springer International Publishing AG.
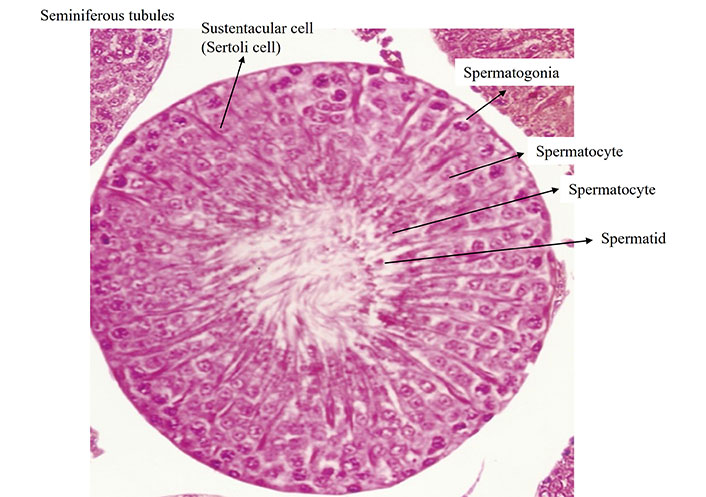
Histological structure of testis (HE; ×400; fluorescent microscope with bright field digital microphotography Leica-1000, serial No./model-Leica DFC-450C DM-1000)
Note. Adapted with permission from “Evaluation of contraceptive efficacy of methanol extract of Citrullus colocynthis fruits in male Wistar rats” by Sharma C, Mali PC, Chaturvedi M. Med Drug Res. 2015;3:6–14 (https://www.researchgate.net/publication/301545968_Evaluation_of_contraceptive_efficacy_of_methanol_extract_of_Citrullus_colocynthis_fruits_in_male_Wistar_rats).
Reproductive studies revealed that immunometabolic problems may lead to infertility. The reproductive system ensures the perpetuation of the species, while the immune system safeguards internal well-being, thereby supporting sustained health and survival [16]. Male reproductive tracts and gametes pose a distinctive challenge to the immune system. Unlike hematopoietic tissues, which are present from early development, sperm only differentiate after sexual maturation, long after the immune system has matured and systemic immunity has been established [17]. Immunometabolism is a recently developing field of research that focuses on the interaction between the immune and metabolic systems [18]. A growing body of evidence from cellular and animal studies examining how metabolism affected immune cell activity has been published, opening up novel therapeutic possibilities for a variety of conditions associated with immune system dysregulation, including autoimmune illnesses and cancer [19].
Regulation of hypothalamic-pituitary-testicular axis on male reproduction
The hypothalamic-pituitary-testicular (HPT) axis is homeostatically regulated by both positive and negative feedback regulatory mechanisms. The gonadotropin-releasing hormone (GnRH), which controls the production and release of the gonadotropins luteinizing hormone (LH) and FSH by the pituitary gland, is the primary regulator of the HPT axis (Figure 3). GnRH is secreted from the hypothalamus in a periodic pulsatile manner. In turn, gonadotropins operate on the testes to promote the production of sex gonadal steroid hormones and regulate the morphological and physiological changes unique to the testis [20]. In contrast, the pituitary and hypothalamus get constant negative feedback from the testes’ testosterone release to keep levels of GnRH, LH, and FSH stable. In order to maintain good male reproductive function, these gonadal hormones specifically promote physiological homeostasis through feedback regulatory mechanisms in conjunction with pituitary gonadotropins [21].
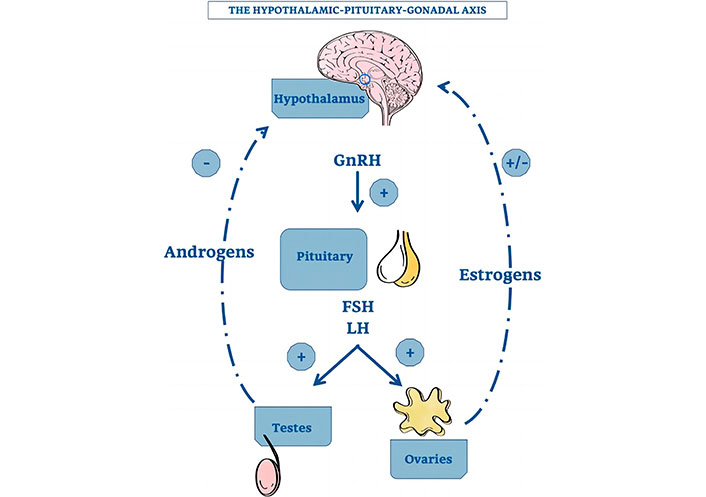
The hypothalamic-pituitary-gonadal axis [22]. GnRH: gonadotropin-releasing hormone; FSH: follicle-stimulating hormone; LH: luteinizing hormone
Note. Reprinted from “Thymus-Pineal Gland Axis: Revisiting Its Role in Human Life and Ageing” by Rezzani R, Franco C, Hardeland R, Rodella LF. Int J Mol Sci. 2020;21:8806 (https://doi.org/10.3390/ijms21228806). CC BY.
