Abstract
The reproductive axis of male mammals implies a coordinated interaction of its three components: a population of gonadotropin-releasing hormone (GnRH)—producing neurons of the hypothalamus, gonadotropic cells of the adenohypophysis, and most distally located testes that produce male sex hormone (testosterone) and germ cells (spermatozoa). It is generally believed that for the accurate and constant realization of reproductive function, the testes must be isolated from the internal environment of the whole organism with the help of a hematotesticular barrier. The internal immune system of the testes is primarily represented by testicular macrophages. They are located primarily in the peritubular space and provide the physical organization of peritubular myoid cells into niche-like clusters that can form an environment conducive to the differentiation of spermatogonia A1 in sperm cells. The development of this complex system occurs in mammals over a long period and is determined by the timely synchronization of all its parts. Maternal immune activation (MIA) is associated with the powerful production of proinflammatory cytokines in the mother’s body and further in the placenta and fetal region. Many of these cytokines have a pleiotropic effect and act as morphogenetic factors. Thus, cytokines can influence the development of testes even in the prenatal period. The review is focused on the effect of MIA on testicular development during prenatal state and on the holistic nature of the development of the reproductive axis of mammals on the example of laboratory rodents. Laboratory rodents (especially mice) are often used to develop models of the development of certain human systems and are the generally accepted standard for the introduction of these models into medical practice.
Keywords
Mammals, male reproductive axes, maternal immune stressIntroduction
The Austrian ethologist K. Lorenz formulated the theory of critical periods of development at the end of the last century on the example of studies of the behavior of young domestic birds (geese). These works were awarded the Nobel Prize in 1973. A little later, these studies were continued in the works of DJP Barker [1], who suggested the developmental origins of chronic adult disease on the example of the development of pathologies of the cardiovascular system in humans. Critical periods are described for the development of many organ systems during the prenatal development of mammals.
Maternal immune stimulation leads to a powerful production of proinflammatory cytokines first in the mother’s blood, then in the placenta and amniotic fluid [2], which in turn leads to increased expression of proinflammatory cytokines in fetal biological fluids. These cytokines also have a pleiotropic effect and play the role of morphogenetic factors of development. This was accurately demonstrated for leukemia inhibitory factor (LIF) [3]. These cytokines can determine the abnormal path of development of many organ systems, appearing in fetal tissues in their critical periods of development.
In this article, the main attention will be focused on the mechanisms of formation of the mammalian reproductive axis and will direct the research of mammalian reproductive system immunologists to correct possible disorders in the prenatal and early postnatal period of development. This phase of the formation of the future reproductive system of a developing prenatal or immediately after the birth of a boy is very important and has great potential for correction.
Adult testis structure, function, and development
The main function of the testes is the production of germ cells which is necessary for fertilization, as well as the production of testosterone which regulates potency and libido, as well as sperm production. These functions are provided by two different types of cells (Leydig cells and Sertoli cells).
Neurohumoral regulation of spermatogenesis is well studied to date. Neurosecretory nuclei of the hypothalamus produce key factors regulating spermatogenesis—releasing hormones. They activate the basophilic cells of the adenohypophysis, which pulsatively secrete gonadotropins folliculostimulating (FSH) and luteinizing hormones (LH) into the blood. The target cells mediating its stimulating effects on the course of spermatogenesis are Sertoli cells. Sertoli cell is a target cell for FSH. Under the influence of follicle-stimulating hormone, they synthesize a number of biologically active compounds: inhibin, activin, transferrin, cytokines, androgen binding protein, and others (Figure 1).
The Leydig cells are located in the spaces between the seminiferous tubules known as the interstitial compartment. The Leydig cells are hormone-producing mammalian cells located between the connective tissue membrane and the layer of the nutrient epithelium of the testes. Testosterone and other androgen compounds are produced in them, and a small amount of female sex hormones estrogens, and progestins are also produced in them. In addition to sex hormones, they produce β-endorphin [5], small amounts of oxytocin (stimulating contractions of peritubular myoid cells of convoluted seminiferous tubules) [6], as well as interleukin (IL)-1, acting as a growth factor on type B spermatogonia [7].
Testosterone is mainly synthesized by Leydig cells by the sequential conversion of low-density lipoprotein cholesterol into pregnenolone, dehydroepiandrosterone, and androstenedione. Testosterone is the main male sex hormone responsible for the formation of secondary sexual characteristics and sexual function. Its synthesis is stimulated and controlled by LH produced by the pituitary gland.
