Abstract
γδ T cells express unique T cell receptor (TCR) γ and TCR δ chains, with structural and functional heterogeneity. Taking advantage of the diverse γδ TCR repertoire or other ligand-receptor interactions, γδ T cells can recognize a broad spectrum of tumor-associated antigens (TAAs) in a major histocompatibility complex (MHC)—independent manner, thereby activating downstream pleiotropic effects. γδ T cells recruited into the tumor microenvironment can act as effector cells to mediate cancer immune surveillance. Their advantage lies in the ability to perceive tumors with a low mutation load, thus establishing the first line of defense against pathogens. Activated γδ T cells exhibit strong cytotoxic activity and cytokine secretion functions and are effective antitumor lymphocytes with simple and direct recognition modes and rapid responses. However, the clinical application of tumor—infiltrating γδ T cells has certain limitations. First, γδ T cells exposed to complicated cytokine networks are potentially affected by multiple inhibitory mechanisms. Additionally, these cells show highly flexible and dynamic plasticity and are extremely easily polarized into regulatory phenotypes. This review further emphasizes the diversified cross-talk between γδ T cells and other immune cells. Effective immunity of the body is often manifested by counterbalance under mutual restriction. Therefore, an in-depth understanding of γδ T cells that play conflicting roles in the tumor microenvironment is necessary. These cells may be a key factor ultimately mediating the deviation of the antagonistic response between tumor inhibition and tumor promotion. Finally, it retrospectively analyze the activation strategies and clinical relevance of existing γδ T cell adoptive immunotherapies. According to current challenges, there is a need to explore innovative immunotherapies, maximize the tumor-killing efficacy of γδ T cells, and attenuate or eliminate tumor immunosuppression. It is hoped that the host immune status can be accurately predicted and gradually advance γδ T cell precise individualized medicine.
Keywords
γδ T cells, tumor microenvironment, plasticity, cytokines, immunosuppression, cross-talk, precision immunotherapyHeterogeneity of human γδ T cell subsets
Classification of diverse γδ T cell receptor repertoires
At present, the phenotypic heterogeneity and functional variation of human γδ T cells are increasing. It is of great significance to gain insight into the classification of γδ T cell subsets. In this review, we use the Heilig nomenclature to describe human γδ T cells [1].
Similar to αβ T cells, γδ T cells also develop in embryos, and their intra-thymic development shows strict regulation and flexible plasticity [2]. During thymic ontogeny in fetal development, both types of T cells originate from a common lymphoid precursor cell, while γδ T cells emerge before αβ T cells at early stages and predominate the CD3+ population [3]. Naive γδ T cells generally express polyclonal T cell receptors (TCRs) and then migrate to different peripheral tissues. The pairing of δ-chain variable region 1 (Vδ1) chains with different Vγ chains appears first and is preferentially enriched in the intestinal epithelial tissue. Subsequently, γδ TCRs undergo TCR switching and produce diverse TCR repertoires [4]. The frequency of γδ T cells gradually decreases after birth, accounting for only 0.5–16% of human circulating T lymphocytes. Instead, αβ T cells dominate. In addition, the distribution of γδ T cells varies greatly among different species [5]. In some nonprimates, such as cattle and rabbits or Macaca mulatta, γδ T cells can reach 60% of peripheral blood CD3+ T cells. Moreover, functional and effective Vγ9Vδ2T cells are only found in higher primates and do not exist in mice used in preclinical studies [6]. Therefore, discrepancies in biological evolution have consistently been the main obstacle in the study of human γδ T cells.
The γδ TCR gene family is highly evolutionarily conserved [7]. Similar to the human αβ TCRs TCRα (Chr14, q11.2)/TCRβ (Chr7, q34), the individual γδ TCRs TCRγ (Chr7, p14)/TCRδ (Chr14, q11.2) develop into heterodimers through somatic rearrangement of the variable (V), diversity (D) and joining (J) gene segments mediated by recombination activating genes (RAGs) [8]. Generally, the TCR δ chain undergoes V-J rearrangement, and the TCR γ chain is assembled via V-D-J rearrangement. The preferential access of a distinct set of V, D, and J segments, flexible D-D segment connections, extensive N-region nucleotide additions, and junctional variability together constitute the structural hyperdiversity of the γδ TCR repertoire and even surpass that of αβ T cells [9]. All individuals show a highly personalized γδ T cell repertoire. Among these genes, TCR γδ complementarity determining region 1/2 (CDR1/2) may reflect the relative germline D. Their high biodiversity focuses on the CDR3, which forms the center of the antigen-binding site. Unlike αβ T cells, the length of the CDR3 δ chain is not as limited and is more V in γδ T cells, laying the foundation for MHC nonrestriction and the abundance of antigen recognition. Theoretically, approximately 1016–1020 unique γδ TCRs can be recombined in humans [10]. Unexpectedly, the detected γδ TCRs are usually arranged in a fixed pattern instead of random combination and permutation, and they are much more constricted.
The biased use of certain TCR gene segments of individual γδ T cells is relevant to tissue type and localization. In humans, different γ-chain V region (Vγ) gene segments (Vγ2, Vγ3, Vγ4, Vγ5, Vγ8, Vγ9, and Vγ11) and Vδ gene segments (Vδ1, Vδ2, Vδ3, Vδ5) rearrange to combine various subtypes of γδ T cells [11]. These subtypes mainly contain two cell populations, peripheral blood γδ T cells and organized lymphoid tissue-resident (thymus, tonsil, lymph nodes, and spleen) γδ T cells. The latter are abundant in epithelial and mucosal tissues, such as the digestive tract (20–40% of intestinal intraepithelial T cells) and female reproductive tract (10–20% of CD3+ T cells) [12]. This section will emphasize Vγ9Vδ2 T cells, Vδ1 T cells, and other non-Vγ9Vδ2 T/non-Vδ1 T cells.
Vγ9Vδ2 T cells
Human Vδ2 preferentially and strictly combines with Vγ9 to form Vγ9Vδ2 T cells [13]. They are the most well-known γδ T cell subgroup, ranging from 50–90% in the peripheral blood of most healthy individuals. Vγ9Vδ2 T cells are a crucial type of unconventional effector cell and are the first-line barrier against infections such as mycobacteria and Epstein-Barr virus (EBV) infections and solid tumors [14]. In view of their strong immune surveillance effect and ability to kill cancer stem cells (CSCs), they have been widely exploited for clinical immunotherapy [15]. They exert antitumor effects by inhibiting cancer cell proliferation, preventing angiogenesis, and increasing apoptosis. They have been clinically proven to have curative effects on lymphoma, myeloma, liver cancer, lung cancer, and ovarian cancer [16, 17].
Vδ1 T cells
The role of Vδ1+ γδ T lymphocytes in the tumor microenvironment (TME) is ambiguous and controversial. Circulating Vδ1 T cells consistently exhibit cytotoxic and regulatory properties concurrently, which makes them a hotspot for researchers [18]. In contrast to Vγ9Vδ2 T cells, Vδ1 T cells do not show a specific selection of Vγ chains, displaying diverse combinations. Human Vδ1 T cells constitute only a small population of peripheral blood but account for the majority of tissue-resident or mucosal-associated lymphoid tissue γδ T cells [19]. They function to maintain the integrity of normal epithelial tissue, including the skin (Vδ1), intestine (Vδ1 and Vδ3), liver, and spleen. Their presence is also detected in the peripheral blood of human immunodeficiency virus (HIV)-infected and B-cell chronic lymphocytic leukemia (B-CLL) patients, with an increasing frequency of Vδ1 T cells and an inversion ratio of Vδ2/Vδ1 [20].
Non-Vγ9Vδ2 T and non-Vδ1 T cells
The specific functions of non-Vγ9Vδ2 T and non-Vδ1 T cells have not been clarified thoroughly. Vδ3 T cells and Vγ4Vδ5 T cells are more commonly mentioned at present. Vδ3 T cells were mainly enriched in the liver and intestinal epithelium [21]. Trace amounts can be detected in the peripheral blood of patients with chronic viral infections and leukemias. Vδ3 T cells are glycolipid-responsive T lymphocytes that recognize CD1d+ target cells but not CD1a+, CD1b+, or CD1c+ cells. This process will lead to CD107a+ degranulation. Decreased expression of CD1d on myeloma or primitive neuroectoderm cells is conducive to immune escape. In regard to killing theory, Vδ3 T cells closely resemble natural killer (NK) T cells. Both can be used as adjuvants to trigger the differentiation of immature dendritic cells (DCs) into antigen-presenting cells (APCs) that are capable of activating naive T cells.
The team of Petrasca et al. [22] used positively labeled magnetic beads and fluorescent sorting to purify Vδ3 T cells. Practically, they only account for 0.6% of lymphocytes in freshly isolated peripheral blood mononuclear cells (PBMCs). Utilizing a medium system filled with irradiated allogeneic PBMCs, phytohemagglutinin (PHA), and interleukin-2 (IL-2), Vδ3 T cells were amplified 1,000 times in vitro. Interestingly, this study concluded that Vδ3 T cells can induce a 2.6-fold increase in immunoglobulin M (IgM) production by B cells, which may be one of the special features of Vδ3 T cells.
Vγ4Vδ5 T cells primarily appear in the peripheral blood. The Vγ4Vδ5 TCR can directly bind to the endothelial protein C receptor (EPCR) on epithelial tumor cells [23]. Furthermore, Vδ4, Vδ6, Vδ7, and Vδ8 T cells have been observed in the peripheral blood of lymphoma patients.
Classification by cell surface markers
Relatedly, we can identify and distinguish γδ T cells into four subtypes according to the classification determinants (CD) expressed on the cell surface. CD27 is also called T-cell activation antigen or tumor necrosis factor (TNF) receptor superfamily 7 (TNFRSF7) [24]. It can activate nuclear factor-kappaB (NF-κB) and the mitogen-activated protein kinase 8/c-Jun N-terminal kinase (MAPK8/JNK) signaling pathway by binding with the CD70/CD27 ligand, which is essential for the survival and long-term maintenance of activated T cells. Protein tyrosine phosphatase receptor type C (PTPRC) is also known as CD45 antigen, originally named leukocyte common antigen (LCA) [25]. It functions by activating the proto-oncogene tyrosine-protein kinase Src. CD45 isoforms are quite well conserved in mammals and specifically expressed according to the cell type and differentiation stage.
The four subgroups are the naive phenotype (γδ Tnaive, CD27+CD45RA+), the central memory phenotype (γδ TCM, CD27+CD45RA−), the effector memory phenotype (γδ TEM, CD27−CD45RA−) and the terminally differentiated phenotype (γδ TEMRA, CD27−CD45RA+). γδ TEM lacks the chemokine receptor 7 (CCR7) lymph node homing receptor, so it is a memory subpopulation that is commonly found in lymph nodes and the peripheral circulation [26]. These cells maintain an important self-renewal ability and are crucial for a quick immune memory response after encountering the same antigen stimulation. γδ TEM and γδ TEMRA primarily play a role in acute inflammation, acting as CD8+ variants [27]. They confer powerful instant cytotoxicity against pathogens, including viruses, bacteria, and cancer cells, by secreting cytokines [28].
Pleiotropic effects of γδ T cell ligand recognition and activation stimulus
It is universally known that conventional T cells bearing αβ TCRs generally recognize antigen peptides loaded onto MHCs or human leukocyte antigen (HLA). However, interactions between γδ TCRs and corresponding ligands are typically independent of antigen processing or presentation, not considering MHC/HLA restriction. Therein lies the distinction between antigen recognition and activation patterns. Multiple patterns can activate Vγ9Vδ2 T cells and Vδ1 T cells, where direct TCR γδ recognition or other receptor interactions account for the majority. In addition, butyrophilin (BTN) 3A (BTN3A)—related molecules are absolutely necessary. They work individually or synergistically to activate particular γδ T cell subsets, generating the appropriate signals.