Another important aspect that directly affects male reproduction is the regulation of the metabolic activity in the testes. Specific metabolic requirements are necessary for the development of germ cells, which preferentially use lactate as a substrate for the generation of ATP [23]. Through glycolysis and fatty acid oxidation (FAO), Sertoli cells meet their own and the germ cells’ energy needs [24]. It has been established that the HPT axis hormones FSH and sex steroid hormones control Sertoli cell metabolism [25]. The metabolism of Sertoli cells is also regulated by androgens and oestrogens. In human Sertoli cells, 17β-estradiol and 5α-dihydroxytestosterone are said to control lactate synthesis and glucose uptake [26]. Recently, it has been reported that sex hormones govern lipid metabolism in adult rat Sertoli cells by transcriptionally modulating the expression of the genes [27], thanks to a genome-wide analysis of androgen and estrogen receptor (ER) binding sites. Leydig cells are triggered by LH and metabolize cholesterol to testosterone and other steroid hormones, which are necessary for spermatogenesis and other processes for male reproduction despite energy metabolism for germ cell development [28].
Influence of GnRH and pituitary gonadotropins on reproductive organs
As the neuroendocrine, immunological, and central neurological systems are connected through a complex network [29] of signal molecules, such as cytokines, hormones, and neurotransmitters [30], the immune system does not function in isolation. The hypothalamic-pituitary-gonadal (HPG) axis and associated hormonal system may affect immunological function, according to evidence [31]. GnRH controls immunological and neuroendocrine processes in an autocrine or paracrine manner. Human peripheral lymphocytes have been found to contain the immunoreactive and bioactive GnRH receptor (GnRH-R), suggesting that GnRH may function as an autocrine or paracrine agent to modulate immunological functions [32]. Blockade of central and peripheral GnRH-R during maturation of the brain-thymus-lymphoid axis and HPG axis remarkably inhibits immune system development [33]. Thymocyte counts and immune cell development were suppressed when GnRH antagonist was given to rodent and primate fetuses, indicating GnRH is essential for immune system modulation and development [34]. Interleukin-2 (IL-2) and interferon-g (IFN-g) are two cytokines that GnRH stimulates in animals, boosting their production and the activation of immune cells [35]. When combined, these parts of evidence suggest that GnRH may play a direct role in the cell- and humoral-mediated immune response despite the lack of studies demonstrating the immunomodulatory effects of FSH and LH, experimental and clinical data suggested that these two gonadotropins stimulate the immune cell proliferation and alter the production of cytokines (like IL-10 and IFN-g) [36]. Male patients with idiopathic hypogonadotropic hypogonadism (IHH) had gonadotropin treatment, and the immune cell populations changed. This suggests that gonadotropin can affect both cell-mediated and humoral immunity [37]. Additionally, it has been demonstrated that the HPT axis’s FSH and sex steroid hormones (androgens and oestrogens) control Sertoli cell metabolism.
Effect of sex steroid hormones on the immune system
Due to their lipophilic nature, sex steroid hormones have the ability to integrate into immune cell membranes and alter their membrane’s physical and functional characteristics [30]. The two primary gonadal steroid hormones generated by the testes are androgens and oestrogens. The widely recognized ERs and androgen receptors (ARs), found in primary lymphoid organs and various immune cells, are pivotal in regulating a range of physiological processes such as reproductive functions, bone health, and metabolic activities through activation by their respective ligands [35].
Effects of androgen
Incidences of autoimmune illness are still substantially lower in men than in women, yet males make 20 times more testosterone than women do. As a result, androgens are thought to have an impact on both the innate immune system’s development and operation as well as the adaptive immune system [38]. Loss of testicular immune privilege was observed in mice with AR deficiency, which is characterized by an increase in serum levels of inflammatory cytokines, such as IL-1b and tumor necrosis factor α (TNF-α), and the number of macrophages in circulation demonstrating the function of androgen in testicular immune privilege in Sertoli cells [39]. Testosterone levels are lower in rats with experimental autoimmune orchitis (EAO), a type of male immunological infertility.
