Abstract
Coronavirus disease 2019 (COVID-19) infected individuals showed either mild symptoms or were paucisymptomatic, with severe impact on human health, revealing heightened risk and direct effects on health. Among various factors contributing to complications, bacterial and fungal co-infection remains very common and is highly lethal. This narrative review aims to focus on the collective role of gut microbiota and mycobiota in COVID-19. Fungal infection has been identified as a key risk factor for the spread of COVID-19 and mortality. Gut mycobiomes diversity and abundance also vary due to the different types of SARS-CoV-2 variant infection. Their cross-talk plays a vital role in immune regulation and disease severity, with an emphasis on understanding the altered condition as a predictive marker. On the other hand, the gut microbiome is well known for shaping metabolic functions, generating immune responses, and deciphering the signal to decide the healthy state and disease condition of an individual. Immune response during COVID-19 infection was also linked with metabolites produced by the gut microflora, specifically amino acids, sugar metabolites, and neurotransmitters. The cross-talk between gut microbiota and gut mycobiota for clinical implications in terms of early detection, identification of the disease severity, and even therapeutic alternatives will open newer avenues. A deep dive understanding of the cross-talk between the microbiome and mycobiome, and their role in immune response will take scientific discovery knowledge to develop gut-targeted safe therapeutic approaches in the form of FMT (fecal microbiota transplantation) probiotics, peptides, antibacterial, and antifungal metabolites. Overall cross-talk and immune interplay are critical determinants of host immunity, providing insights into their role and key take home lessons for better management of crisis in the future.
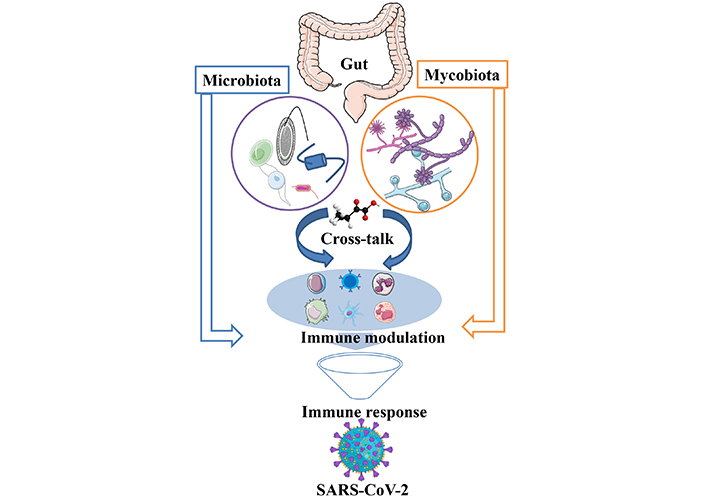
Keywords
Gut mycobiota, gut microbiota, COVID-19, inflammation, immune response, PACSIntroduction
Inflammation is a crucial component in developing a strong and effective immune response against pathogens. The initiation of immune cell recruitment is triggered by pathogens or their components when they come in contact with host cell receptors. This is an essential event to trigger a cascade of inflammation. This novel SARS-CoV-2 infects humans by encompassing to the cell membrane of the host, which is mediated by spike glycoprotein [1]. This turns into causing respiratory, hepatic, and physiological dysfunction. In some infected conditions, the body generates an excessive immune response in the form of cytokines and chemokines. In many cases, this strong response is prolonged and is termed as the cytokine storm [2]. The SARS-CoV-2 is reported to have higher transmissibility and infectivity than Middle East respiratory syndrome (MERS-CoV). The majority of infected individuals are asymptomatic or have mild symptoms, which is possible because of their different levels of immune system activation in the host. High levels of viral load lead to conditions like; acute respiratory distress syndrome (ARDS), cardiac complications, organ failure, and death (Figure 1). Bacterial and fungal infections are one of the major concerns observed in patients with COVID-19. Spike protein mutation, deletion, and several other genomic mutations of SARS-CoV-2 make this virus more infectious and easier to escape medication. This intermittent mutation in the virus is randomly distributed across the world [3]. Multiple variants have been identified first: alpha (B.1.1.7), beta (B.1.351), gamma (P.1), delta (B.1.617.2), and omicron (B.1.1.529) [4]. The complexity of this pandemic is a serious concern to humankind, and it’s urgently needed to be addressed. As a single variant targeted drug does not suffice for this deadly disease, safe gut microbiota (GM), mycobiota, and their metabolites can be explored to address this challenge.