Sertoli cells are large supporting cells with a well-developed cytoskeleton (constructed by actin, intermediate filaments, and tubulin) and cove-shaped recesses of the cytoplasm. They lie on the basement membrane of the convoluted seminiferous tubule, are a component of the hemato-testicular barrier. In the convoluted seminiferous tubule, the number of Sertoli cells reaches up to 7% of the total number of cells. In 1865 Enrico Sertoli was the first to suggest that Sertoli cells function in the seminiferous tubule as “feeding cells”. In other words, these cells provide trophic substances to male germ cells, as well as various modulators, performing the task of mediated regulators of spermatogenesis. This is ensured by the close connection of the cytoplasm of Sertoli cells and germ cells. Sertoli cells synthesize and secrete a wide variety of factors, including steroids, proteins, cytokines, growth factors, opioids, proteases, prostaglandins, cell division modulators, and so on. Thus, Sertoli cells control the process of spermatogenesis in all its aspects, ensuring the development of germ cells from stem spermatogonia to late spermatids. The mechanisms of interactions of germ cells with Sertoli cells have not yet been sufficiently studied.
The immune system of the adult testis and its role in spermatogenesis
Mammalian testes consist of two main compartments: the seminiferous tubules and the interstitial space between them. Accordingly, their main functions are spermatogenesis and steroidogenesis. The interstitial space is a loose connective tissue with thin collagen fibrils, as well as a small number of fibroblasts and mesenchymal cells. It also contains cells of the immune system, which are responsible for innate and acquired immunity, and Leydig cells localized in the form of clusters of cells near capillaries.
Two tissue-resident macrophage populations have been identified in the testis. Peritubular macrophages lie on the surface of seminiferous tubules next to the peritubular myoid cells, and interstitial macrophages are located within the testicular interstitium in close contact with Leydig cells [8–11]. Currently, it is thought that the peritubular macrophages are derived from circulating, bone-marrow-derived monocytes [11] and that the interstitial macrophages originate from fetal, yolk-sac-derived cells but are then replaced by bone-marrow-derived macrophages after birth [9].
Macrophages can account for up to 25% of the interstitial cell population in adult mice and rats [12] but remain undervalued contributors to the development and function of the testes during the fetal period. They are known to play an important protective and functional role during reproductive life [13].
At least two major adult testicular macrophage populations have been described: CSF1R+CD206+MHCII– interstitial macrophages, which are embedded amongst Leydig cells and blood vessels in the interstitial parenchyma; and CSF1R–CD206–MHCII+ peritubular macrophages, which are exclusively localized to the surface of seminiferous tubules [10, 11]. There has been some debate regarding the hematopoietic origin of adult testicular macrophage populations, with one study proposing a circulating, bone marrow-HSC-derived origin for peritubular macrophages, and others proposing a fetal-monocyte-derived origin for these [11, 14]. Interstitial macrophages have long been implicated in regulating Leydig cell steroidogenesis [13, 15, 16] while peritubular macrophages have been proposed to promote spermatogonial differentiation [10]. However, specific functions for distinct testicular macrophage populations have not been functionally investigated.
Macrophages perform an important function—they support and regulate steroidogenesis [13]. In addition, macrophages belong to the family of antigen-presenting cells. However, a number of authors have shown that testicular macrophages have properties of cells involved in the development of inflammation and antigen elimination. It is quite obvious that under normal conditions testicular macrophages have relatively low proinflammatory and sufficiently high immunosuppressive properties compared to macrophages of other organs [17]. It has been shown that they constantly produce anti-inflammatory cytokines [18]. Such phenotypic manifestations are part of a complex mechanism of immune privilege of the testis. Immune privileges are probably an adaptation mechanism to prevent damage to the most vital organs (brain, eye, and testis) by one’s own immune system. So, the sperm cells within the testes do not cause any reaction of the immune system, but outside of they are recognized as foreign tissue.