Testosterone is the major androgen in the testes. Less than 3% of testosterone circulates in a free, bioavailable form. Sex hormone-binding globulin (SHBG) plays a crucial role in regulating the amount of unbound testosterone accessible to target tissues due to its high affinity for testosterone. Upon entering target cells, testosterone is converted into the most biologically active androgen, dihydrotestosterone (DHT), by the enzyme 5α-reductase, predominantly in the male reproductive organs. Additionally, testosterone can undergo metabolism by aromatase, leading to the production of estradiol. This conversion primarily occurs in adipose tissue, the hypothalamus, and hematopoietic cells [40]. In comparison to the EAO rat without testosterone replacement, testosterone therapy had a protective effect on disease development and the inflammatory response. It prevented the growth of macrophages and decreased the number of CD4+ T cells while increasing the number of regulatory T cells (Tregs) in the testes [41]. While having no effect on testicular macrophages, testosterone reduces the lipopolysaccharide (LPS)-induced inflammatory response on TNF-α mRNA expression in Sertoli cells and peritubular cells, which support spermatogenesis, spermatozoa transport, and testicular immune control [42]. Androgens affect the quantity and secretion of testicular immune cells, which in turn affects the function and reactions of innate immunity cells in mammals as well as the immunosuppressive effect on male reproduction. It’s evident that androgens influence the innate immune response through various mechanisms such as cell proliferation, cytokine secretion, and toll-like receptor expression. Interindividual genetic variations within innate immune genetic pathways, along with non-infectious environmental factors, could further influence the effects of androgens on the innate immune response [43]. The function and mechanism of androgens in controlling the testicular immunological state have yet to be fully understood.
Effects of estrogen
As important physiological regulators in men, oestrogens (estrogens) have a positive impact on the immune system [38]. The mammalian immune system expresses two intracellular ER subtypes (ERα and ERβ) that control the formation of immune cells and the innate and adaptive immune systems [44]. Many different immune cell types (Figure 4), including T cells, B cells, macrophages, dendritic cells (DCs), and natural killer (NK) cells, express both ERα and ERβ [45]. In males, estrogen controls immunity and upholds immunometabolic function. The over expression of human aromatase genes in transgenic male mice (AROM+ animals) stimulated testicular macrophage activation; however, a rat model of EAO showed increased testicular macrophages, demonstrating the stimulating influence of oestrogens on the immunoregulation of male reproductive function [46]. Androgens and oestrogens have varied effects on various immune cell subsets, and sex steroids as a whole regulate the immune system. Generally speaking, androgens seem to have a stronger immunosuppressive effect than estrogen does on immune cells and immunological function. As a result, although oestrogens have immunoenhancing effects in the immune-privileged environment of the testes, androgens have immunosuppressive effects, which calls for more research.
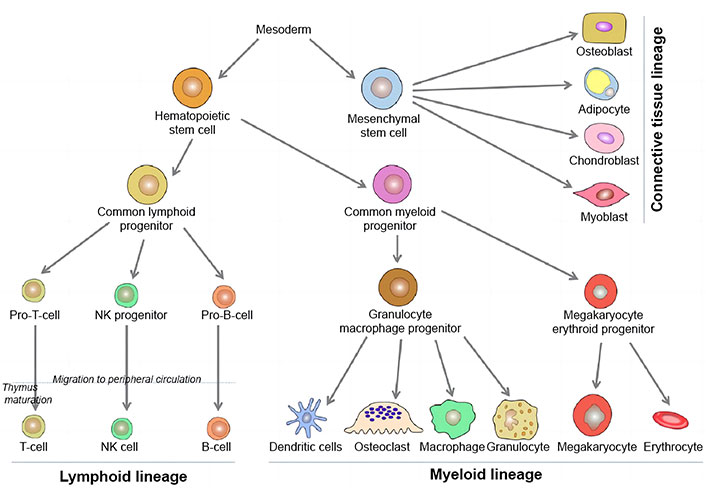
Diagrammatic presentations of cells and organs of immune system [47]. NK: natural killer
Note. Reprinted with permission from “Paget’s disease of bone: an osteoimmunological disorder?” by Numan MS, Amiable N, Brown JP, Michou L. Drug Des Devel Ther. 2015;9:4695–707 (https://doi.org/10.2147/DDDT.S88845). CC BY-NC.