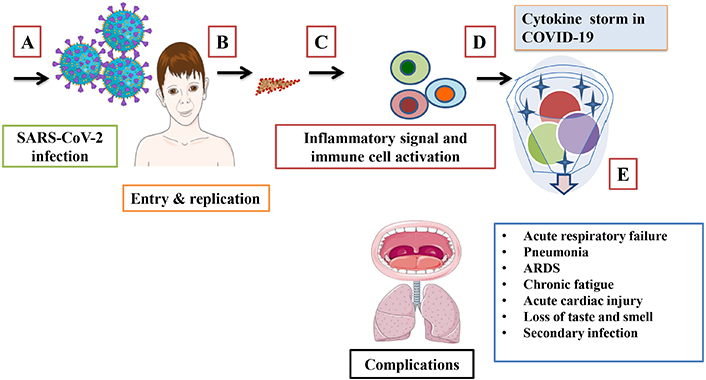
Schematic representation of SARS-CoV-2 infection mode, immune response, and complications. (A) Viral entry (B) replication inside the host, (C) excessive immune response, (D) cytokine storm formation, and (E) associated complications. ARDS: acute respiratory distress syndrome. Parts of the figure were used from or adapted from pictures provided by Servier Medical Art, licensed under CC BY 4.0
GM and immune interplay
The GM is a collection of diverse communities of microbes that reside in trillions of numbers inside the gut, outnumbering our body cells, which live in a symbiotic relationship with their host. They inhibit the pathogen’s colonization properties and provide protection, as well as play a crucial role in immune regulation. GM is linked to viral infections such as chronic hepatitis C (HCV) and chronic hepatitis B (HBV). This is significantly correlated with the liver diseases that take place during disease progression. A significant alteration in the abundance of GM at species, genus, and phyla levels has been reported in disease-infected patients compared to healthy controls. At the phylum level, there is an increase in Bacteroidetes and a decrease in the level of Firmicutes [5]. Immune cells such as macrophages and natural killer (NK) cells exhibit PRRs that recognize PAMPs expressed by microbes. This interaction is required to develop an immune response [6]. A study in a germ-free model animal suggests that GM deficient mice model with a high difference in metabolic and immunological parameters as compared to conventional animals having normal gut flora [7]. The GM is well known for shaping metabolic functions, generating immune responses, and deciphering the signal to decide the healthy state and disease condition of an individual. The health of an individual is often decided by their physical activity and the kind of diet they take on a daily basis. Together, they contribute to the gut microbial diversity. However, the microbial colonization starts in our gut at the time of birth. The infant’s intestines are believed to be sterile or contain a very low level of population at the time of birth. This is very essential for early gastrointestinal and immune development. The colonization of such microbiota may vary with the delivery mood of infants, such as natural birth and via cesarean section birth. Additional factors, such as tenure of breastfeeding, may impact the level of colonization [8]. A rapid colonization starts in the gastrointestinal tract (GIT) during and after delivery, as the neonate passes through the birth canal of the mother where they are exposed to the microbial population of the mother’s vagina and show similar microbial diversity to his or her mother [9].