Direct TCR γδ recognition of TAAs
Human peripheral blood Vγ9Vδ2 T cells exert TCR-dependent activation of nonpeptide phosphorylated antigens [phosphoantigens (pAgs)]. The two representative pAgs are (E)-4-hydroxy-3-methyl-but-2-enyl pyrophosphate (HMB-PP) and isopentenyl pyrophosphate [IPP, or isopentenyl diphosphate (IDP)]. HMB-PP is an essential metabolite of the nonmevalonate pathway [2-C-methyl-d-erythritol-4-phosphate (MEP) pathway] in most pathogenic bacteria and is an absent intermediate in the human host. IPP is generated via the 3-hydroxy-3-methylglutaryl-coenzyme A (HMG-CoA; β-hydroxy β-methylglutaryl-CoA) reductase pathway classically in eukaryotic cells, commonly called the mevalonate pathway [29]. The mevalonate pathway starts with a carbon feed stock acetyl-CoA and ends with the production of two five-carbon building blocks: IPP and dimethylallyl diphosphate (DMAPP). IPP can also be synthesized in the MEP pathway of isoprenoid precursor biosynthesis. Apart from the typical pAgs, there are other antigens such as adenylated, thymidylated, and uridylated adenosine 5’-triphosphate (ATP) derivatives. In the physiological state, human Vγ9Vδ 2 T cells can be activated by minute bioactivity (< 1 nm) of HMB-PP. Their effectiveness is 10,000–10,000,000 times stronger than that of other natural activators (such as IPP or alkyl amines).
γδ TCRs can sense cells undergoing stressful or transformed conditions. Moreover, an evolutionary protective mechanism is present in the human body; that is, the levels of some natural metabolites are so low that they cannot be recognized as danger signals by Vγ9Vδ2 T cells, thus preventing overactivation of weak stimuli in the basal metabolism. Metabolic disorders usually exist in malignant tumor cells, which initially guarantee an energy supply and lead to a high upregulation of pAgs. Therefore, that content is sufficient to activate Vγ9Vδ2 T cells, and Vγ9Vδ2 T cells can rapidly respond to either exogenous infection or endogenously transformed cells. In clinical trials, the combination of aminobisphosphonates (N-BPs), such as pamidronate (PAM) or zoledronate combined with low-dose recombinant human IL-2, can achieve selective activation and expansion of Vγ9Vδ2 T cells in vitro [30]. The underlying mechanism consists of inhibiting the rate-limiting enzyme in the isoprenoid pathway, called farnesyldiphosphate synthase/farnesylpyrophosphate synthase (FDPS/FPPS), and causing IPP accumulation. This mechanism can dramatically boost the sensitivity and cytotoxicity of Vγ9Vδ2 T cells to dysfunctional tumor cells.
Current studies indicate that pAgs are not recognized directly by γδ TCRs but produce a marked effect by binding to specific proteins. Several molecules with relatively certain interactions are BTN3A1, CD277, and F1-ATPase [combined with apolipoprotein A-I (Apo A-I)] [31]. The Vγ9Vδ2 TCR is capable of recognizing the stable F1-ATPase/ApoA1 complex instead of ApoA1 alone. This complex is usually expressed on the inner mitochondrial membrane. However, it is ectopically overexpressed in tumor drug-resistant cells, especially in a human leukemia cell line (K562) and lymphoma (Raji). F1-ATPase functionally participates in the production of the acidic TME, promoting tumor invasion and metastasis. The specific role of the BTN family will be described in detail in the following section.
Regarding the molecular mechanism of Vγ9Vδ2 T cell phosphorylation activation, Hayday [4] suggested that crossing CD3 and pAgs can activate phosphatidylinositol 3-kinase (PI3K)/extracellular signal-regulated kinase (ERK)/MAPK signaling, thus triggering a series of NF-κB downstream reactions involved in the release of interferon-γ (IFN-γ) and TNF-alpha (TNF-α). Vγ9Vδ2 T cells are activated by the MAPK signaling pathway to upregulate the expression of scavenger receptor B2 (SR-B2) CD36b [32]. CD36 is heavily involved in γδ T cell migration and signaling, together with protecting the host against bacteria, fungi, and parasites. It is closely related to homeostasis, apoptosis, inflammatory diseases, and pathogen clearance.
In addition to phosphorylated antigen, Vγ9Vδ2 T cells can also recognize the cell stress-induced annexin A2 and ephrin receptor A2 (EphA2) through γδ TCRs [33]. Cells may have undergone a transformation, cytomegalovirus (CMV) infection, or exposure to stressors such as heat. In addition, occasional cross-reactions in ligand recognition are observed for γδ TCRs, which are interpreted to represent an Ig-like antigen recognition pattern. For example, nonself ligands such as viral glycoprotein 9, tetanus toxoid, Igλ light chain, herpes simplex virus glycoprotein I, and staphylococcal enterotoxin A are all stimulants capable of activating γδ TCRs.
γδ T cell activation through other receptor-ligand interactions
In addition to the participation of γδ TCRs, other receptors, especially NK receptors (NKRs) and Toll-like receptors (TLRs) are prime characteristics of γδ T cell activation. Deng et al. [34] proposed that NKRs expressed on γδ T cells were suspected to be costimulatory receptors of γδ TCRs, similar to NK cells. These NKRs strictly control the potential high self-reactivity of γδ T cells by activating and inhibiting the NKR balance.
On the one hand, the NK group 2D (NKG2D) pathway exerts a synergistic and cumulative stimulating effect by promoting the secretion of cytotoxic factors, accelerating cell proliferation, and upregulating the calcium mobilization pathway [35]. Specifically, UL16-binding protein 4 (ULBP-4), stress-induced MHC class I polypeptide–related sequence A and B (MICA/B) and human mutS homolog 2 (hMSH2) molecules are well defined and participate in the induction of Vγ9Vδ2 T cell killing activity through γδ TCR and NKG2D [36]. Their appearance is usually accompanied by DNA damage. Research has shown that the cytolytic toxicity of human intestinal epithelial Vδ1 T cells is more susceptible to lymphoma cells that express ULBP1 at high levels [33]. Vδ1 T cells also recognize ULBP3 expressed on B-CLL through NKR, thus significantly preventing tumor progression. On the other hand, NKG2D can work independently of γδ TCRs. Blocking the NKG2D pathway can directly inhibit the cytotoxic effect of Vγ9Vδ2 T cells on hematological tumors.
Moreover, some inhibitory NKRs negatively regulate Vγ9Vδ2 T cells, such as the NKG2A/CD94 heterodimer, NKG2C/CD94 heterodimer, and Ig-like transcript 2 (ILT-2), should be considered [37]. They belong to the lectin-like receptor family and the Ig family, aiming to suppress the immune response that targets themselves.
Certain Vγ9Vδ2 T cells express the natural cytotoxic receptor NKp44, mediating cytotoxic activity against multiple myeloma (MM) cells. NKG2D ligands are lacking in the expression on such cells. Previous studies have indicated that in the presence of IL-2 or IL-15 stimulation, Vδ1 T cells can also recognize B7 family members B7-H6 through NKp30, NKp44, and NKp46 and strongly secrete IFN-γ [38]. This is a relatively unique feature that has been confirmed in acute myeloid leukemia (AML). B7-H6 is also called natural cytotoxicity triggering receptor 3 ligand 1 (NCR3LG1). No B7-H6 expression is detected in any normal tissues. It selectively appears on several tumor cell lines, including T and B lymphomas, melanomas, myeloid leukemias, and large T simian virus 40 (SV40) antigen-transformed cells, at the protein level. B7-H6 specifically triggers natural cytotoxicity receptor-3 (NCR3)—dependent NK cell activation but does not interact with NCR1, NCR2, killer cell lectin like receptor K1 (KLRK1), or other NK cell-activating receptors. Interactions between B7-H6 and NKp30 contribute to the activation and cytotoxicity of NK cells or γδ T cells.
TLRs are also an important link between innate and adaptive immunity through their presence in Vγ9Vδ2 T cells and DCs [39]. Via myeloid differentiation primary response 88 (MyD88)-dependent and Toll/IL-1R domain-containing adaptor-inducing IFN-beta (TRIF)-dependent pathways, TLRs recognize molecules that are constantly associated with threats (cell stress and pathogens). Their advantages lie in a high degree of focalization, pertinence, and specificity and would not misidentify the normal expression of autoantigens under physiological conditions. Activation by TLRs can cause cytokine production, proliferation, and survival to help Vγ9Vδ2 T cells fight unfavorable factors [40]. TLRs may be explored as cooperative drugs for cancer immunotherapy in the future.
DNAX accessory molecule-1 (DNAM-1; CD226), an NK-associated cell surface receptor, is also involved in regulating the activation of Vγ9Vδ2 T cells [41]. DNAM-1 mediates cellular adhesion via its ligands, CD112/Nectin2 and CD155/Nectin-like-5, which are mostly expressed on hepatocellular carcinoma cells. Upon ligand binding, it promotes T cell proliferation and cytokine production, including that of IFN-γ, IL-2, IL-5, IL-10, and IL-13 released by cytotoxic T lymphocytes (CTLs), NK cells, and Vγ9Vδ2 T cells (a diagram is displayed in Figure 1).
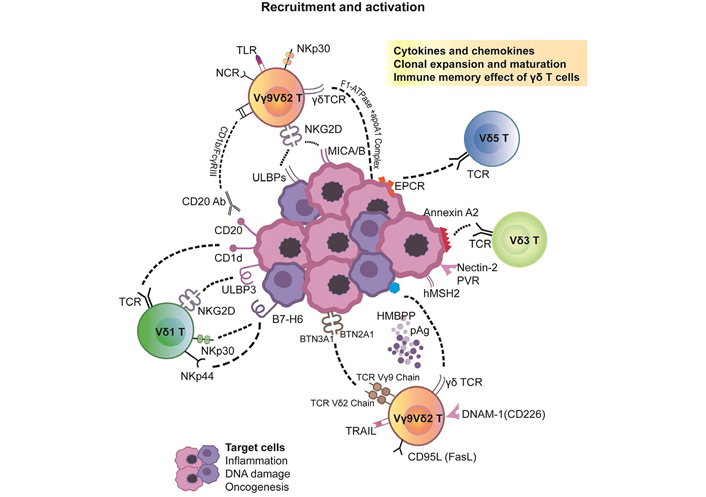
Pleiotropic effects of γδ T cell ligand recognition and activation stimulus. It mainly includes the following two parts. 1. Direct TCR γδ recognition of TAA. 2. γδ T cell activation through other receptor-ligand interactions. Among them, BTN3A based Vγ9Vδ2 TCR selection and activation played a pivotal role. TRAIL: TNF-related apoptosis-inducing ligand; PVR: proliferative vitreoretinopathy
BTN3A-based Vγ9Vδ2 TCR selection and activation
BTN is a family of proteins, including three types of BTN1A1, BTN2A2 (BTN2A 1/2), and BTN3A1 (BTN3A 1/2/3) [42]. BTN1A1 is involved in regulating the secretion of milk-lipid droplets of mammary gland epithelial cells. Here, we mainly emphasize the effects of BTN3A1 and BTN2A1 on the phosphorylation activation of Vγ9Vδ2 T cells. Neither are specifically expressed in certain tissues but are widely present on tumor cells without genetic restriction. They participate in the metabolism of lipids, fatty acids, and sterols and serve as necessary prerequisites for the recognition of pAgs by Vγ9Vδ2 T cells. The Vγ9 region recognizes BTN2A1, and the Vδ2 region recognizes BTN3A1. The exploration of BTN3A1 is more detailed [43].