The development of mammalian testes and the factors involved in this process
In mice, gonad development begins around day 9.0 of embryonic development (ED9.0), when Sf1+ (steroidogenic factor 1), Wt1+ (Wilms’ tumor protein 1) cells of the coelomic epithelium located on the ventral side of the mesonephros begin to actively proliferate and sink into its parenchyma, forming small thickenings by ED9.5 (sex rollers) facing into the coelomic cavity. At ED10.0, these rollers begin to accumulate in the primordial germ cell [19]. At this moment of development, the gonads are arranged identically in both sexes, and their Sf1+ somatic cell precursors are bi-potential and can differentiate into both ovarian and testicular cells. The choice of the path of further development is the determination of gender, triggered by a complex cascade of gene expression. For most mammals, including mice, the key factor determining the development of testes is the Y chromosome gene Sry (sex-determining region Y), in the absence of expression of which the genes Rspo1 (R-spondin 1) and Wnt4 (Wnt family member 4) direct the development of bi-potential gonads in the ovaries [20]. That triggers the expression of the Sox9 (Sky-box transcription factor 9) gene in progenitor cells, activating the development program in them. In response, they begin to highly express the COP marker genes Amh (anti-mullerian hormone) and Dmrt1 (doublesex and mab-3 related transcription factor 1), actively proliferate and by ED12.5 form an irregular network of sex cords with germ cells located in the center [21]. The genital cords are the precursors of the convoluted seminiferous tubules of the testis, and the mesonephric duct and the tubules of the mesonephros give rise to most of its excretory system, respectively, to the epididymis and excretory tubules. The network of the testis is laid as a connecting structure between these two structures. This position of the testis network determines the features of its formation. According to recently obtained data [22–24], in mice this structure can be laid as two separate structures. One of them begins to form at the stage of the bi-potential gonad at ED10.5 from a small group of Sf1+ progenitor cells located between the tubules of the mesonephros at the anterior end of the gonad [23]. These cells by ED12.5 already form a network of tubules extending from the anterior end of the gonad to the posterior and contacting on the one hand with the tubules of the mesonephros, and on the other with the gonadal sexual cords.
It is unknown when macrophages enter the testes: In rats, testicular macrophages were first described as occurring before embryonic day ED16.0 [25]. Fetal liver-derived macrophages rather than yolk-sac-derived macrophages or bone-marrow-derived cells are the dominant cells among both interstitial and peritubular macrophages, and that macrophages are present at both testicular compartments already at birth [26]. Macrophages play an important role during de novo morphogenesis of testis cords by controlling vascularization and tissue pruning. This unexpected role for fetal macrophages may be a developmental mechanism that is used commonly during neovascularization and morphogenesis of many fetal organs [9].
Maternal immune activation and cytokine storm observed in the biological fluids of the mother and fetus
The concept of maternal immune activation (MIA) was defined clearly at the beginning of the 21st century [27]. The first such conclusions were based on the facts of the development of schizophrenia in the offspring of mothers who had had influenza in the 1980s in Finland [28]. Later, these data served as the basis for studying the effect of prenatal immune stress of a developing fetus on the development of various systems of its regulated organs. The injection of lipopolysaccharide (LPS) is a well-characterized and widely accepted model of systemic inflammation. Many studies in adults describe the effect of LPS administration on brain function. The inhibitory effect of LPS-induced inflammation on the gonadotropin-releasing hormone (GnRH) system and pulsating luteinizing hormone secretion has been clearly demonstrated in adults [29]. The administration of LPS to newborn baby rats also leads to an inhibitory effect on the formation and function of the reproductive system in adults [30]. According to experimental and clinical data of recent decades, the effects of adverse environmental factors during critical periods of development of fetal physiological systems have a negative impact on their formation and functioning [31–33]. Regulation of the formation of neuroendocrine, immune, and reproductive systems is carried out with their close interaction already in early ontogenesis [34, 35]. Changes that have arisen in the development of one of them program the development of other interacting systems. It is in early development that epigenetic mechanisms are implemented that ensure the adaptive plasticity of physiological systems in changed conditions. The effects of infectious factors on the mother’s body, pharmacological effects, improper nutrition, and lifestyle of the mother during pregnancy can disrupt the functions of the systems for a long time. The effects of various stressful stimuli on the mother can change the physiological concentrations of various mediators of the immune and neuroendocrine systems in fetuses and disrupt the regulatory mechanisms of development [33]. A significant risk factor causing miscarriage or altering the structure and functions of the interacting systems of the fetal body is inflammation induced by infectious factors in the mother [36–39]. One of the targets for the negative effects of inflammatory products is the brain of a developing fetus [37, 38]. Disorders of brain development lead to further maladaptation, and in some cases—disability of children. After birth, their risk of developing diseases such as schizophrenia, depression, and autism increases [37, 40, 41]. Changes occurring in the brain can also lead to disorders of the development of the hypothalamic-pituitary-gonadal (HPG) axis, a decrease in reproductive ability or infertility in sexually mature offspring [40–43]. In childbearing age, treatment of gonadal functions impaired in early development is often ineffective. In this regard, for the timely prevention of stress-induced disorders in people in the pre- and early postnatal periods, it is necessary to conduct research on experimental models that allow determining critical periods of development, specific signaling molecules, and mechanisms of their action. The processes of formation of physiological systems are characterized by sensitivity to many regulatory factors. To date, data are accumulating on the possibility of preventing or canceling the consequences of intrauterine inflammation [44–46]. Activation of the mother’s immune system by LPS leads to an increase in the synthesis of pro- and anti-inflammatory cytokines. Their level increases in the placenta, amniotic fluid, and on the periphery during the first 2 hours after injection. LPS and some maternal cytokines [e.g., IL-6, tumor necrosis factor-α (TNF-α), and IL-1β] activated by maternal immune challenge can pass to the fetus through the placenta and produce inflammation in the fetal brain [47]. The fetus is highly exposed to the cytokines released into the maternal circulation after LPS administration [48]. In the brain and peripheral blood of fetuses, it’s detected also a significant increase in the content of proinflammatory cytokines—TNF-α, IL-1β, IL-6, monocyte chemotactic protein (MCP)-1, LIF. Them all have a negative effect on the developing organism, including the fetal HPG system [49]. Disorders of the pituitary gland and gonads can lead to the development of reproductive potential disorders in the postnatal offspring developed after MIA. It is known that the development, steroidogenesis, and gonad functions in males and females are modeled by cytokines. Urogenital crest cells express LIF receptors [50], and IL-6 receptors have been identified in human fetal ovarian cells [51].