The microbial diversity and their number differ from species to species, and individual to individual at different stages of age. The mammalian GM is highly variable at lower taxonomic levels however, there are major reported dominant phyla such as Bacteriodetes, Firmicutes, Actinobacteria, and Proteobacteria [10]. In immunological response, PRRs act as key factors that recognize microbial pathogens by recognizing the molecular structure pattern of PAMPs and DAMPs (damage-associated molecular patterns). This is released by the damaged and stressed cells undergoing necrosis that act as a danger signal to generate the inflammatory response. The Toll-like receptors (TLRs), NLRs (NOD-like receptors), C-type lectin receptors (CLRs), and various cytoplasmic proteins like RIG (retinoic acid-inducible gene) are linked in disease pathogenesis and inflammation. In dysbiotic conditions, the TLRs sense different kinds of ligands and initiate the inflammatory process. The NLRs, also known as NOD (nucleotide-binding oligomerization domain), have receptors, which are major components of the innate immune system. These NLRs cross-talk with TLRs to trigger nuclear factor kappa—light-chain enhancer of activated B cells (NF-kB) activity. This cascade activation is required to mediate inflammation and play a crucial role in generating an effective immune response against pathogens [11].
Mucus and commensal bacteria enhance barrier function that restricts viral entry. In general, gut-derived antimicrobial peptides and bacteriocin train the host immune system to generate an effective response at the time of pathogen activation by dendritic cells (DCs), which provide critical signals for immune cell development such as B lymphocytes and NK cells (Figure 2). This active and trained version of the immune system tends to have an early response and clearance of virus-infected cells that help in IgA production that is known to play a vital role in SARS-CoV-2 neutralization. IgA completes this neutralization just before it reaches epithelial cells. GM has been shown to have antiviral activity via IFN response modulation that regulates the homeostatic IFN level while the infection state of the host is regulated, which requires triggering a prompt antiviral response during infection [12].
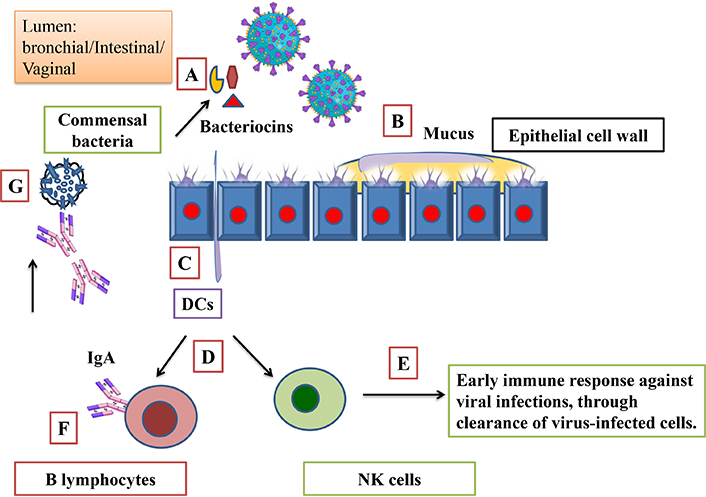
Protective role of gut microbiota and mediated immune modulation. (A) Bacteria derived bacteriocin acts as an immunobiotic. (B) Mucus and commensal bacteria enhance barrier function. (C) Dendritic cells (DCs) activation by antimicrobial peptide and bacteriocin. (D) Activated DCs provide critical signal for immune cell development such as B lymphocytes and natural killer (NK) cells. (E) Early immune response and clearance of virus-infected cells. (F) B lymphocytes activation by DCs, IgA production, and (G) SARS-CoV-2 neutralization by IgA. Parts of the figure were used from or adapted from pictures provided by Servier Medical Art, licensed under CC BY 4.0
High genome mutation is a serious concern associated with viral replication. This helps the virus to re-infect and adapt against the first immune response generated by the host. Therefore, preventive and supplementary interventions can be used to counter viral load and ensure an adequate immune response. High doses of antibiotics altered GM which trigger microbial pathogenesis. Due to the immunobiotic nature of GM, they offer potential health benefits and strengthen the immune response. The microbial groups such as Akkermansia muciniphila, Faecalibacterium prausnitzii, Bifidobacterium, and Lactobacillus significantly known to bolster immune function for a potential medical value with an inflammation remission response [13]. GM-derived metabolites such as biosurfactants, hydrogen peroxide, nitric oxide, lactic acid, and other organic acids are known for their inhibitory effects on viral proliferation [14]. Immune response during COVID-19 infection was also linked with metabolites produced by the GM, specifically amino acids, sugar metabolites, and neurotransmitters [15].