The BTN genes are a group of MHC-related genes encoding type I membrane proteins with 2 extracellular Ig domains and an intracellular B30.2 domain (PRYSPRY). The B30.2 domain is a positively charged pocket that can directly bind pAgs. By forming the BTN3A1-pAgs complex, the structure of the extracellular dimer of BTN3A1 will change to directly activate Vγ9Vδ2 TCRs [44]. Another theory is to present pAgs to the surface of Vγ9Vδ2 T cells to perform the activation function. The biological molecular basis of BTN3A1 enables it to be a real-time sensor for measuring phosphoantigen levels in cells [45]. Therefore, we generally reach a consensus that BTN-related molecules promote the activation and proliferation of Vγ9Vδ2 T cells by the precise perception of phosphorylated metabolites (such as IPP), thereby regulating the release of IFN-γ or other cytokines. This process is vital for γδ T cell activation and the adaptive immune response (Figure 1).
Plasticity, polarization or reprogramming of γδ T cells in the TME
γδ T cells have structural plasticity and acquired functional plasticity [46]. When exposed to different constantly changing environments, γδ T cells undergo permanent or nonpermanent changes in morphology, physiology, and even functionality. Their plasticity can be understood as a basic evolutionary adaptive behavior.
Naive γδ T cells have acquired a restricted homologous γδ TCR repertoire, directional tissue homing, and definite functional characteristics during their development in the thymus. Innate plasticity is independent of the unique infection that human individuals encounter in the periphery and will not produce long-term functional effects. The inherent plasticity of different γδ T subsets often depends on their surface receptors, the intensity of the activated TCR signaling, and different inflammatory stimuli. In one scenario, the δ chain, which is mainly used to distinguish functional subsets of γδ T cells, has been demonstrated to have germline-dependent receptor encoding and organ-specific selection [47]. On the other, innate plasticity is usually not a succession of compound responses. For example, in the absence of cytokines, the cytotoxic lysis function of Vγ9Vδ2 T cells triggered by NKG2D alone is relatively limited and only suppresses the survival of target cells to a small extent. In another example, IL-12 or interferon type I released by stimulated myelomonocytic cells in response to TLR3 and TLR4 can trigger only a small amount of IFN-γ production by Vγ9Vδ2 T cells.
The acquired plasticity of γδ T cells is a very fascinating topic worth studying. Since peripheral γδ T cells respond to diverse environmental elements, they differentiate to form specific polyclonal γδ T cells, reflecting tremendous individual differences. This phenomenon may be involved in the inconsistency of adoptive immunotherapy of Vγ9Vδ2T cells in the clinic. Researchers first showed solicitude for the preferential polarization of γδ T cells into T-helper 1 (Th1)-like cells, which exist as enemies of tumors. As the tumor develops to a later stage, the consequences of recruited γδ T cells mainly depend on the complex TME, whether it is antitumoral or protumoral, where a variety of polarization factors can shape regulatory cells [48]. Functional polarization of γδ T cell subsets is reversible.
The TME
In recent years, the theory of “seed and soil” has laid a foundation for the research field of TME and has been revisited by Witz [49]. Specific cancers, represented as seeds, can migrate to other physiological sites (soil) based on the similarity of the primary and secondary tumor environments. The TME includes tumor cells and surrounding blood vessels, immune cells, fibroblasts, extracellular matrix (ECM), and signaling molecules. The TME is a heterogeneous soil that maintains the survival of tumor cells. Arneth [50] found that the same cancer cell line inoculated in mice caused more rapid tumor development than in vitro. It is also regrettable that the transplantation therapy of autologous tumor-infiltrating lymphocytes (TILs) is only successful in melanoma. Cancer cells themselves induce apoptosis of activated T cells and NK cells by secreting exosomes containing Fas ligand (FasL) and TRAIL. This evidence indicates that the TME actively counter regulates the effector functions of immune cells, which is conducive to tumor progression, invasion, and metastasis. This function induces peripheral immune tolerance and accelerates immune escape from the host immune system.
γδ TILs are γδ T cells that penetrate tumors. Their fate lies in the TME. In the next section, we will describe some factors responsible for subverting the antitumor immunity of γδ T cells.
Cancer-associated fibroblasts and ECM remodeling
Stromal cells refer to the nonmalignant cells in the TME. In a specific tumor, up to 90% of the cells may be stromal cells, and the rest are cancer cells. It is generally believed that the matrix is rich in fibroblasts, T cells, macrophages, and endothelial cells, which accelerate cell cycles to promote tumor development. Among them, cancer-associated fibroblasts (CAFs) are the critical element. CAFs mostly originate from normal fibroblasts or through epithelial-mesenchymal transition (EMT) or endothelial-mesenchymal transition (EndMT) [51]. First, CAFs secrete insulin-like growth factors 1 (IGF-1) and IGF-2 to assist cancer cell survival. By generating matrix metalloproteinases (MMPs) and mediating the biosynthesis of C-X-C motif chemokine ligand 12 (CXCL12), CAFs destroy the ECM and form loose fibronectin and collagen regions, thereby limiting the distribution of γδ T cells in tumor tissues. In a preclinical mouse model of pancreatic ductal adenocarcinoma (PDAC), CAFs can recruit myeloid progenitor cells through chemokines and high levels of stromal cell-derived factor 1 (SDF1) [52].
Recently, studies in non-small cell lung cancer (NSCLC) have shown that CAFs constitutively express programmed death ligand-1 (PD-L1) and PD-L2 and induce effector T cell disability or Treg generation through the programmed cell death protein 1 (PD-1) signaling pathway [53]. SDF1 methylation and PD-L1 expression have been implicated in the progression of prostate carcinoma. The less mentioned mechanism is that CAFs can deliver lactic acid produced by aerobic glycolysis to cancer cells, which is called the reverse Warburg Effect. The TME is usually far from the blood supply and in a hypoxic environment. Under hypoxia, the mismatch repair (MMR) pathway is downregulated and inhibits DNA repair. Upregulation of hypoxia-inducible factor 1α (HIF1-α) induces increased angiogenesis and cell migration. All the above factors aggravate cancer instability.
γδ T cell recruitment and preferential migration into the TME
The consequences of immunotherapy are largely affected by the infiltration capacity of immune cells that are linked with the expression of chemokine receptors and integrins, which in combination determine the migration and tissue localization of TILs. In a variety of preclinical xenograft mouse models, cytotoxic Vγ9Vδ2 T cell adoptive immunotherapy has been shown to be effective. Vγ9Vδ2 T cells can infiltrate from the bloodstream to the exact tumor site in both the early and late stages of cancer development. The recruited γδ T cells may gradually extend from tumor suppression to tumor promotion.
To date, specific chemokines in the environment and chemokine receptors on the surface of γδ T cells have not been clearly identified. Most tumor cells can produce CC chemokines (β-chemokines) and CXC chemokines (α-chemokines). Glatzel et al. [54] showed using in vitro experiments that C-C motif chemokine ligand 2 [CCL2; macrophage chemoattractant protein-1 (MCP-1)], CCL3 [macrophage inflammatory protein 1-alpha (MIP-1-α)], CCL4 [macrophage inflammatory protein-1-beta (MIP-1-β)], CCL5 [regulated upon activation normal T cell expressed and presumably secreted (RANTES)], CXCL9 [monokine induced by gamma interferon (MIG)], CXCL10 [interferon gamma-induced protein 10 (IP-10)], CXCL11 [interferon-inducible T-cell alpha chemoattractant (ITAC)], and CXCL12 (SDF1) can attract human γδ T cells for directional migration [54]. Specifically, CCL5 and CXCL10 act as key mediators of cancer signal transduction, producing many pro-inflammatory cytokines related to signal transducer and activator of transcription 3 (STAT3). The CCR2/CCL2 association greatly boosts the migration of γδ T cells in B16 melanoma [55]. Tumor-associated fibroblasts release generous amounts of CXCL12, which stimulates the infiltration of Vγ9Vδ2 T cells in kidney cancer. In addition, CXCL10 (also called interferon gamma-induced protein 10) secreted by breast cancer cells can attract regulatory γδ T cells and exert a negative effect.
Compared with αβ T cells, the movement of γδ T cells is considerably restricted. These cells constitutively express a series of chemokine receptors. Vδ1 T cells preferentially upregulate the inflammatory homing chemokine receptors CCR5 (CD195) and CCR7 (CD197) for recruitment from peripheral blood to the tumor locus, which is typical steady-state receptors. Vγ9Vδ2 T cells stimulated by pAgs show transient expression of CXCR4 [56]. Additionally, CCR2 (CD192) and CCR6 (CD196) mostly facilitate γδT17 cell infiltration into inflammatory tissues [57].
Moreover, adhesion molecules such as lymphocyte function-related antigen 1 (LFA-1), L-selectin, and CD44, are also involved in driving Vγ9Vδ2 T cells to the tumor site. Nevertheless, the reality may be contradictory. The chemokine pattern derived from the tumor stroma tends to recruit suppressive cells, such as Tregs, tolerable DCs, and M2 macrophages, to promote autoimmune escape. Wu et al. [58] used intravital microscopy to observe the endothelial adhesion interaction of γδ T cells and found that they are restricted to tumor microvessels. Hayes and Seigel [59] isolated endothelial cells from human tumor tissues and analyzed their surface intercellular adhesion molecule-1 (ICAM-1) and vascular cell adhesion molecule-1 (VCAM-1). The results revealed a significant decrease in the expression levels of these molecules. The authors explained this finding as probably due to the overexpression of endothelin receptor type B signaling in tumor endothelial cells, which downregulates the expression of ICAM-1 and forms a barrier to prevent T cell penetration. The above environment clearly limits the infiltration of effector γδ T cells.
One of the limitations of γδ T cell immunotherapy is the relatively low abundance in the TME. Therefore, appropriate and careful selection of chemokines clinically seems to drive the effective expansion of γδ T cells. The infusion of specific chemokines into tumors has been shown to be positively correlated with a good prognosis while promoting lymphocyte recruitment and reversing the inhibitory microenvironment.
Antigen-presenting γδ T cells
The functional plasticity of γδ T cells is also manifested in their indirect antitumor effects. Human γδ T cells also show superior antigen presentation ability and were first discovered in tonsillar γδ T cells [60]. γδ T-APC cells can enhance local antitumor adaptive immunity and act as intermediates that bridge innate and adaptive immunity. They have recently attracted much attention.
Flow cytometry has distinguished two populations of tonsillar γδ T cells, namely, resting and preactivated MHC II γδ T cell subpopulations [61]. The former lacks classical APC surface markers, while the latter activated by pAgs displays antigen presentation-related molecules within a short period of time (reportedly in 18 h) [62]. This display may include costimulatory molecules CD80/CD86, MHC-I, and MHC- II molecules, chemokine receptor CCR7, costimulatory receptor CD40, and integrin ligand CD54, which are basically the same as DCs. The final effect is to synergistically initiate CD4+ and CD8+ T cell adaptive immunity.
Comparing the ability of γδ T cells and DCs to present antigens, one analysis demonstrated that peripheral blood Vγ9Vδ2 T cells respond much earlier to the tumor and infection signals. First, γδ T-APCs use insulin-regulated aminopeptidase (IRAP) to transport antigens and become activated [63]. Then, they take up soluble protein antigens into the cytoplasm. Through internal proteasome degradation and endosomal acidification, γδ T-APCs effectively induce CD4+ T cell proliferation. This process is delayed in DCs. Second, activated Vγ9Vδ2 T cells can process exogenous antigens with MHC class-I and present antigen to CD8+ T cells, which are more inducible and exert more intense effects than DCs. Cross-presentation is a key feature of professional APCs, which triggers activated cytotoxic CD8+ T cells against infected peripheral tissue cells.
The above results indicate that γδ T cells are more powerful antigen-processing cells than mature DCs (mDCs). Highlighting the exceptional characteristics of γδT-APCs is helpful for designing new immunotherapeutic strategies.