Possible negative effects of maternal immune stress on the formation of mature testes during embryonic development
Based on all of the above, using the example of the development of mouse testes, it can be noted that the MIA model of laboratory rodents (mice or rats) opens up new opportunities for studying this process and forming fundamental approaches to correct the negative effects of MIA in humans. It can be noted that the immune stress of the mother on ED12.0 in mice corresponds to a long period of active formation of such parts of the mammalian reproductive axis as the hypothalamic link (GnRH-neurons) and testes. The formation of the hypothalamic GnRH system was discussed earlier [52]. In this work, the attention will be focused on the formation of the final level of the reproductive axis of male mammals—the testes.
As can be understood from the previous chapters on ED12.0 in mice (which corresponds to the end of the first trimester of pregnancy in humans), significant events occur in the testis of the developing embryo, which is necessary for the organization of seminiferous tubules. During this period of development, the main pool of Sertoli cells is formed in the testes of the mouse and the hemato-testicular barrier has not yet been formed by them. As a result of the massive release of anti-inflammatory cytokines into the biological fluids of the fetus, these processes are disrupted.
In previous studies, it was shown that maternal immune stress on ED12.0 leads to the fact that the testes of her offspring develop incorrectly [2, 41]. In rats, MIA followed by LPS injection on ED12.0 caused the disrupted testicular morphology, decreased testosterone production, and coupling success in postnatal male offspring in rats [41]. While in mice MIA leads to abundant development of symplastic spermatids in the testes of her offspring [2]. The development of symplastic spermatids in mice with a recessive mutation [53], or as a result of the administration of aflatoxin to normal mice [54], leads to complete sterility of males. The sterility is associated with abnormal loss of adhesion between spermatids and Sertoli cells, which occurs when round spermatids reach step 8 of spermiogenesis. The defective adhesion is associated with the opening of cytoplasmic bridges that normally form connecting structures between adjacent spermatid nuclei. This results in the formation of spherical symplasts of spermatids. The symplasts subsequently degenerate and are either phagocytized by Sertoli cells [53].
The possibility of correcting the level of production of proinflammatory cytokines during embryonic development
Currently, attempts are being made to neutralize bacterial infections by intravenous administration of immunoglobulin G (IVIG) [43, 55]. IVIG was first used in 1996 in the treatment of pregnant women with recurrent pregnancy loss associated with combined allo- and autoimmune disorders [56]. The mechanism of action of IVIG in bacterial infection is not yet fully understood. It is assumed that IVIG, by binding to Fc-γ receptors on macrophages [57], reduces their sensitivity to signals via Toll-like receptors (TLR), including LPS, which leads to a decrease in the synthesis of pro-inflammatory cytokines and protection from LPS-induced death in mice [43].
In addition, when using a culture of proinflammatory murine bone marrow-derived macrophage differentiated in the presence of granulocyte-macrophage colony-stimulating factor (GM-CSF), it was shown that the introduction of IgG into the culture medium simultaneously with the introduction of LPS reduces the production of such proinflammatory cytokines as TNF-α and IL-6 [43]. This coincides with the results obtained in vivo, where the authors showed a decrease in the content of these cytokines in the blood serum of mice using a model of chronic sepsis in mice [58]. It has shown also that LPS stimulates cAMP production in peritoneal macrophages, immunoglobulins can reduce cAMP production activated by LPS [33]. Apparently, the inactivation of the action of LPS in peritoneal macrophages, the first to encounter and mediate the action of LPS in a pregnant female entire body, is mediated through cAMP. However, this action depends on the balance and interaction of various receptor systems present on peritoneal macrophages. Many of the mechanisms proposed for the immune-modulating effects of IVIG demonstrate the complexity of immune effector functions in disease processes. In this work, an abundance of data was presented indicating a violation of the development of the sexual phenotype and the possibility of early prenatal correction of maternal bacterial activation immediately after its development.