GM had the potential effect on COVID-19 vaccination, concerning developing the appropriate immune response while at the same time lowering its adverse effects. Bacteria such as Bifidobacterium adolescentis act as adjuvants and are known for their immunomodulatory role that enhances neutralizing antibodies in vaccinated individuals [16]. Studies suggest the potential outcome of GM modulation and supplementation of metabolites as ameliorate tools to diminish post-vaccination immunogenicity and other vital activity that is required for lowering its adverse effects [17]. High abundances of Bifidobacterium are likely to enhance the immunogenicity of vaccines [18]. In-vivo models and clinical studies have also shown that GM may influence the immunogenicity of the vaccine and, thus, its effectiveness [19].
Gut mycobiota in COVID-19
Gut mycobiomes are small biospheres that are often less studied, for the role that they play in their host. The healthy human gut contains Candida and Saccharomyces species, with other frequently detected taxa including Malassezia, Debaryomyces, Cladosporium, Aspergillus, Pichia, and Alternaria [7]. According to metagenomics data, fungi contribute around 0.01–0.1% of the total human gut mycobiomes [20]. This flora is involved in the production of primary and secondary metabolites, which play a crucial role in various biological activities as well as antimicrobial activities [21]. Gut mycobiomes diversity and abundance also vary due to the different types of SARS-CoV-2 variant infection. Mainly Aspergillus, Mucorales Candida auris and Candida species groups cause co-infections in COVID-19 [22]. A reduction in intestinal short-chain fatty acid (SCFA) was observed in infected individuals that resulted from an altered gut mycobiomes [23].
Viral infection is one of the major contributors to the disease-affected population globally due to fewer vaccines and antiviral drugs to combat infection. Several fungal extracts and their isolated components have shown inhibitory action on the virus, such as herpes simplex virus (HSV), papilloma virus (PV), and human immunodeficiency viruses (HIV) and viral particles or adsorption of the virus on cell surfaces that prevent their spread and infectivity [24]. Individuals with health complications such as diabetes and trauma are more prone to acquire the secondary infection mucormycosis is also widely termed as black fungus, which was reflected in the tissue of COVID-19 infected patients due to the invasion of non-pigut microbial oriented hyphae [25]. The gut mycobiomes remains constant at the time of SARS-CoV-2 infection in moderate instances, although changes continue with lower diversity for six months after recovery in severe cases [26]. In a balanced environment, the gut mycobiome and their cellular components, such as chitin, glycans, and glycoproteins act as protective players for the host. They train the immune system for reorganization in early entry and invasion by mimicking the similar immune pathway activation that our body follows in adverse conditions. This helps the host by preventing from secondary infection (Figure 3). Secondary infection added a high rate of mortality and morbidity rates to COVID-19 affected patients, where early diagnosis and treatment played a vital role in survival. On the other side, people with weak immune systems are highly prone to getting secondary infections due to their low neutrophils, which leads to mucormycosis. Characterization of this condition is mainly done by hyphal growth near the vicinity of blood vessels, which results in tissue infraction, thrombosis, and necrosis [27].