Immune memory effect of γδ T cells
Recently, a new concept has emerged: infection or immune-related memory γδ T cells in humans and nonhuman primates. This concept is regarded as a γδ T-subpopulation that is re-emphasized and evaluated. γδ T cells have been confirmed to have characteristics of adaptive immunity, similar to conventional αβ T cells, which can have long-term memory protective effects.
Memory γδ T cells have been fully verified in mouse models. In the mesenteric lymph nodes of mice infected with Listeria, Vγ4+ and Vγ6+ γδT17 cells can rapidly proliferate in response to that pathogen in a TCR-dependent manner [64]. Similar memory-like γδT17 cell responses have also been observed in mouse models of psoriasis dermatitis and Staphylococcus aureus infection [65]. Of course, this phenomenon may be due to an increased frequency of γδT17 cells, resulting in activity accumulation. Comparably, NK cells, bone marrow cells, and epithelial stem cells also have memory-like responses. Epigenetic changes promote stronger activation when these cells are re-exposed to inflammatory stimuli.
In humans, our understanding of memory γδ T cells is still unclear. Whether they are tissue-resident or long-term effectors is unknown. Limited evidence has been provided by the addition of Mycobacterium tuberculosis antigens to in vitro culture to simulate an active tuberculosis environment. γδ T cells on infected patients produce two times higher amounts of IFN-γ than those of healthy people [66]. Another example was provided by analysis of the γδ TCR sequence of rhesus monkeys. The results showed that Vγ9Vδ2 T cells vaccinated with the second Bacillus Calmette-Guérin (BCG) vaccine had 9 times more clonal expansion. Compared with cells in the first vaccination, they exerted immunological memory effects both in blood and mucous membranes. This field requires further research to resolve doubts about memory-like γδ T cells.
γδ T cells in cancer immune surveillance act as effector cells
γδ T cells that migrate into the TME have potent and HLA-unlimited cytotoxicity and can perform immune surveillance of tumors. In one study, a large metagenomic dataset was used to perform bioinformatics analysis of the biopsy samples of 39 cancer patients (including solid tumors, leukemia, and lymphoma) [67]. The results indicated that the relative abundance of γδ T cells is a favorable prognostic feature of cancer, although the numbers are not large. However, this conclusion was subsequently questioned because it was impossible to specifically distinguish the molecular characteristics of immune cells, such as γδ T cells, CD4+ T cells, CD8+ T cells, or NK cells. However, γδ T cells exert an antitumor effect to a large extent and have the potential to mobilize immunotherapy.
The latest developments in ribonucleic acid sequencing (RNA-seq), microarray expression databases, Immune Cell Abundance Identifier (ImmuCellAI), and deconvolution methods such as cell type identification by estimating relative subsets of RNA transcripts (CIBERSORT), combined with flow cytometry, will allow the accurate monitoring of lymphocytes in tumor samples and prediction of patient responses to immunotherapy. The isolation of tumor-infiltrating γδ T cells and detection of their immunohistological phenotypes are also good research methods. These techniques are more realistic and intuitive for tumors or adjacent tissues. γδ TILs have been detected in prostate cancer, pancreatic cancer, metastatic renal cancer, hepatocellular carcinoma, glioblastoma multiforme, colorectal cancer (CRC), and breast cancer, among others [68–71]. For example, algorithms have shown that in patients with melanoma, more γδ T cell infiltration combined with PD-1 inhibitor treatment provides a significantly better clinical response [72].
As a special lymphocyte lineage, the antitumor mechanisms of γδ T cells are as follows.
First, Vγ9Vδ2 T cells and Vδ1 T cells recognize TAAs through a variety of surface receptors. Due to the existence of NKG2D, their advantages compared with NK and CD8+ cytotoxic T cells are further magnified. γδ T cells can directly exocytose perforin and granzyme B in the shaped immune synaptic space to kill tumor cells. Indirectly, they activate caspases mediating tumor cell DNA damage.
Second, γδ T cells can induce tumor cell death through antibody (Ab)-dependent cell-mediated cytotoxicity (ADCC). Studies have shown that the addition of pAgs plus IL-2 to the in vitro culture system of Vγ9Vδ2 T cells can upregulate the expression of Fcγ receptor III (FcγRIII; CD16), similar to NK cells, as a transmembrane receptor [73, 74]. In patients with chronic lymphocytic leukemia, breast cancer, or neuroblastoma, when Vγ9Vδ2 T cells were treated with therapeutic monoclonal antibodies in combination, the Fab fragments of the Ab bound to the infected cells or tumor antigen epitope, and the Fc fragments then homeopathically activated γδ T cells. These include the B lymphocyte antigen CD20-specific monoclonal Ab rituximab/obinutuzumab, human epidermal growth factor receptor 2 (HER2) monoclonal Ab trastuzumab, and CD52 monoclonal Ab alemtuzumab, all of which can significantly enhance γδ T cell lysis activity against target cells [75]. Preferably, there is a tribody [(HER2)2xCD16] that comprises two HER2-specific single chain fragments and targets CD16-expressing γδ T cells and NK cells [76]. The enhanced cytotoxicity may benefit from the redirection and strengthened degranulation reaction, overcoming some of the limitations of trastuzumab.
Third, γδ T cells induce target cell apoptosis via the CD95/CD95L (Fas/FasL pathway) and TNF-related apoptosis-inducing ligand [TRAIL/TRAIL receptor (TRAIL-R)] pathways. Specifically, FasL+ γδ T cells bind to the γδ Fas receptor on the surface of osteosarcoma cells to form Fas trimers, resulting in the binding of death effector domain (DED) and Fas-related death domain-containing protein (FADD), ultimately activating caspases to induce osteosarcoma cell apoptosis. The TRAIL pathway also requires the participation of downstream caspases, which has been confirmed in chronic myelogenous leukemia and lung cancer in vitro [77].
Finally, activated γδ T cells secrete IFN-γ or TNF-α, which are the main mediators of effector functions, acting on transformed host cells or pathogens. Autocrine IFN-γ leads to higher expression of MHC-I molecules, which then positively regulate CD8+ cytotoxic T cells and induce an antitumor response. Moreover, IFN-γ can further upregulate Fas expression on the surface of osteosarcoma cells, forming a long-lasting positive feedback activation loop (Figure 2).
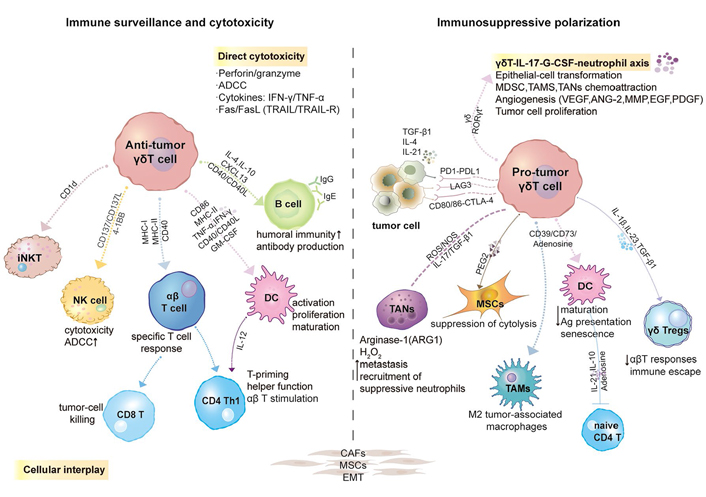
Plasticity, polarization, or reprogramming of γδ T cells in the TME. Induced by a series of chemokines, γδ T cells can be recruited and preferentially migrate into the TME. Initially, γδ T cells act as effector cells in cancer immune surveillance. However, under various regulatory factors such as heterogeneous cytokines, checkpoint molecules, epigenetic regulation, CD39/CD73/Adenosine (ADO) metabolic pathway, etc., the tumor-infiltrating γδ T cells are gradually polarized into tumor-promoting phenotype. During this period, intratumoral γδT cells interacted wonderfully with numerous cells. GM-CSF, granulocyte-macrophage colony- stimulating factor; TGF-β1, transforming growth factor β1; RORγt, retinoic-acid-receptor-related orphan receptor-γt; iNKT: invariant NKT; G-CSF: granulocyte-colony stimulating factor; MDSC: myeloid-derived suppressor cell; TAMs: tumor-associated macrophages; TANs: tumor-associated neutrophils; VEGF: vascular endothelial growth factor; ANG-2: angiopoietin-2; EGF: epidermal growth factor; PDGF: platelet-derived growth factor; LAG-3: lymphocyte activation 3 gene; CTLA-4: cytotoxic T-lymphocyte-associated protein 4; ROS: reactive oxygen species; NOS: nitric oxide synthase; MSCs: mesenchymal stem cells; H2O2: hydrogen peroxide
It is worth examining whether γδ TILs can coordinate the interaction of various immune cells. They form a cell network with DCs, NK cells, macrophages, neutrophils, MSCs, MDSCs, and B cells, among others. This phenomenon will be discussed separately in a subsequent section. In short, γδ T cells play an important role in tumor immune monitoring by their rapid recruitment and HLA-independent killing properties.
Tumor-infiltrating γδ T cell polarization into a tumor-promoting phenotype
The functional plasticity of tumor-infiltrating γδ T cells involves the following three aspects: heterogeneous cytokines, the influence of signaling pathways, and cellular cross-talk. Long-term stimulation by a specific TME seems to trigger γδ T cell immune failure.
Regulation by heterogeneous cytokines
IL-17: a double-edged sword
It is believed that chronic inflammatory cells infiltrate into tumor tissues, and long-term release of abundant inflammatory factors may be one of the causes of carcinogenesis. Among them, IL-17 is an important proinflammatory cytokine that is mainly produced by Th17 cells. IL-17 is involved in the innate immune response, various cancers, infectious diseases, and autoimmune diseases. In this section, we mainly discuss Th17-like γδ T cells and the double-edged effect of IL-17 [78].
As the chief source of IL-17 in the TME, IL-1β, IL-6, and IL-23 induce IL-17 differentiation, which is triggered by tumor-activated monocytes and enhanced by prostaglandin E2 (PGE2). Additional contributors are γδ T cells, cytotoxic T cells, DCs, NKT cells, NK cells and neutrophils, MDSCs, and TAMs. IL-17 secreted by TANs is dependent on IL-6 and IL-23 and is also regulated by IL-1β or TGF-β1. Uncommon cellular contributors are lymphoid tissue inducer (LTi) cells, which are stimulated by IL-23 to secrete IL-17 downstream. For our key point, γδT17 cells in the TME may be polarized in situ or recruited by cytokines. IL-1 and IL-23 are necessary for the polarization of human γδ T cells into Th17-like cells. TGF-β1 is responsible for their development and maintenance, and other cytokines, such as IL-6, mainly play an activating role. Compared with adults, IL-6 is more significant in maintaining the differentiation orientation of human cord blood Vγ9Vδ2 T cells stimulated by HMB-PP. In addition, IL-7 is a stimulant that strongly promotes the expansion of γδT17 cells in vitro. As a result, naive Vγ9Vδ2 T cells gradually differentiate into the γδT17 or Th1/Th17 phenotype, producing IL-17 and IFN-γ [79].
The paradoxical effects of IL-17 are mainly manifested in the following aspects. γδT17 cells are protagonists involved in the establishment of an immunosuppressive TME. Studies have shown that γδ T cells secreting IL-17 accumulate and infiltrate regions of meningitis, gallbladder cancer (GBC), breast cancer, colon cancer, and fibrosarcoma [80]. The patient peripheral blood is dominated by TEM+ Vδ1 T cell types. Simultaneously, higher levels of IL-17 and lower levels of granzyme B, perforin, IFN-γ, FasL, TRAIL, and NKR are detected, while healthy volunteers show TCM+ Vδ1 T cells. Emerging evidence suggests that IL-17 can recruit MDSCs to inhibit the activation of NK cells and CTLs, polarize neutrophils, inhibit tumor cell apoptosis, and weaken the antitumor response in a chemokine-dependent manner.