The metabolic influence of immune cell activity on male reproduction
Through the generation of cytokines and other immunoregulatory chemicals like androgens by interacting cell types like macrophages, DCs, T cells, NK cells, mast cells, and Leydig cells, the testicular interstitial space has strong immunoregulatory abilities [48]. While maintaining defense against microbial infections, these immune cells largely exhibit a high tolerance to germ cell autoantigens. Currently, the signals from the microenvironment, such as androgens, have been suggested that prostaglandins and corticosterone affect the phenotypic and functionality of testicular immune cells [49]. The area of immunometabolism has advanced significantly over the past ten years because metabolic flux can determine a cell’s fate, such as its ability to perform regulatory and effector functions on immune cells. However, metabolic reprogramming in testicular immune cells has not been demonstrated. This might be because there aren’t many human nonpathological tissue samples available [50]. In COVID-19 scenarios, an inflammatory cytokine storm arises, which diminishes viral infection within cells and results in heightened leukocyte infiltration into interstitial tissue. This cascade ultimately leads to orchitis and male infertility [51]. It is conceivable that immunometabolism patterns in tumors and the gravid uterus, both of which are immune-privileged places, have partially been identified. These patterns could serve as a guide for the newest fields of research on immunometabolism in male reproduction. Thus, we seek to identify potential immunometabolic pathways implicated in testicular function in this research.
Role of macrophages
Macrophages are immune cells that are active in most prevalent in the interstitial area of the testes. For instance, around 80% of the testicular leukocytes in rat testicles were macrophages. According to a recent study on human testes, resident macrophages in the testes make up about 62% of the testicular myeloid cells and express the M2 macrophage marker (CD163) at levels six times greater than those of blood monocytes [52]. In a stable state, these macrophages can sustain themselves for extended periods of time without needing blood monocyte replenishment. Leydig cells and interstitial macrophages have intimate interactions, which may help testosterone production. For instance, when Leydig cells were cultivated in the medium that had been conditioned by testicular macrophages, the production of testosterone increased dramatically, although it had less of an impact on the conditioned medium from peritoneal macrophages [53]. By interacting with the AR in Sertoli cells, testosterone controlled the expression of downstream genes such as reproductive homeobox 5 (Rhox5) and then controlled spermatogenesis processes such as maintaining spermatogonial numbers and BTB, having spermatocytes complete meiosis, adhering elongated spermatids to Sertoli cells, and releasing mature spermatozoa [54].
A testosterone derivative called DHT affects the amounts of enzymes and transporters involved in glycolysis, such as lactate dehydrogenase and phosphofructokinase 1, which has an impact on glucose metabolism in rat Sertoli cells [55]. The retinoic acid biosynthetic enzymes aldehyde dehydrogenase 1A2 (ALDH1A2) and retinol dehydrogenase 10 (RDH10), as well as colony stimulating factor 1 (CSF1), may be expressed by testicular macrophages to encourage the proliferation and differentiation of spermatogonial cells. Spermatogonia development would be disturbed in testes lacking in macrophages after ALDH1A2 and RDH10 levels decreased [56]. It is unknown if the immunosuppressive status in the testicular interstitial space was partially caused by the nutritional composition. According to the aforementioned data, testicular macrophages may increase oxidative metabolism while decreasing anaerobic metabolism in order to retain M2 macrophage phenotype. However, further research is required to fully demonstrate the role of macrophages in spermatogenesis and their pattern of metabolism in the testes.
Role of DCs
Under physiological circumstances, testicular DCs, a tiny immune cell population within the testes, are phenotypically immature and functionally tolerogenic DCs. Under physiological circumstances, they are connected to the adaptive immune system of the testes and play a role in the inactivation of effector T cells and the formation of Tregs [57]. Additionally, it’s possible that immature DCs play a role in the identification of typical sperm antigens and the establishment of immunological tolerance to safeguard sperm cells under physiological circumstances. For the growth of immature DCs, FAO and oxidative phosphorylation (OXPHOS) are crucial catabolic processes. Notably, substances that support the OXPHOS pathway, such as vitamin D3, may support tolerogenic DC (acquired tolerant status) phenotype and function, including the generation of IL-10 [58]. IDO (indoleamine 2,3-dioxygenase), an enzyme that catalyzes’ the metabolism of tryptophan and ultimately produces kynurenine, is typically kept at a basal level in DCs. Kynurenine has been discovered to trigger the development of Foxp3+ (Forkhead box protein 3 gene) Tregs and the overexpression of programmed cell death-1 (PD-1) receptor on activated CD8+ T cells, both of which were linked to immunosuppression. Kynurenine serves as an endogenous ligand for aryl hydrocarbon receptors on T cells [59]. In tumors and the uterus during pregnancy, both of which are immunologically privileged sites like the testes, IDO is said to induce DCs to start immune tolerance and stimulate the production of Tregs [60]. IDO was only weakly expressed by testicular DCs, and EAO rats had decreased IDO activity. These variations could be explained by the Sertoli cells’ indirect inhibition of LPS-induced DC maturation through a paracrine action. The expression of inducible nitric oxide synthase (iNOS), cyclooxygenase 2 (COX2), and IDO is reduced in bone marrow-derived DCs when they are cocultured with Sertoli cells from mouse testes that released galectin-1, whereas the levels of IL-10 and TGF-b1 are dramatically elevated in LPS-stimulated DCs, providing evidence in favor of this theory [61]. By upregulating co-stimulatory proteins like CD40, CD80, and CD86, inflammatory cytokines, and major histocompatibility complex (MHC) class-II, maturing DCs under pathological conditions overcome immune tolerance, which leads to autoimmunity and male infertility [62]. The production of iNOS, a reactive nitrogen species that can limit mitochondrial respiration and reduce OXPHOS, is likewise increased in mature DCs stimulated by LPS [63].