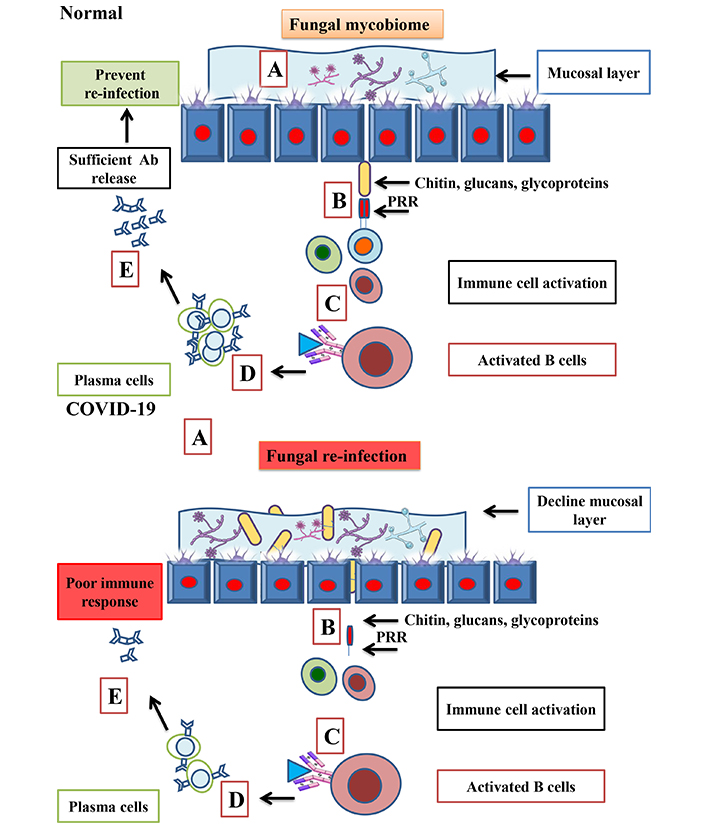
Protective role of gut mycobiome, in normal and in SARS-CoV-2 condition. In normal conditions, the presence of the fungal mycobiome (A) and their components triggers an immune response (B). A strong PRR (pattern recognition receptor) that prevents the host from re-infection by generating (C–E). In COVID-19, due to poor immune response leads to fungal re-infection and increases the level of fungal abundance in COVID-19 infected patients (A) that leads to poor immune response in the form plasma and Ab response (B–E). Ab: antibody. Parts of the figure were used from or adapted from pictures provided by Servier Medical Art, licensed under CC BY 4.0
Cross-talk and immune modulation in viral load clearance
A healthy gut environment is crucial for the host because it trains the immune system for a timely and optimal response. Some populations didn’t get severe complications during infection and recovered naturally due to their herd immunity. It has been reported that GMs such as gram-positive lactobacillus negatively impact the growth of Candida on a molecular level. Molecular mechanisms mediated via lactobacilli, not only inhibit growth but also can inhibit hyphal morphogenesis of their host [28]. Advances in understanding the epidemiology of COVID-19 have revealed that Staphylococcus aureus is involved in bacterial and fungal co-infection and has shown to contribute around 39.2% of total co-infection [29]. In normal conditions, the immune system remains active with the GM and mycobiota. The components such as monocytes, and macrophages act as memory characters for β-glucan and chitin. These fungal cell wall components protect their host from the next set of fungal infections due to their trained immunity [30]. Some of the gut metabolites, such as lactic acid and SCFAs particularly butyrate, partially inhibit the growth of Candida species and inhibit fungal growth and colonization [31]. SCFA is reported for its regulatory effect on Aspergillus spp. in the host [32]. SCFA also acts as a vital component for B cell metabolism in the gut and in some systemic tissue, which promotes infection-specific antibody production and lowers the COVID-19 progression [33]. SCFA, which is collectively present in a ratio of 3:1:1 (acetate, propionate, and butyrate) [34], butyrate enhances naïve T cell differentiation for T regulatory cell production that is required to counter pathogenic infection [35]. A similar role of propionate is reported in the case of Treg cell proliferation, which is mediated via GPR43 activation [36]. There are other ways by which this cross-talk protects our body from pathogens, whether it’s a bacterial infection or viral infection. This immunoprotective function is very important to trigger an appropriate immune response. Viruses such as poliovirus and reovirus have been known to use both lipopolysaccharide (LPS) and PG (peptidoglycan) for better thermostability and receptor affinity. This is equally important for other mechanisms that are required to facilitate in vivo infection [37].