The classic paradigm used to study γδT17 cells in human solid tumors is CRC, in which up to 83% of IL-17 sources are Vδ1 T cells. Abnormal development of the colorectal intestinal epithelial barrier first appears in CRC, and then the influx of large quantities of microbial products initiates local acute inflammation. At this time, activated DCs secrete the key pro-inflammatory cytokine IL-23, which provides a suitable environment for the polarization of Vδ1 intraepithelial lymphocytes (IELs) to γδT17 cells. McGeachy et al. [80] also recently demonstrated that γδ T cells activated by IL-23 can cause excessive IL-17 release, which plays a pivotal role in secondary brain injury after intracerebral hemorrhage. Simultaneously, γδT17 autocrine IL-8, GM-CSF, and TNF-α, which act to recruit immunosuppressive polymorphonuclear (PMN)-MDSCs, introduce many immunosuppressive granulocytes for involvement in tumor progression. The number of IL-17+ Vδ1 TILs in CRC patients is related to a high recurrence rate, distant metastasis, and a poor survival rate [81].
The γδ T cell-IL-17-G-CSF-neutrophil axis also reveals important significance in squamous cell carcinoma (SCC) patients [82]. In the early stage, intratumoral γδ T cells tend to produce IFN-γ, while in the advanced stage, they tend to produce IL-17. This phenomenon reflects a positive correlation between the γδT17 frequency and tumor-node-metastasis (TNM) staging (tumor volume, metastasis, immune cell infiltration). Regarding the specific mechanism, some reports indicate that IL-17 promotes tumor cell proliferation by activating downstream IL-6/STAT3 and NF-κB. Moreover, studies involving GBC patients have shown that γδT17 cells can induce GBC cells to produce VEGF, ANG-2, and MMP, all of which are γδ T-cell-derived growth factors that jointly promote angiogenesis, enrich the tumor vascular supply, and further induce the formation of metastatic tumors [83]. Other growth factors include EGF, keratinocyte growth factor (KGF), PDGF, IGF-1, and angiopoietin, which increase the cell reproduction rate, leading to hyperplasia in stromal and epithelial compartments, representing the initial sign of cancer development.
In contrast, IL-17 can serve as a tumor suppressor in patients with ovarian cancer, gastric cancer, cervical adenocarcinoma, and esophageal SCC (ESCC). Relatively high expression of IL-17 is correlated with good clinical efficacy. This feature is well reflected in ESCC patients. IL-17 recruits CTLs, the killing activity of which is further enhanced by IL-12 produced by macrophages. In lung cancer, IL-17 induces the expression of the proinflammatory mediators IL-1β, IL-6, TNF-α, and PGE2 and polarizes tumor-related N2 neutrophils into antitumor N1 neutrophils. It is necessary to mention that IL-17 can increase CXCL9, CXCL10, and CCL20 levels on tumor cells, which generally elicit their chemotactic functions by interacting with CXCR3 [84]. CXCL9/CXCR3 stimulates CTLs and NK cells through Th1 polarization and activation, producing IFN-γ, TNF-α, and IL-2 to strengthen tumor immunization.
The almost opposite effects of γδT17 cells may influence the differential medical treatment of tumor patients, leading to disparate abundances of γδ T cells in the TME and corresponding IL-17 expression levels (a diagram is displayed in Figure 2).
TGF-β1
TGF-β is a highly pleiotropic cytokine that regulates cell growth, differentiation, and immune function [85]. At present, TGF-β1 has been studied most thoroughly among members of the human TGF-β superfamily. Based on different tumor types and stages, TGF-β1 plays a dualistic and contradictory role. However, during the malignant progression of tumors, it is generally accepted that TGF-β1 changes from suppressing to promoting cancer (Figure 2).
In the human body, TGF-β1 monomer and latent TGF-β-binding protein (LTBP) are noncovalently bonded to form a precursor peptide complex, which is fixed in the ECM and inactive. Alternatively, TGF-β1 can be covalently attached to glycoprotein A repetitive predominant (GARP) or leucine-rich repetitive protein 33 (LRRC33). Active TGF-β1 can be released from the latent complex only after integrin undergoes acidification stimulation or thrombin hydrolysis. Mature-free TGF-β1 forms homodimers or heterodimers stably by intra/interchain disulfide bonds and acts on its target cells by binding to TGF-β1 receptors [86].
TGF-β1 is enriched in the inhibitory TME, which induces the differentiation of naive CD4+ T cells into Tregs or Th-17 cells, interfering with the proliferation and effector functions of lymphocytes. In vitro experiments have also confirmed that TGF-β1 can selectively expand inhibitory Vγ9Vδ2 T cells with stable forkhead box P3 (FOXP3) expression for 10 days. Consistent results are found for Vδ1 T cells [87]. This is a common experimental method for obtaining inhibitory γδ T cells in vitro. The level of FOXP3 mRNA in γδ Tregs can be boosted by the methyltransferase inhibitor 5-aza-2’-deoxycytidine (Aza) or decitabine. Additionally, our teammates found that FOXP3+ Vγ9Vδ2 T cells can suppress PBMC proliferation in vitro stimulated by an anti-TCR monoclonal Ab, particularly affecting CD4+ T cell proliferation and the production of IFN-γ and TNF-α [88]. However, without pAgs, Vδ2 T cells activated by CD3/CD28 soluble antibodies can only promote transient expression of FOXP3 and do not exert a regulatory effect.
Recent studies of vitamin C (VC) treatment during γδ T cell development has been attractive. The team of Kouakanou et al. [89] in 2018 used VC (L-ascorbic acid) and its less toxic, more stable derivative L-ascorbic acid 2-phosphate (pVC), optimizing the culture conditions of γδ T cells to achieve maximum proliferation and functional activity [89]. The results confirmed that VCs could promote the proliferation of only purified γδ T cells by reducing apoptosis and enhancing cell cycle progression in the G2/M phase. There was no correlation with the inhibition of pAg-restimulated induced cell death [bromohydrin pyrophosphate (BrHPP) triggered activation-induced cell death (AICD)] [90]. VC- and pVC-pretreated Vγ9Vδ2T cells produce more IFN-γ, which can obviously support antitumor immunity. These pretreated cells also showed a moderate increase in oxidative respiration but a strong increase in glycolysis. In 2020, the researchers also pointed out that pVC potently aggravates the suppressive activity of TGF-β-stimulated Vγ9Vδ2T cells and stabilizes FOXP3 expression through differential methylation of the FOXP3 gene and other regulatory cell lineage genes. In another scenario, high levels of TGF-β1 and ADO were found in the lysates of human colorectal tumor tissues, which were infiltrated with CD39+ and FOXP3+ γδ T cells. Anti-TGF-β1 antibodies could partially reverse this inhibitory effect. The CD39/CD73/ADO pathway will be explained below.
Regulation by other cytokines: IL-4
IL-4 is a cytokine that acts to induce the differentiation of naive Th0 cells into Th2 cells and upregulate the expression of MHC class-II [91]. Simultaneously, Th2 cells activated by IL-4 produce extra IL-4, forming a positive loop. IL-4 also promotes the proliferation and differentiation of activated B cells into plasma cells and induces B-cell IgE Ab class switching, working similarly to IL-13 (Figure 2).
In the TME, IL-4 seemingly has dual effects. The first is its ability to promote tumors by enhancing tumor cell resistance to apoptosis. In cancers with high γδ T cell infiltration, including breast cancer, renal cell carcinoma, glioblastoma, prostate cancer, and lung cancer, overexpression of IL-4 receptor (IL-4R), excessive enrichment of IL-4, and an extreme Th2 orientation have been found [92]. Fibroblast growth factor receptor 1 (FGFR-1), transferrin receptor (TfR), and EGF receptor (EGFR) may also be found on the tumor cell surface, which directly functions in promoting tumors. IL-4 inhibits NKG2D expression on Vδ1 T cells, which is not conducive to the identification of acute ligands in the TME and damages the γδ T cell immune response. In addition, IL-4 is related to the differentiation of M2 macrophages with reduced IFN-γ production.
In contrast, IL-4 may contribute to the polarization of the thymus and peripheral γδ T cells toward the Vδ1 subpopulation. The enriched concentration of IL-4 in the serum of patients with lymphoma corresponds to an increase in circulating Vδ1 T cells and a better prognosis. The research of our team member Mao et al. [93] showed that IL-4 inhibits activation of γδ T cells in a STAT6-dependent manner in the presence of γδ TCR signals. This effect is IL-4 dose-dependent. Specifically, IL-4 triggers the phosphorylation of STAT5 and STAT6 and inhibits the phosphorylation of the key signaling molecules SH2 domain containing leukocyte protein of 76kDa (SLP-76), and ERK1/2. IL-4 also acts to inhibit Ca2+ release. However, for activated γδ T cells, IL-4 promotes the proliferation of Vδ1 T cells, significantly increasing the number and proportion of Vδ1 T cells. Alternately, IL-4 indirectly inhibits the growth of Vδ2 T cells through IL-10 secretion. By comparison of Vδ1 T cells and Vδ2 T cells in terms of antitumor aspects, we found that Vδ2 T cells secrete more IFN-γ (3 ng/mL) and TNF-α (600 pg/mL), while Vδ1 T cells secrete more IL-10 (400 pg/mL) and a small amount of IFN-γ (70 pg/mL). Therefore, IL-4 is probably not strongly correlated with stronger killing activity.
IL-21
In normal human tissues, IL-21 is exclusively expressed on the surface of activated CD4+ T cells [94]. Elsewhere, Hodgkin’s lymphoma (HL) cancer cells can produce IL-21. IL-21 has been noted to be beneficial to Vγ9Vδ2 T cell long-lasting tumor immunity. Human Vγ9Vδ2 T cells amplified by IL-21 in vitro express more perforin, granzyme B, IFN-γ, and CXCR3 mRNA, which enhances their effector function. These features are similar to the effect of IL-21 on NK and CD8+ T cells. Clinically, IL-21 has been approved for phase 1 clinical trials in patients with metastatic melanoma and phase 2 clinical trials in combination with rituximab. The reported side effects, such as influenza-like symptoms, are generally considered safe.
However, Barjon et al. [95] discovered that IL-21 has an impact on regulating the differentiation of γδ T cells, polarizing Vγ9Vδ2 T cells and Vδ1 T cells toward an inhibitory phenotype. CD73 expression is increased on the cell surface after exposure to IL-21. CD73+ γδ Tregs can suppress immune responses in a manner dependent on the ADO-IL-10-CXCL8 signaling axis [96].
Vγ9Vδ2 T cells cocultured with HMB-PP and IL-21 selectively express B-cell lymphoma 6 protein (BCL-6), the master regulator of follicular B helper T (Tfh) differentiation, thus exhibiting Tfh-like characteristics, such as PD-1, signaling lymphocytic activation molecule (SLAM)-associated protein (SAP), B and T-lymphocyte attenuator (BTLA), and CD57. Activated Tfh-like γδ T cells secrete IL-2 and IL-4 and low levels of IL-10 and CXCL13. They work together to support B cells in synthesizing high-affinity Igs. They show 15 times more IgG, 10 times more IgA, and 5 times more IgM production, which may be associated with CXCR5+ cells recruited by IL-21 promoting cell migration to B-cell follicles [96]. The plasticity of Vγ9Vδ2 T cell responses is much greater than previously thought.