Role of lymphocytes
Lymphocytes made up 10–20% of the total leukocyte population and were sparsely distributed across the interstitial space in healthy human and rat testes, while B lymphocytes were not present [64]. However, the number of lymphocytes, including Tregs and effector T helper cells (Th1 and Th17), rises dramatically in the testicular interstitial space after inflammation brought on by infection or autoimmunity. T cells in rat testes are primarily of the CD8+ subset, in contrast to peripheral blood where CD4+ T cells were the predominate lymphocyte subset. A sizable population of NK cells was also discernible [65]. Foxp3+ under physiological settings, rat, mouse, and human testes include the potent immunosuppressive cells known as Tregs, which may explain the immunosuppressive properties of testes [66]. The CD8+ and CD4+ T-cell subsets’ ability to activate may be inhibited by testosterone. Ethane dimethane sulfonate dramatically raised CD8+ and CD4+ T cell numbers by killing Leydig cells. Lower than baseline amounts of CD8+ and CD4+ T cells would result from the addition of testosterone working with recovering Leydig cells [67]. In order to oxidase exogenous FAs, which is slowed by increased glycolysis, mitochondrial FAO and OXPHOS are crucial for Treg production and suppressive activities. Adenosine monophosphate-activated protein kinase (AMPK) and transcription factor Foxp3 are two regulators of Tregs suppressive function that work by preventing glycolysis and increasing FAO by blocking motor activity [68]. To encourage proliferation and counteract their suppressive effect, Tregs up regulate anaerobic glycolysis and the expression of glucose transporter 1 (GLUT1). A greater number of effector T cell subsets can overcome the inhibitory impact of Tregs by generating proinflammatory cytokines including TNF-α and IL-6 in inflammatory testes, despite higher CD4+ and CD8+ Tregs and TGF-b expression [69]. Thus, excessive T cell activation in autoimmune conditions may not be controlled by Tregs alone.
When T cells are activated, the metabolism of the cell undergoes a dynamic metamorphosis that helps the cell defend itself from infections and coordinate the actions of other immune cells. T cells engage in anaerobic glycolysis, a process typically seen in highly proliferative cells where glucose is digested into lactate rather than oxidized in mitochondria, to meet the bioenergetic requirements for activation. Recent research has described the dynamic profile of CD4+ and CD8+ T cells following activation and shown that glucose plays a critical role in sustaining effector responses and immune cell proliferation [70]. Numerous inhibitory molecules, including IDO and PD-1, change the metabolism of T cells by lowering the expression of GLUT1, which consequently prevents the uptake of glucose. IDO and PD-1 inhibition is also seen in NK cells, however, it is unclear if this affects the ability of these cells to exert effector function by changing their metabolism [71]. Sertoli cells may also play a role in the immunosuppression of the testicular immune privilege.
Role of mast cells
Sertoli cells may also play a role in the immunosuppression of the testicular immune privilege. Extracellular ATP may operate as a dangerous chemical to peritubular cells upon immune cell activation by activating their purinoceptors (P2RX4 and P2RX7). The expression of pro-inflammatory chemicals like IL-6 and chemokine (C-C motif) ligand 7 (CCL7) is then increased in peritubular cells as a result of ATP-mediated processes [72]. Evidence suggests that preventing the synthesis of glycolytic ATP may prevent IL-33-induced bone marrow-derived mast cell activation and the generation of the proinflammatory factors IL-6 and TNF. Therefore, mast cells’ metabolic byproducts may contribute to testicular inflammation [73]. Immune cells’ phenotypic and immunoregulatory functions are established by both their surroundings and metabolic reprogramming. In contrast, inflammatory phenotype cells employ glycolysis as a primary source of energy more than mitochondrial OXPHOS for quick pathogen elimination. Glucose and FA metabolism primarily promote cell differentiation into immunologically tolerant cell morphologies. Inflammation, autoimmune diabetes, metabolic syndrome, and even male infertility may be brought on by the interruption of metabolic reprogramming. Therefore, investigating the roles of immune cell metabolism in the testes is crucial for learning more about the cellular and molecular mechanisms behind male infertility. And this might make it easier to create brand-new anti-inflammatory medicines that target immunometabolism.