To explore the connecting link between bacterial components and CoV infection, PG derived from Bacillus subtilis is shown to have low CoV infectivity. The molecule surfactin, which is a cyclic lipopeptide (CLP) that presents doses and dependent temperature-dependent virucidal properties, is responsible for CoV inhibition. Surfactin can reduce infection severity caused by other enveloped viruses, such as influenza A, Zika, Dugbe, Nipah, and Ebolavirus [38]. This indicates the important role of commensal bacteria in increasing or decreasing viral load and infections, which reflects the potent role of the microbiota. Angiotensin-converting enzyme-2 receptor (ACE-2R) is believed to play a key role in facilitating SARS-CoV-2 entry.
More than 140 peptides are studied under clinical trials representing a promising hope to counteract pathogens potentially for coronavirus in general and SARS-CoV-2 [39]. Due to its properties of having protein-protein interactions, it can be used for diseases that are difficult to target. High specificity makes it a potent player with lower side effects; furthermore, many peptide inhibitors have been demonstrated to counteract efficiently viruses. GM and gut mycobiome cross-talk not only activate the immune system, but it also helps in viral protein clearance inside infected cells (Figure 4), first by physical means of protection in the form of mucous followed by immune activation in the vicinity of epithelial cells, where ACE2 that facilitates virus entry [40]. This influences the immune response to target infected host cells and prevents disease progression [41]. The antiviral mode of peptide action includes but is not limited to, blockage of host cell receptors, virolysis, and targeting via viral fusion or replication adaptive immune response. This helps in inhibiting virus-host cell fusion by cleaving the core protein [42].
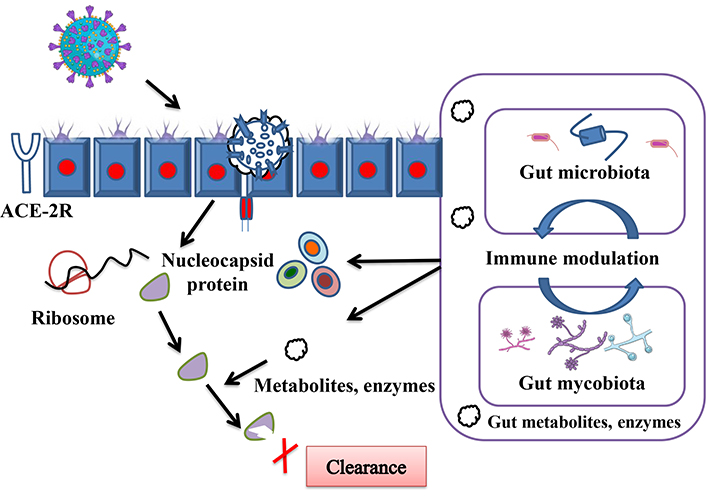
Depicted cascades of mechanisms by which gut microbiota and gut mycobiota cross-talk help the host in viral clearance, immune cell recruitment, and viral load clearance at the time of COVID-19 infection. Also highlighting possible roles for gut derived metabolites and enzymes, which participate in immune response and clearance of remaining viral proteins that escape from early immune response. ACE-2R: angiotensin-converting enzyme-2 receptor. Parts of the figure were used from or adapted from pictures provided by Servier Medical Art, licensed under CC BY 4.0
Entries of viruses trigger immune responses, which try to eliminate them at an early stage. This is confirmed by the high levels of inflammatory cytokines observed in severely infected patients. Gut bacteria produce enzymes like; β-glucuronidase, sulfatase, and various glycosidase deconjugate, compounds generated by such enzymes are readily absorbed across the intestinal wall [43].