IL-15
Recently, the multidirectional cytokine IL-15 has gradually gained attention and has been described as the optimal cytokine to effectively improve human γδ T cell adoptive immunotherapy [97]. IL-15 plays a downstream role through the common gamma chain (γ-C, CD132) and IL-2/IL-15 receptor β chain (CD122) complex. Stimulations with TLRs, IFN-γ, lipopolysaccharides, double-stranded (ds) mRNA, GM-CSF, unmethylated cytosine triphosphate deoxynucleotide (“C”)-phosphodiester-guanine triphosphate deoxynucleotide (“G”; CpG) oligonucleotides, and bacterial or viral infections can all facilitate IL-15 expression.
IL-15 usually has advantages and disadvantages together with IL-2. IL-15 is characterized by an inability to induce AICD and upregulation of antiapoptotic factors to support immune cell survival. Using tumor-infiltrating and in vitro-cultured γδ T lymphocytes, the present research showed that IL-2 and IL-15 guide the differentiation of γδT cells into two subgroups. IL-15 tends to polarize CD122+ Th1 γδ T while IL-2 tends to polarize CD25+ Th17 γδ T cells [98]. This phenomenon allows IL-15 to prevent the production of immunosuppressive γδ Tregs.
The omnipotence of IL-15 is that it can basically utilize all classic γδ T cell killing pathways, thus working as a high-quality immune system stimulant. For example, combined use of IL-15 and BrHPP can recruit more γδ TILs in patients with renal cell carcinoma and rectal and colon cancer [99]. They showed stronger antitumor activity associated with higher CD56 and abundant cytotoxic mediators. In addition, more CD16, a surface molecule involved in γδ T cell opsonization and ADCC, is transferred.
There are currently two IL-15 superagonist complexes: IL-15SA and ALT-803 [100, 101]. The latter can stimulate the expansion of cytotoxic cells, such as NK cells, CD8+ T cells, and γδ T cells, and it is being examined in phase III trials of bladder cancer.
IL-7
Next, we discuss the relationship between IL-7 and γδ T cells. IL-7 may act as a regulator of intestinal mucosal lymphocytes and is locally produced by stromal cells, such as intestinal epithelial and goblet cells [102]. The IL-7 receptor is composed of the IL-7Rα subunit and another γc chain. The latter is common to IL-2, IL-4, IL-9, IL-15, and IL-21.
Researchers have found that approximately 90% of γδT17 cells produce IL-17 when stimulated with specific antigens or IL-1 and IL-23. This group of cells expresses IL-7R on their surface and more easily transform into memory-like γδ T cells [103]. IL-7 is generally recognized to exert prominent functions in inducing immune memory and maintaining the balance of T and B cells. In IL-7 or IL-7Rα knockout mice, mature γδ T cells are completely absent, with T cells, B cells, and NK cells subject to developmental restrictions [104]. Similarly, after adding IL-7 to the human umbilical cord blood in an in vitro expansion system, IL-17-producing γδ T cells are specifically expanded, while IFN-γ+ γδ T cells are sacrificed. The application of an IL-7R Ab completely blocks the proliferation of IL-17+ γδ T cells.
The standard method for in vivo and in vitro expansion of human γδ T cells is the usage of IL-2. IL-7 and IL-15 seem to favor the antitumor activity of γδ T cells. Recombinant IL-7 has been introduced in clinical trials to increase the number of circulating CD4+ and CD8+ T cells. In cases of allogeneic γδ T cell transplantation, combination therapy with IL-7 and anti-γδ TCR antibodies may help support the survival of γδ T cells and improve immune recovery [105].
In summary, γδ T cells can release various pro-inflammatory and anti-inflammatory cytokines, which further aggravates the intricate roles of γδ T cells in cancer and the disparate effectiveness of therapies. Therefore, we must thoroughly investigate the γδ T-cell cytokine profile, minimize harmful factors and enable patients to obtain the best-adapted therapy.
Other potential inhibitory mechanisms
Modulation by checkpoint molecules
In the field of cancer immunotherapy, γδ T cells are widely used owing to their unrestricted MHC antigen recognition and strong secretion of cytokines. Their pharmacodynamic activation, partial effectiveness, and safety of phosphorylated antigens have been confirmed in patients. Their further development is restricted because the average response rate and average clinical benefit rate have failed to reach expectations, with values of 21% and 57%, respectively. The host immune status before γδ T cell adoptive immunotherapy may be a point worth exploring. The host immune status includes three effective aspects: immune checkpoints, cell composition, and inhibitory cytokines [106]. Immune checkpoints greatly impacting γδ T cells include PD-1, mucin domain-containing molecule 3 (TIM-3), LAG-3, CTLA-4, T cell immunoreceptor with Ig and ITIM domain (TIGIT), and co-stimulatory adhesion molecule DNAM-1. We will focus on the first three molecules (Figure 2).
Activated γδ T cells and PD-1: Our team usually uses IL-2 and nitrogen-containing bisphosphonate (such as zoledronate) systems to expand human peripheral blood γδ T cells in vitro. Analysis of the experimental results has shown that compared with CD8+ T cells and CD4+ T cells, PD-1 expression levels of freshly isolated γδ T cells are very low. PD-1 shows a transient and significant increase in the γδ T cell activation phase, reaches a peak value on day 4, and then decreases and stabilizes to the initial baseline value. In addition, cocultivation with tumor cells also results in an inducible increase in PD-1. This finding suggests that PD-1 is involved in regulating the antitumor immune response of γδ T cells in the TME.
Correspondingly, the latest experimental results from our group showed that adoptive transfer of modified γδ T cells with autocrine PD-1 Ab show stronger inhibition in transplanted ovarian tumor models of immunodeficient mice. PD-1-Ab+ γδT cells prepared in vitro that secrete PD-1 Ab by itself have stronger antitumor activity than natural γδT cells. They have the advantages of early upregulation of CD69, a strong degranulation response, increased secretion of IFN-γ and TNF-α and enhanced perforin-granzyme activity. Remarkably, PD-1-Ab+ γδ T cells can block the PD-1/PD-L1 signaling pathway by continuously releasing PD-1 antibodies to the tumor site. The ultimate meaning of this cell design is to restore the antitumor activity of endogenous T cells, ultimately enhancing host immune conditions. A healthy individual must effectively and actively deal with tumor antigens and switch the inhibitory TME to a normal environment (unpublished results).
Castella et al. [107] revealed the physiological tolerance superiority of PD-1+ Vγ9Vδ2 T cells. At the fetal-maternal interface, neonatal Vδ2 T cells upregulate PD-1 in the early activation stage, and its expression is persistent for at least 28 days. PD-1/PD-L1 signaling controls the high susceptibility of Vδ2 T cells to a variety of pathogens. This is one of the sticking points that prevent excessive inflammation in the placental bed and the maintenance of a smooth pregnancy.
Iwasaki et al. [108] (high PD-L1) lymphoma cells and a γδ T cell (medium PD-1) system to determine the role of the PD-1/PD-L1 system in γδ T cells. As expected, PD-1 transmits coinhibitory signals in γδ T cells, where γδ T cell cytotoxicity and IFN-γ production are reduced, which is consistent with the application of B7 homolog 1 (B7-H1) blockade in advanced cancer patients to induce long-lasting tumor regression and prolong the stable period of the disease.
In some patients with follicular lymphoma (FL), immunostaining, flow cytometry, and in silico analyses have identified abundant γδ TILs, which are mainly located in the follicular space. Their phenotypic expression is PD1+CD16+ Vγ9Vδ2 TCR [109]. Compared with infiltrating CD4+ and CD8+ T cells, the surface PD-1+ ratio of γδ TILs is highest. Correspondingly, γδ T cells develop ADCC against FL cells when combined with anti-CD20 mAb, but the PD-1 axis can impair this effector function. Overexpression of PD-1 is related to the robust immune escape and low cytolytic index of γδ T cells.
Aotsuka et al. [110] demonstrated that in patients with ovarian cancer and endometrial cancer, γδT17 cell counts together with elevated IL-17 are positively correlated with high expression of PD-L1. This process requires IL-6 activation and STAT3 phosphorylation. Upregulation of PD-L1 can cause a high ratio of neutrophils/lymphocytes and a poor prognosis.
Research by Hoeres et al. [111] demonstrated that in primary AML, pembrolizumab is completely irrelevant to the lysis response of activated or expanded γδ T cells but can promote the secretion of the pro-inflammatory factor IFN-γ. Interestingly, zol-sensitized primary AML cells can directly induce PD-1 upregulation on γδ T cells and contain more IFN-γ, helping to effectively improve the clinical response rate and therapeutic effect of leukemia.
There have been similar verifications in other models, such as the PDAC mouse model. Since γδ T cells in PDAC tissue can occupy 75% of CD3+ cells, this model is unique in simulating the development of human PDAC tumors. Tumor cells and infiltrating inflammatory DCs show high expression of PD-L1, maintaining the survival and inhibitory activity of FOXP3+ Tregs. Furthermore, infiltrated γδ T cells can inhibit αβ T cell activation through PD-1/PD-L cell contact [112].
Effect of TIM-3 and LAG-3 on γδ T cells: Recent reports indicate that TIM-3+ γδ T cells appear in the peripheral blood and tumor tissues of patients with colon cancer [113]. T-cell Ig and TIM-3, also known as hepatitis A virus cellular receptor 2 (HAVCR2), is generally expressed on the surface of Th17 cells, Tregs, DCs, monocytes, and NK cells. TIM-3 has four ligands, including galectin-9, phosphatidylserine (PtdSer), high-mobility group protein 1 (HMGB1), and carcinoembryonic antigen-related cell adhesion molecule 1 (CEACAM1). It is generally believed that HAVCR2 is an immune checkpoint that causes an influx of calcium to the intracellular space and induces programmed cell death, apoptosis, or failure of CD8+ T cells.
Li et al. [113] published a study suggesting that TIM-3+ γδ T cells (mainly Vγ9Vδ2 T cells) have reduced antitumor function through the ERK1/2 pathway [114]. This phenomenon is mainly reflected in a decreased synthesis of perforin and granzyme B, thereby significantly inhibiting the killing efficiency of colon cancer cells. TIM-3 may be an important negative regulator of Vγ9Vδ2 T cell activation. In addition, the HAVCR2 pathway can interact with the PD-1 pathway in dysfunctional CD8+ T cells, Tregs, and γδT17 cells, showing a positive correlation with expression.
Human PDAC has been linked to γδ T cells and TIM-3. γδ TILs in PDAC account for 40% of total tumor-infiltrating T cells, and they express high levels of the depletion ligands PD-L1 and galectin 9, which are significantly higher than those in cancer cells. The use of neutralizing mAbs to permanently block PD-L1 or galectin-9 in γδ T cells can allow the recruitment and infiltration of more CD4+ and CD8+ αβ T cells, enhance their immunogenicity, and induce effective tumor protection, demonstrating that TIM-3 may represent a novel therapeutic target to improve Vγ9Vδ2 T cell adoptive immunotherapy with further validation [115].
Another distinctive checkpoint is the LAG-3. Compared with PD-1 and CTLA-4, little is known about the effects of LAG-3 on the function of γδ T cells. It has been reported that LAG-3 expression defines the exhaustion of intratumoral PD-1+ T cells in FL and correlates with poorer outcomes [116]. In nonadenocarcinoma, especially NSCLC patients, isolated mixed TILs exhibit higher expression of LAG-3+, which is also related to a poor prognosis. The main ligand of LAG-3 is MHC class-II, which negatively regulates T cell homeostasis and prevents autoimmunity, having a synergistic effect with PD-1/PD-L1 [117]. Therefore, LAG-3-based bispecific antibodies may provide a promising therapeutic strategy for cancer.