Disorders in male reproduction caused by immune dysfunction and metabolism
Impact of metabolic disorders in immune cells and functions on male reproduction
Immune cell’s metabolic reprogramming is primarily responsible for controlling both their phenotypic and adaptability. Given the abundance of immune cells in the testes, pro-inflammatory stimuli that activate and polarize macrophages cause metabolic reprogramming, which further shifts the balanced mitochondrial metabolism in favor of reactive oxygen species (ROS) generation [74]. In vitro Sertoli cell function may be regulated by the IL-1 system, and immature rats that are 21 days old have been shown to have reduced testicular steroidogenesis when exposed to IL-1b [75]. Due to the lipid peroxidation of the cytomembrane, DNA damage, protein oxidation, and enzyme inactivation caused by elevated ROS levels, male infertility is the end outcome [76]. Not only are macrophages among the immune cells that secrete certain types of cytokines in response to stimulations, but these cytokines may also have an opposite effect on immune cells’ metabolic processes and functions. Therefore, immune cells may modify cellular metabolism upon activation, resulting in the release of cytokines that may impair the immune cells’ normal activity in the testes [77].
Cytokine changes in inflammation in the male reproduction
The male reproductive system has a variety of immune cells that release cytokines in response to infections, external antigens, and chronic inflammation. The earliest sign of innate host defense against inflammation of the reproductive tract comes from the release of cytokines. One of the common etiological causes of human subfertility and infertility is orchitis [78].
In autoimmune orchitis, the immune system’s recruitment was followed by its activation and an increase in the production of pro-inflammatory cytokines including IL-1 and TNF-α. These diverse proinflammatory cytokines alter BTB permeability, which allows them to penetrate the seminiferous epithelium and trigger the death of germ cells. There is mounting evidence that semen contains higher concentrations of cytokines including IL-1b, IL-6, and IL-8, which are frequently linked to inflammation and infection of the male reproductive system [79]. It has been discovered that inflammation in the male reproductive system raises the levels of ROS, which causes cell damage. Concurrently, both necrosis and apoptosis ROS may also contribute to sperm and spermatogenic cell damage, and also harms Sertoli cells and Leydig cells in the testes, which adds to the failure of spermatogenesis [80].
Systematic metabolic and immune disorders in male reproduction
Hyperinsulinemia, hyperlipidemia, hyperleptinemia, and chronic inflammation are features of metabolic syndrome and obesity. By dysregulating the HPT axis and immunological activities in male reproduction, these conditions may also directly impact testicular functions [81]. In obese males, lower testosterone levels are linked to lower LH and worsened semen quality (including lower sperm count and motility as well as morphologically normal sperm) [82]. An obesogenic environment triggers Th1-lymphocyte and M1-macrophage chronic inflammatory responses that eventually lead to systemic inflammation in the male reproductive system and a decline in immune regulatory cells and cytokines [83]. Furthermore, obesity has been linked to elevated ROS in semen, which has a negative effect on the quality and functionality of semen. Obesity and metabolic syndrome have similar effects on male reproductive system and diabetes mellitus (DM) also alters testicular metabolism, which eventually has an impact on spermatogenesis [84].
Harnessing immunometabolism for alleviating inflammation in male infertility
Mechanism of male reproduction immune dysfunction may contribute to inflammation such as epididymitis (inflammation of the tube at the back of the testicle that stores and carries sperm) and orchitis (inflammation of one or both testicles), which can lead to malignancy, benign hyperplasia (prostate gland enlargement that can cause urination difficulty), or male infertility. Infection in the male reproductive system is most frequently treated with antibiotic therapies; however, according to recommendations from the European Association of Urology, the therapy had no beneficial impact on inflammatory changes and was unable to reverse functional deficits and anatomical dysfunction [85]. The relationship between immune and metabolism is reciprocal and includes both metabolic factors that regulate immune cell functions and the integrated role of inflammation in the pathogenesis of metabolic disorders, such as obesity and metabolic syndrome brought on by unhealthy lifestyle choices or chronic diseases [86]. These data reveal the possibility that immunometabolism-targeting treatments could represent a cutting-edge potential method for reducing inflammation and autoimmune.