A colonization resistance characteristic of gut bacteria reduces the probability of pathogens establishing their presence in the local gut environment. This helps the host by inhibiting physical attachment, and their growth is affected at the same time. In some conditions, gut microbes trigger early antiviral responses which protect the host from a high viral load. New findings suggest that germ-free mice possess impaired cytokine gene expression, this is more specific to type I interferon (T1I), T1I proteins are produced by different cells and are very critical to generating an effective host antiviral response. To support the role of GM, an ablation of gut microbial populations induced using antibiotics downregulates the capacity of the host to generate robust immune responses in viral infection. Further, it was confirmed that an influenza virus infection triggers more severe symptoms in antibiotic-treated mice compared with the untreated group. Collective studies highlight the importance of a healthy gut microbiome and its necessary role in generating appropriate antiviral defense [44]. Patient with high risk factors of COVID-19 is found rich in Aspergillus species, which contributes to pulmonary aspergillosis, an Aspergillus species load can be used as a marker for disease severity and timely treatment [45].
Post-acute sequelae of COVID
Post-acute COVID-19 syndrome (PACS) is a major global health concern, characterized by persistent symptoms and complications beyond the illness, where infected patients suffer from several adverse complications during disease onset and even after several months of recovery. Most of the symptoms associated with chronic alterations are neuropsychiatric, gastrointestinal, musculoskeletal, and endocrine. Gut dysbiosis contributes to the onset and progression of many viral diseases, including COVID-19 and post-COVID-19 manifestations [46]. The PACS results in conditions such as reinfection, physical fatigue, chest pain, dyspenea, and cognitive slowdown due to immune dysregulation [47]. The potential involvement of the GM in the pathogenesis of PACS. The gut dysbiosis and its association during the course of infection and disease severity are closely associated with COVID-19.
GM, dysbiosis during infection, which leads to alterations in microbial diversity and abundance, has been implicated in various systemic inflammatory conditions, including PACS. The GM plays a pivotal role in regulating immune responses, modulating inflammation, and maintaining gut barrier integrity. Dysbiosis-induced alterations in immune homeostasis and gut barrier function may contribute to the development of chronic symptoms observed in PACS [48].
Furthermore, the communication between the gut-brain axis, may influence neurological manifestations commonly reported in PACS. Emerging evidence also suggests a potential link between gut dysbiosis and the persistence of respiratory symptoms in PACS, including and exercise intolerance. Gut-lung axis communication mechanisms, involving immune cells, microbial metabolites, and neuronal pathways, could contribute to respiratory dysfunction in PACS.
Moreover, alterations in the GM composition may impact metabolic health and contribute to the development of metabolic abnormalities. Understanding this interplay between the GM and PACS pathophysiology could be promising for novel therapeutic targets. Restoring gut microbial balance, such as probiotics, prebiotics, and fecal microbiota transplantation (FMT), may offer potential avenues for mitigating symptoms and improving outcomes in PACS patients. However, further research is warranted to elucidate the specific mechanisms underlying gut dysbiosis in PACS and to evaluate the efficacy of microbiota-targeted interventions in clinical settings, which need to be further explored [49].
This cross-talk is a crucial determinant of immune response, metabolic regulation, and neurological functions during the pathogenesis of PACS. Targeted modulation of the GM could offer promising therapeutic approaches for managing PACS and improving patient outcomes [50].