The CD39/CD73/ADO metabolic pathway
ADO is an endogenous ADO nucleoside that is widely present in nature in the form of ADO monophosphate (AMP), diphosphate (ADP), and ATP derivatives and is shaped through continuous dephosphorylation reactions. Nucleoside triphosphate diphosphohydrolase 1 (NTPDase1), also known as CD39, is an extracellular nucleotidase that converts ATP or ADP into AMP. The 5’-nucleotidase (5’-NT), also known as ecto-5’-nucleotidase or CD73, mediates the final dephosphorylation reaction to produce ADO [118].
Under physiological conditions, CD4+ T cells utilize CD73 to mediate immune tolerance to normal tissues and maintain homeostasis, especially the protection of the fetus against maternal rejection during pregnancy [119]. In the TME, the CD39/CD73/ADO pathway is chiefly involved in the establishment of an immunosuppressive environment [120]. Elevated levels of ADO are found in various types of tumors, including glioblastoma, melanoma, esophageal cancer, prostate cancer, and breast cancer.
ADO receptors contain the following four subtypes: A1, A2A, A2B, and A3. All of them are G protein-coupled receptors and possess different abilities to stimulate or inhibit adenylate cyclase (AC) and phospholipase. Specifically, tumor-derived ADO is coupled to Gs through the A2A receptor, which stimulates AC activation and increases cyclic AMP (cAMP), engaged in immune escape and impairment. Cells expressing ADO receptors are T cells (also γδ T cells), NKs, macrophages, and DCs. Therefore, ADO may alter the consequences of tumor cell killing by immune cells.
In human colorectal and breast cancers, the TME seems to be more willing to attract CD39+ γδ T cells than classic Tregs [121]. Enriched CD39+ Vδ1 T cells have been isolated, approximately 20% of which have FOXP3+ and/or CD73+ phenotypes. CD73 has been shown to inhibit antigen-specific activation of CD3+ T cells in an ADO-dependent manner. It can also damage the maturation potential of DCs. Concurrently, the accumulation of ADO further aggravates tumor invasion and metastasis. Likewise, under IL-2 and HMB-PP stimulation in vitro, the CD39+ and/or CD73+ γδ T cell subsets isolated from peripheral blood are also tightly linked to immunological inhibition. The concentrations of ADO, IL-21, and IL-10 detected in this culture environment also increase to some extent. Therefore, it is of great importance to control the balance between the physiological and pathological significance of anti-inflammatory ADO (a diagram is displayed in Figure 2).
Epigenetic regulation and γδ T cell activation
Large amounts of evidence show that epigenetic regulation is also active in γδ T cells. One example is the epigenetic drug histone deacetylase inhibitor valproic acid (VPA) and the DNA demethylating agent decitabine (DAC), both of which affect γδ T cell activity to varying degrees [122]. VPA shows zoledronate-like direct activation characteristics. DAC is a cytosine analog and was originally used as a chemotherapy drug. It can be metabolized in the body to form deoxynucleoside ATPs, which can competitively inhibit the activity of DNA methyltransferase and initiate tumor suppressor genes. Furthermore, DAC can modulate the expression of the NKG2D ligand, making tumor cells more easily recognized by Vδ2 T cells. It is regarded as an antitumoral factor in clinical trials of liver cancer, lung cancer, and ovarian cancer.
Cellular interplay of intratumoral γδ T cells
In this section, we principally describe the interaction network formed by γδ T cells and other immune cells in the TME to fully understand the biological characteristics of γδ T cells. This cross-talk may encompass a mixture of beneficial or harmful effects (a diagram is displayed in Figure 2).
Bidirectional cross-talk between γδ T cells and DCs
There is potent bidirectional cross-talk between γδ T cells and DCs, representing an essential interaction of paracrine and cell contact interactions. This phenomenon reflects an integrated control mechanism of the immune response to tumors and infections. The mutual activation of DCs and γδ T cells confirmed this statement, thereby bridging innate and adaptive immunity. Three types of DCs have been defined in human blood circulation: CD1c+ myeloid DCs, CD141+ myeloid DCs, and CD303+ plasmacytoid DCs, which perform intricate functions, such as chemokine production and cross-presentation of antigen. DCs are derived from hematopoietic bone marrow progenitor cells and initially differentiate into immature DCs (iDCs), with lower T-cell activation potential and higher endocytic activity. iDCs can be activated into mDCs by presentable antigens [123].
First, DCs produce type I interferon and TNF-α, IL-1β, IL-12, and IL-18, which induce the proliferation of γδ T cells and preferably enhance the release of cytotoxic IFN-γ and TNF-α. DCs can simultaneously induce effective activation of both Vγ9Vδ2T cells and traditional CD8+ CTLs [124]. Notably, when zoledronate is used to prestimulate DCs (including iDCs and mDCs) for 24 h, high levels of IPP can be detected in the cocultures. This condition can be biased to induce central memory and affect memory γδ T cell subtypes and to upregulate the lymphocyte activation markers CD25 and CD69, revealing a strong degranulation reaction and antitumor effect. This effect has been applied and confirmed in clinical studies of CRC patients. DCs trigger contact-dependent activation of γδ T cells, which depends on the CD86-CD28 interaction, as well as the expression of lipid A, pAgs, and other activating ligands [125]. Moreover, Cardone et al. [126] research revealed that the number and function of these two populations are significantly affected by HIV-1 infection. HIV-induced DC dysfunction fails to produce IL-12. They undergo reduced functional maturation, which impairs the interplay with γδ T cells, reducing the capacity of DCs to promote functional γδ T cell activation.
Second, the available research suggests that pAg-stimulated Vγ9Vδ2 T lymphocytes can promote DC maturity and trigger an effective T-cell immune response. The combination of cytokines and cell contact-dependent signals is responsible for this interplay, consisting of IFN-γ, TNF-α, CD1, Fas-FasL, and CD40-CD40L. γδ T cells activated by different ligands act on different pathways. The latest research concerns amino bisphosphonate (ABP)-related synthetic compounds, such as PAM, alendronate (ALD), risedronate, ibandronate, and zoledronate, which effectively activate the cytotoxic activity of human γδ T cells [127]. For example, PAM-activated γδ T cells strictly depend on physical contact with DCs, but IPP does not require physical contact. Monocyte-derived iDCs show a significant upregulation of the costimulatory molecules CD80 (B7-1) or CD86 (B7-2), MHC class-I or MHC class-II, CD40, and CD83 during maturation, achieving the classic APC function. This theory was first raised by Ismaili et al. [128].
Third, DCs and γδ T cells influence each other in terms of chemokines and cytokines. To illustrate this phenomenon, Vγ9Vδ2T cells upregulate CCR7 molecules to induce DC homing to various secondary lymphoid organs. Examples include homing to the spleen through the bloodstream or homing to lymph nodes through the lymphatic system. CCR7 is also closely linked to γδ T cell trafficking within the spleen. Alternately, in ovarian cancer or hepatitis B virus (HBV) infection, γδT17 cells may increase the number of tumor-infiltrating DCs through CCL20 and sometimes indicate an improvement in the patients’ overall survival rate. In contrast, some researchers have also indicated that DC-TILs are often accompanied by low expression of CD80/CD86, disrupting antigen presentation [129]. For instance, iDCs derived from the peripheral blood of breast cancer patients can induce T cell anergy and tumor tolerance, thereby promoting immune escape.
At present, the combined treatment of DC vaccines and γδ T cells may cooperate to counteract the immunosuppressive cancer microenvironment. Monitoring the effector characteristics of γδ TILs will be a parameter for evaluating DC vaccines and subsequently assessing the benefit rate of cancer patients [130].
Follicular B helper γδ T cells
The interplay between γδ T cells and B cells is essential in adaptive immunity. Early reports by Rezende et al. [131] and Lee et al. [132] showed that γδ T cells can help B cells produce antibodies and maintain the germinal center (GC). In the presence of IL-21, HMB-PP induces Vγ9Vδ2 T cells to express IL-21R, which is greatly enhanced compared with the resting state. Activated Vγ9Vδ2 T cells polarize to the Tfh-like subtype. Costimulatory molecules, such as CD40L, CD28, and inducible T-cell costimulatory (ICOS) molecules, are upregulated on their surface. Through upstream and downstream interactions of CD40/CD40L, ICOS/ICOS ligand (ICOSL), and CD28/CD86, γδ Tfh cells have mutual contact with B cells and secrete IL-2, IL-4, and IL-10. The above reactions result in differentiation into extrafollicular short-lived plasma cells and promote B-cell migration into follicles, incidentally forming GCs.
γδ Tfh cells express CXCL13, BCL-6, and CXCR5, which support B cells in producing antigen-specific and high-affinity antibodies, as well as switching Ab classes. This situation can occur 2 days after autologous B cells are cocultured with HMB-PP and IL-21. Additionally, unconventional costimulation enhances the production of IgM, IgG, and IgA. Instead, Vδ3 T cells have recently been confirmed to upregulate the expression of CD40, CD86, and human leukocyte class II DR antigens (HLA-DR) and promote the synthesis of specific IgM subtypes by B cells. The Vδ3 T cell subpopulation is completely unrelated to the production of IgG, IgA, or IgE.
By re-examining this relationship, the new discovery that γδ T cells can encourage the body’s production of autoantibodies by B cells is thought-provoking [133]. This process uniquely depends on whether DNA damage has occurred. In a model of skin epithelial injury caused by the carcinogen 7,12-dimethylbenz[a] anthracene (DMBA), Crawford et al. [134] found that γδ TCR+ IELs can drive the synthesis of autoreactive IgE. Another chemical agent 12-O-tetradecanoylphorbol-13-acetate (TPA) model, which is incapable of damaging DNA, does not stimulate endogenous IgE. Autoimmune diseases and the destruction of tolerance are probably involved in this process. The number of γδ T cells is significantly higher in human rheumatoid arthritis and systemic lupus erythematosus (SLE) patients. Autoantibodies such as anti-nuclear antibodies (ANAs), anti-ds-DNA, and small nuclear ribonucleoproteins have also been detected in Vγ4/6-deficient mice, showing a high degree of similarity to human SLE [134]. The author interprets this result as due to the remaining Vδ1 T cell (possibly including αβ T cell) excessive production of IL-4, followed by the acceleration of B cell maturation through an overrun tolerance mechanism.
In return, human γδ T cells isolated from peripheral blood are also affected by B cells. Activated B cells upregulate B7 and CD39 and contribute to the activation of human Vδ1 T cells, which appear to be TCR ligand-dependent.
Likewise, BTNs also affect cross-talk. BTN2A2 is a less-mentioned isomer in the BTN family. It is encoded by the BTN2A2 gene in humans and participates in lipid, fatty acid, and sterol metabolism. BTN2A2 is highly expressed in the brain, bone marrow, small intestine, muscle, spleen, and pancreas. BTN2A2+ splenic B cells may be involved in γδ T cell recognition and homeostasis [131].
Immunosuppressive effect of MSCs on γδ T cells
MSCs are multifunctional stromal cells that can differentiate into multiple cell types. Research on MSCs mainly focuses on tissue engineering and regenerative cell therapy. Moreover, MSCs can impact both innate and acquired immune cells [135]. As mentioned previously, the tumor stroma is rich in MCP-1, SDF1, PDGF, EGF, and VEGF, which tend to migrate into tumors (frequently occurring in glioma, melanoma, breast cancer) and constituting an indispensable component of the TME.
MSCs mediate the formation of a local immunosuppressive TME. They can inhibit the proliferation of γδ T cells, prevent the activation of specific Th1 cells, reduce the expression of NKG2D, NKp44, and NKp30, inhibit the differentiation and maturation of DCs, and counteract the production of antitumor cytokines [136]. The suppressive effect of MSCs toward Vγ9Vδ2 T cells mainly occurs through the interaction of receptor ligands and the production of polymorphic immunomodulatory molecules. The latter include PGE2, indoleamine 2,3-dioxygenase (IDO), nitric oxide (NO), human growth factor (HGF), and inhibitory cytokines such as IL-6, IL-10, and TGF-β1. Moreover, many solid tumor-infiltrating MSCs display positive phenotypes for FasL, PD-L1, and PD-L2. Thus, intratumoral MSCs are more likely to play a regulatory role and escape the cytotoxicity of Vγ9Vδ2 T cells.