Antioxidant therapy for infertility treatment
A redox imbalance caused by excessive ROS generation results in the synthesis of a lot of inflammatory cytokines [87]. Male infertility is linked to oxidative stress brought on by excessive ROS generation and chronic inflammatory conditions that manifest in the body, such as obesity and metabolic syndrome. For men with subfertility, blocking ROS production is regarded as a first line of treatment [80]. ROS play a critical role in various aspects of male reproductive functions, including supporting spermatogenesis, maintaining sperm viability, facilitating maturation, hyper activation, and capacitation, enhancing sperm motility, and triggering the acrosome reaction [88]. It is necessary to use the best management techniques, which include reducing the increased inflammatory state and changing one’s lifestyle with the proper intervention [83]. Adopting a healthy lifestyle, which includes sufficient eating, useful exercise, and successful weight control, stands out as the most important strategy for managing metabolic diseases and ensuring excellent health for male fertility. Metabolites and neutraceuticals like vitamin C, vitamin E, beta-carotene, magnesium, selenium, and zinc that are administered concurrently enhance the immune system’s regulatory mechanisms [89], causing anti-inflammatory activities that might be due to the antioxidant nature. Common antioxidants increase basic semen parameters and lower ROS and sperm DNA fragment levels, all of which have a positive impact on male fertility [90]. The heterogeneous nature of the study designs, including the type of antioxidant, dosages, treatment period, sample size, and included criteria of participants with either poor semen quality or high levels of oxidative stress, may contribute to the conflicting findings of various studies about using antioxidants to improve semen quality. However, there is a lot of variation in the claimed antioxidant effect on semen parameters, and the Cochrane review found it difficult to draw any conclusions regarding how well sperm quality is improved by antioxidants [91]. The effects of antioxidant therapy on male infertility are still debatable. The impact of antioxidant supplementation on male fertility requires further research, particularly with bigger sample sizes and well-designed randomized controlled trials.
Targeting metabolism for immune cell therapy
According to reports, the hypoglycaemic drug metformin, which is typically used as the first-line therapy for the management of type II DM (T2DM), directly lowers chronic inflammation and ROS while also enhancing insulin sensitivity, hyperglycemia, and dyslipidemia [92]. Additionally, metformin has been shown to ameliorate poor B cell function associated with T2DM in vivo, reduce B cell-intrinsic inflammation in vitro, and block antigen-induced T cell proliferation in an AMPK independent way [93]. Metformin modulates fertility status and sperm quality, particularly in T2DM, by 1) restoring the structure and weight of the testes, epididymis, and seminal vesicles, 2) inhibiting germ cell apoptosis by enhancing the nutritional function of Sertoli cells to produce, and 3) increasing sperm count, motility, and normal morphogenesis. However, the effects of metformin on male reproductive function and fertility are yet unclear. Metformin may have a negative impact on the male reproductive system, either in non-diabetic or non-T2DM circumstances [94]. Since mammalian target of rapamycin (mTOR) signaling pathway is a master regulator of cell growth and metabolism and several human diseases such as cancer, diabetes, obesity, neurological diseases, and genetics caused by disorders due to the deregulation of the mTOR pathway. mTOR is blocked by rapamycin, which also promotes tolerance, the production of memory T cells, and tissue-resident macrophages [95]. Studies have also shown that the stimulation of the mTOR signaling pathways in rat models of chronic nonbacterial prostatitis increases inflammatory immunological responses by inhibiting NF-кB and IL-1b, while rapamycin treatment reverses these effects [96].
Hormone therapy for testicular inflammation
A testicular immunosuppressive microenvironment is maintained by high testosterone levels. This is primarily related to the inhibited NF-кB signaling pathway and reduced production of proinflammatory cytokines, which result in maintained M2-like macrophage phenotype and lower systemic inflammation [50]. Some studies have attempted to regulate testosterone in an EAO animal because of the immunosuppressive effects of testosterone. Additionally, they discovered that testosterone therapy dramatically slows the evolution of inflammation. This is accomplished by preventing the influx of inflammatory immune cells and encouraging the growth of capable CD4+CD25+Foxp3+ Tregs in the testes [41, 96]. Aromatase inhibitors raise testosterone levels in obese men and enhance spermatogenesis and sperm quality, although there are still few large-scale, evidence-based researches on the treatment of male fertility [97]. Although spermatogenesis requires an acceptable concentration of testosterone, testosterone therapy that is too aggressive typically worsens male reproductive indices through a negative feedback process, and should not be utilized in the management of fertility [98]. Based on Tregs’ immunosuppressive characteristics, medications that stabilize Tregs [such as glucocorticoids and nonsteroidal anti-inflammatory drugs (NSAIDs)] or medications that encourage Tregs to differentiate (like TGF-b and all-trans retinoids) are anticipated to maximize the efficacy of Tregs-based therapies for autoimmune disease suppression [69].