Conclusions
The COVID pandemic has taught us so many lessons, about the medical infrastructure to tackle such pandemic, people are hesitant about vaccines and vaccine affordability per capita between countries [51]. A systematic, scientifically standardized, and comprehensive study of microbial and mycobial imbalances may be considered a novel approach to detecting the severity level in patients with COVID-19. As smaller size of virus and high infection rate is a major concern associated with this pandemic, technical integration such as antiviral picotechnology can also be incorporated to control [52]. Biophysical models like FDA-approved tumor treating fields which might help by preventing viral genomic replication, apoptosis in SARS-CoV-2 infected cells [53]. A close connection of the gut with the brain, immunological activity, and host physiology will help to understand the COVID-19-associated complications. The understanding of lung microbiota can also be explored for its potential as prognostic biomarker as its changes in the host with critical illness [54]. A deep dive understanding of the cross-talk between the microbiome and mycobiome, and their role in immune response will take scientific discovery knowledge to develop gut-targeted safe therapeutic approaches in the form of FMT probiotics, peptides, antibacterial, and antifungal metabolites. Combination of nutracutical and active pharmaceutical ingredients can be used as a modulatory agent for beneficial microbes that present in the host [55]. This will also give an idea to minimize the use of antibiotics and antifungal drugs. A collective study will open doors for the precise use of targeted drugs to replace the broad spectrum, which will help the gut to restore gut dysbiosis quickly. The gut microbiomes may be used in clinical settings as non-invasive biomarkers to identify individuals who are more susceptible to infection and its severity [15].
There are many anticipated studies that need to be undertaken that further investigate active metabolites and enzymes that flow between GM and mycobiota as a signaling medium to derive this cross-talk and how this cross bridge connects the brain and immune system that is required for virus clearance. Upon administration, these active metabolites and enzymes will promote normal cell function by preventing the interruption caused by viral nucleaocapcide protein, boosting immune response response, and normal cellular activity of the host. Studies will integrate the idea to develop safe and sustainable gut-oriented therapeutic tools. Elucidating this cross-talk between the gut and immune could help develop clinical strategies to identify individuals at risk, disease severity, and progression associated with PACS. Moreover, here we have highlighted the importance of targeting the GM composition to avoid dysbiosis and to develop therapeutic measures against COVID-19 and PACS. The prebiotics, probiotics, and FMT-based engraftment in COVID-19 can be explored as a crucial roadmap for a safe therapeutic approach, this will also minimize the use of antibiotics to avoid hurdles like drug resistance and associated side effects on health. Restoration of the axis between the gut, brain, and immune system will help timely response to infected individuals in speedy recovery via optimal immune functioning against mutated viral strains. Often a good policy on place plays a vital role in global health issues, and to face such global pandemic threats hence a well-designed effective public policy for better management of the pandemic will help to tackle future crisis [56].
Abbreviations
ACE-2R: | angiotensin-converting enzyme-2 receptor |
CLRs: | C-type lectin receptors |
DCs: | dendritic cells |
FMT: | fecal microbiota transplantation |
GM: | gut microbiota |
NK: | natural killer |
NLRs: | NOD-like receptors |
NOD: | nucleotide-binding oligomerization domain |
PACS: | post-acute COVID-19 syndrome |
PAMPs: | pathogen-associated molecular patterns |
PG: | peptidoglycan |
PRRs: | pattern recognition receptors |
SCFA: | short-chain fatty acid |
T1I: | type I interferon |
TLRs: | Toll-like receptors |
Declarations
Author contributions
SK: Conceptualization, Visualization, Writing—original draft. ZB: Conceptualization, Writing—original draft. SS: Conceptualization, Supervision, Validation, Writing—review & editing. All authors have read the journal’s authorship statement and approved the submission of the manuscript.
Conflicts of interest
The authors declare no conflict of interest.
Ethical approval
Not applicable.
Consent to participate
Not applicable.
Consent to publication
Not applicable.
Availability of data and materials
Not applicable.
Funding
SK is thankful to the Indian Council of Medical Research (ICMR) Government of India, and the Scheme of Developing High Quality Research (SHODH) Government of Gujarat for providing fellowship. ZB is thankful to the Nirma University, Ahmedabad for providing fellowship. The funders had no role in study design, data collection and analysis, decision to publish, or preparation of the manuscript.
Copyright
© The Author(s) 2025.
Publisher’s note
Open Exploration maintains a neutral stance on jurisdictional claims in published institutional affiliations and maps. All opinions expressed in this article are the personal views of the author(s) and do not represent the stance of the editorial team or the publisher.