Activin A, a member of the transforming growth factor (TGF)-β superfamily, is one of the cytokines induced by IVIG, which modulates inflammatory processes and, like IVIG, is able to exert pro- and anti-inflammatory effects. In embryogenesis, activin A influences the proliferation of Sertoli cells [59]. Its deficiency causes testis dysgenesis in mouse embryos, which leads to low sperm production and abnormal histology of testes in adulthood. In addition, activin A is involved in oocyte maturation, endometrial restoration, decidualization, and pregnancy preservation [60]. An increase in the level of activin A in the blood after IVIG administration may contribute to the beneficial effect of IVIG on women with recurrent reproductive failure. Activin A is considered to contribute significantly to the effects of IgG [43]. The data presented above indicate the negative effect of LPS on puberty in male mice. The impact of LPS in early pregnancy causes programming disruptions in the formation of the HPG axis of the fetus, which subsequently leads to impaired reproductive system functions. It is during the early development that epigenetic mechanisms (including DNA methylation and histone modifications) are realized and can be triggered. The processes of physiological system formation are not strictly determined genetically. They are characterized by functional ability and sensitivity to many regulatory factors, which opens up opportunities for correcting developmental disorders not have a pronounced specificity, can also modulate the processes of inflammation. The IgG effect is most pronounced when the LPS-induced synthesis of proinflammatory cytokines has not yet reached its peak, i.e. 40–60 minutes after the administration of LPS [48].
Conclusions
The main conclusion from this review is that an adult testis is formed even in the prenatal development of a male mammal. Immune tolerance, provided primarily by Sertoli cells, should be formed at the end of the first trimester of pregnancy. Their number should be formed by the end of the first trimester of pregnancy in humans. MIA-produced negative effects can be corrected by timely and safe administration of IVIG in the described concentration (Figure 2).
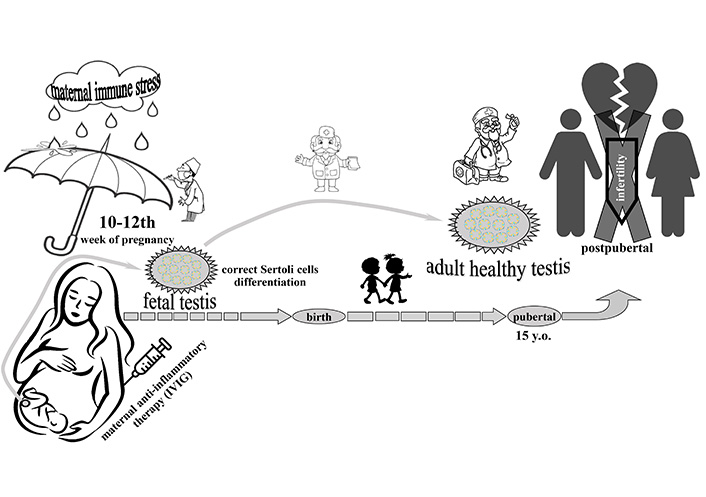
Determination of healthy mammal male reproductive system in critical period of prenatal development. Point of possible correction of maternal immune activation by intravenous administration of immunoglobulin G (IVIG) treatment is limited in some hours following the immune activations. y.o.: years old
Abbreviations
ED: | day of embryonic development |
GnRH: | gonadotropin-releasing hormone |
HPG: | hypothalamic-pituitary-gonadal |
IL: | interleukin |
IVIG: | intravenous administration of immunoglobulin G |
LIF: | leukemia inhibitory factor |
LPS: | lipopolysaccharide |
MIA: | maternal immune activation |
Sf1: | steroidogenic factor 1 |
TNF-α: | tumor necrosis factor-α |
Declarations
Author contributions
MI: Conceptualization, Investigation, Writing—original draft, Writing—review & editing, Validation, Supervision.
Conflicts of interest
The author declares no conflicts of interest.
Ethical approval
Not applicable.
Consent to participate
Not applicable.
Consent to publication
Not applicable.
Availability of data and materials
Not applicable.
Funding
The work was conducted under the IDB RAS Government basic research program in 2024 [0088-2024-0009]. The funders had no role in study design, data collection and analysis, decision to publish, or preparation of the manuscript.
Copyright
© The Author(s) 2024.