Interplay among immune, endocrine, and metabolic systems in testicular function
Endocrine, immunological, and metabolic functions in the testes are all coordinately regulated during the sophisticatedly complex process of spermatogenesis. Even though BTB divides these cells, the amplified cell connections assist the intricate yet meticulous coregulation. As previously mentioned, testosterone produced by Leydig cells and regulated by LH produced in the hypothalamus plays a crucial role in the maintenance of an immunosuppressive microenvironment for normal spermatogenesis within the testes by promoting the growth of CD4+CD25+Foxp3+ Tregs and inhibiting the activation of CD8+ and CD4+ T cells. T lymphocyte infiltration would thus reduce the number of Leydig cells during inflammation brought on by LPS stimulation and viral infection (such as coronavirus disease 2019), whereas the number of Leydig cells would increase in ab, gd, and CD8 [99]. After Leydig cells were killed or their activity was impeded, the number of macrophages also drastically decreased [100]. FSH promoted lactate generation by Sertoli cells in a hypoxia inducible factor (HIF) dependent manner, acting as the main endocrine regulator of Sertoli cells. Sertoli cells would convert 75% of their pyruvate output into lactate or pyruvate to satisfy germ cells because growing germ cells cannot synthesize lactate [101]. It is debatable whether lactate produced by Sertoli cells would cause immunosuppressive macrophage in the testes. LH, FSH, androgens, and prolactin were the primary regulators of testicular FAO metabolism, especially COX2’s role in the generation of PGs. In turn, PGs indirectly controlled sperm maturation by acting as an autocrine regulator of Leydig cell steroidogenesis and Sertoli cell function [102]. Higher anaerobic metabolism is required during the activation of these immune cells to provide the quick energy needed to create pro-inflammatory cytokines like IL-6, NO, and TNF-α. The immunologically privileged characterization of the testis in this situation is undeniably influenced by metabolic reprogramming, which will be further explored in forthcoming investigations. This is supported by the finding that the levels of testosterone and PGs, which are both produced by testicular somatic cells through lipid metabolism, were higher in testes than in peripheral blood [103]. Excessive fat deposition in the scrotal area caused by obesity and metabolic syndrome would lead to proinflammatory conditions once adipocytes rupture and ultimately compromise spermatogenesis [104]. According to a recent study, a high-fat diet not only caused significantly more body weight gain than that of their age-matched littermates but also impaired spermatogenesis by changing the glucose and lipid metabolism in Sertoli cells. This resulted in the Sertoli cells becoming dysfunctional, which in turn reduced the quality of the sperm [105]. To maintain the immunological milieu of the testis and the ideal nutritional content for the spermatogenic process, the interactions between the endocrine immune system and metabolism are crucial.
Conclusions
The immune competent milieu necessary for optimal spermatogenesis and subsequent processes, like sperm maturation, is successfully provided by immune cells. The immune cells grow, activate, differentiate, and take on distinctive phenotypes in response to the combined actions of hormones controlled by the HPT axis and the immunological microenvironment of the reproductive system. The immunological microenvironment is then supported and modeled by the metabolic reprogramming of immune cells. The role of metabolic changes in immune cell activities is becoming increasingly recognized. However, it is still unclear how immunometabolism affects male fertility and whether the HPT axis is involved in altering the metabolic flux of immune cells.
Abbreviations
ARs: | androgen receptors |
BTB: | blood-testes barrier |
DC: | dendritic cell |
EAO: | experimental autoimmune orchitis |
ER: | estrogen receptor |
FAO: | fatty acid oxidation |
Foxp3: | Forkhead box protein 3 gene |
FSH: | follicle-stimulating hormone |
GnRH: | gonadotropin-releasing hormone |
HPT: | hypothalamic-pituitary-testicular |
IDO: | indoleamine 2,3-dioxygenase |
IL-2: | interleukin-2 |
LH: | luteinizing hormone |
LPS: | lipopolysaccharide |
mTOR: | mammalian target of rapamycin |
NK: | natural killer |
OXPHOS: | oxidative phosphorylation |
PD-1: | programmed cell death-1 |
PG: | progenitor |
ROS: | reactive oxygen species |
T2DM: | type II diabetes mellitus |
TNF-α: | tumor necrosis factor α |
Tregs: | regulatory T cells |
Declarations
Acknowledgments
Authors are thankful to the Head Department of Zoology, Centre for advanced studies, University of Rajasthan, Jaipur for providing laboratory facilities and CSIR-UGC-JRF for providing financial support as Junior Research Fellowship.
Author contributions
PY and PCM equally contributed to: Conceptualization, Writing—original draft, Writing—review & editing.
Conflicts of interest
The authors declare that they have no conflicts of interest.
Ethical approval
Figure 2 testis stained with HE is approved by IAEC of Department of Zoology, University of Rajasthan, Jaipur.
Consent to participate
Not applicable.
Consent to publication
Not applicable.
Availability of data and materials
Not applicable.
Funding
Not applicable.
Copyright
© The Author(s) 2024.