In detail, MSCs that secrete a large amount of PGE2 play a pivotal role in this process, which can be partially reversed by the PGE2 inhibitor indomethacin [137]. It is known that pAgs-activated Vγ9Vδ2 T cells secrete IFN-γ and TNF-α. In the context of cyclooxygenase-2 (COX-2), these two biological factors are beneficial for the induction of PGE2 production by MSCs. The PGE2 receptor is a G protein-coupled receptor that includes four protein isoforms of prostaglandin E2 receptor (PTGER1-4). Two receptors, PTGER2 and PTGER4, are expressed on the surface of Vγ9Vδ2 T cells. Intracellular cAMP rises as a result of ligand-receptor binding, which can block early cytotoxicity-related signal transduction. Some reports state that PTGER4 inhibitors can partially suppress angiogenesis and lymphangiogenesis, thereby inhibiting tumor growth and metastasis (Figure 2).
TANs
There are complicated mutual accommodations between γδ T cells and neutrophils, which sometimes show dynamic interplay. Their coordination and balance are crucial for governing the metabolic state of the TME. The γδ T cell-IL-17-G-CSF-neutrophil axis is one interaction mechanism, and IL-17 acts as an emphatic agent to promote this interaction.
First, neutrophils can remodel the orientations and states of γδ T cell proliferation. In human CRC patients, tumor-infiltrating neutrophils produce IL-17 to promote the proliferation of γδT17 cells and support tumor growth and metastasis [138]. IL-17 is an upstream regulator of G-CSF. Neutralization of IL-17 can reduce the concentration of blood G-CSF and downregulate the inhibitory activity of TANs. TANs and Treg cells inhibit the activation of Vδ2 T cells and control the production of IFN-γ by Th1-like γδ T cells, which is predominantly due to the derived IL-10 and TGF-β1 and dependence on tumor promoters, such as arginase-1, a serine protease, metalloprotease, neutrophil elastase (NE) and ROS. Nevertheless, since the antioxidant glutathione in γδT17 cells is usually maintained at a low level, it is particularly sensitive to neutrophil-derived ROS [139]. Therefore, a corresponding treatment plan has been developed for hepatocellular carcinoma and melanoma. That is, inducing a mild oxidative stress environment may prevent γδT17 cells from exerting indirect antitumor functions.
The regulatory γδ T cells then use IL-17 to modify the development of neutrophils, which generally occurs in a highly inflammatory environment (rich in IL-1β and IL-23) caused by cancer cells [140]. For example, IL-17 derived from γδ T cells in breast cancer can act as a proinflammatory cytokine. Relying on GM-CSF and IL-8, it directly recruits MDSCs, polarizes M2 macrophages, and indirectly promotes neutrophil polarization toward an immunosuppressive phenotype. Clinical research has demonstrated that the γδ T cell-neutrophil axis plays a particularly prominent role in lung metastasis of breast cancer and is highlighted only in the early stage. Neutrophils have little effect on tumors that have metastasized.
Finally, we believe that TANs customarily exhibit soft plasticity and differences in phenotype and function. ADCC mediated by neutrophils contributes to the antitumor immune response, most likely in the early period of tumor development [141]. This phenomenon is also reflected in the flexible response of neutrophils exposed to different activators of γδ T cells. Towstyka et al. [142] isolated neutrophils pretreated with HMB-PP, and they found that the cytotoxic mediator IFN-γ was induced in a short period of time, synergistic with the γδ T cell killing ability stimulated by CD3 Ab. However, this only occurred with HMB-PP stimulation, not in N-BPs. Peripheral blood neutrophils undergo endocytosis and absorption of N-BPs, and this reaction does not produce IPP but H2O2 to inhibit γδ T cell activation (Figure 2).
Optimization of γδ T cell-based adoptive immunotherapy
Tumor immunotherapy is currently a hot research topic. γδ T cells have gradually become key research and development targets and are known as “the next great candidate for chimeric antigen receptor (CAR)-modified T (CAR-T) cells” The recognition and killing mode of γδ T cells does not depend on MHC molecules, so most γδ T cells are not limited to the cancer—immune escape mechanism that downregulates MHC class-I on the surface of target cells. More preferably, there is almost no severe complication graft- versus-host disease (GVHD) reaction after γδ T cell transplantation and no cytokine storm or off-target effects, which greatly overcomes the shortcomings of CAR-T technology [143]. Therefore, γδ T cells used as general allogeneic immune cell preparations have very broad prospects.
First and foremost, γδ T cell-based immunotherapy demonstrates safety, effectiveness, and feasibility for patients with advanced tumors or patients who are not sensitive to chemotherapy, which has been confirmed in clinical studies of hematological malignancies (leukemia), head and neck cancer, hepatocellular carcinoma, renal cell carcinoma, lung cancer, prostate cancer, breast cancer, and neuroblastoma.
For example, in a phase I clinical trial involving 132 patients with kidney cancer, leukemia, liver cancer, and lung cancer, a total of 414 allogeneic γδ T cell reinfusion showed that they were tolerable and safe. Only some patients exhibited a transient mild clinical reaction (automatic recovery within 24 h), such as low-grade fever, rash, diarrhea, nausea, vomiting, fatigue, etc. Follow-up visits after treatment until June 2020 revealed a significantly extended average survival time.
In addition, γδ T cells already have a standard large-scale culture mode, with a short supply time and low cost. Currently, the clinical treatment strategy for γδ T cells involves PBMC extraction and in vitro activation of nonpeptide pAgs. BTN3A/CD277-specific Ab 20.1 can enhance reactivity in AML models [144]. Most recently, ImCheck Therapeutics has entered humanized BTN3A agonist (ICT)-01 into phase I trials for patients with hematological malignancies. ICT01 is a Vγ9Vδ2 T cell-activating monoclonal Ab that targets the extracellular domain of BTN3A with good safety [145]. The advantage of the BTN3A Ab is its ability to directly and specifically amplify the potential of Vγ9Vδ2 T cells in finding and destroying patient cancer cells, without the need for later activation and infusion in vitro. Further understanding of the activation mechanism of γδ T cells may provide a powerful research basis for the treatment of various cancers.
Finally, γδ T cells in the TME are highly plastic, which disrupts the effectiveness of immunotherapy to a certain extent. Consequently, the antitumor activity of γδ T cells must be maximized, the in vitro expansion protocol optimized and the tumor-promoting activity minimized to obtain a large number of γδ T cells with high killing activity in a short time.
Therapeutic strategies for the long-term maintenance of γδ T cell effector function
A variety of promising clinical treatment programs for γδ T cells has been developed.
In cancer treatment, targeting bispecific or multispecific γδ T cells have great potential. For example, the Her2/Vγ9 bispecific Ab (BsAb) that binds Vγ9 and Her2/neu [receptor tyrosine-protein kinase erbB-2 (ERBB2)] can selectively recruit γδ T cells to the pancreatic tumor site and reduce the growth of grafted tumors in SCID-Beige immunocompromised mice [75]. Furthermore, the γδ T-NK-cell BsAb significantly enhances the cytotoxic activity of Vδ2/Vδ1 cells and NK cells and does not recruit immunosuppressive γδ T cells.
γδ T cells have many unique advantages compared with other cells. They are superior in terms of conservative monomorphism, selective cell recruitment, and chemotaxis properties. de Bruin et al. [146] used the Vγ9Vδ2 TCR sequence to construct a nanobody-based BsAb that simultaneously targets Vγ9Vδ2 T cells and EGFR, which has been shown to be effective in inducing Vγ9Vδ2 T cell activation. The experimental results of the mouse xenograft model also showed that the tumor tissue lysis triggered by this BsAb is independent of Kirsten rat sarcoma 2 viral oncogene homolog (KRAS) and v-raf murine sarcoma viral oncogene homolog B (BRAF) tumor mutation status. Multispecific antibodies, such as tribody [(HER2)2xCD16], have been introduced previously [75, 147].
γδT cells exhibit reduced alloreactivity and a decrease in ‘on-target off-tumor’ toxicities. Park et al. [148] generated ex vivo armed γδ T cells, named EATs, to specifically amplify their advantages. IgG-[L]-single chain fragment V (scFv)-platformed BsAb was used, where the anti-CD3 (huOKT3) scFv was attached to the light chain of a tumor-binding IgG. The results showed that EATs using γδ T cells were as effective as αβ T cell-EATs. They release fewer TNF-α, accompanied by faster infiltration of tumors. The potency of EATs is dependent on the armed BsAb dose, the number of injected EAT cells, and the strength of the treatment plan.
The use of CARs as carriers for γδ T cells to construct CAR-γδ T cells is also an effective strategy [149]. As of April 2020, CytoMed’s γδ CAR-T cell therapy entered the clinical phase. Other opinions recommend switching to NK cells as carriers, which may reduce the risk of adverse side effects such as cytokine storms. The shortcomings of this therapy are also obvious. First, it relies largely on the mutation burden accumulated by hematological tumors. In addition, the possibility of acquired education or unwanted polarization increases. Both γδ T cells and NK cells are under fine and strict regulation by natural cytotoxic receptors and killer-cell Ig-like receptors (KIRs). Therefore, further investigation of this innovative therapy is required.
Similarly, Adicet Bio is developing a combination therapy based on γδ T cells targeting malignant B cells. This scheme assembles endogenous cytotoxic receptors of γδ T cells and anti-CD20 CAR, showing highly effective antitumor activity in preclinical models [150].
The next generation of engineered γδ T cells, called thermoelectric generators (TEGs), has been employed to target a wide range of solid and hematological tumors. This combination reduces the susceptibility of general γδ T cells to KIR inhibition since a low KIR level exists on the surface of αβT cells. “TEG001” refers to the transduction of high-affinity Vγ9Vδ2 TCR into αβT cells [151]. It has been implemented in phase I clinical trials in patients with MM, refractory AML, and myelodysplastic syndromes.
Utilization of a nanoparticle delivery system in combination with γδ T cell immunotherapy is another emerging technology [152]. Hodgins et al. [153] encapsulated γδ T cell sensitizers (N-BPs, such as zoledronate and ALD) into liposomes to make liposomal ALD (ALN-L). Liposome particles < 200 nm in diameter are most likely to pass through the tumor neovasculature and accumulate in the tumor area, forming an EPR effect. In the athymic nude mouse model of MDA-231 breast cancer cells, compared with direct stimulation with ALD, ALN-L-treated animals show effective tumor growth inhibition without necrosis in the injection area because wrapped ALD activates tumor-resident γδ T cells more intensely, enhancing their killing sensitivity to target tumors. ALN-L strengthens the inhibitory effect of γδ T cells toward the gradual accumulation of solid tumors. The combination of ALN-L and γδ T cells has enlightened the development of clinical biologics.
Conclusions and future perspectives
A fundamental aspect that must be mentioned is the limitations of γδ T cell preclinical research. Vγ9Vδ2 T cells are primarily classified into a unique cell type that only exists in humans and primates [154]. The preclinical models commonly used in experimental research consist of immunodeficient or humanized mice. However, they express neither homologous γδ TCRs nor BTN2A/3A family proteins, making it difficult to completely mimic the human body environment. Similarly, in traditional two-dimensional (2D) monolayer cell cultures, cells can always be maintained artificially in an optimal state for vigorous growth, which evidently differs from the internal environment. Consequently, biological model designs require updating to develop a three-dimensional (3D) cell culture technology that improves the accuracy of the cellular response to endogenous and exogenous stimuli to better mimic the ecology of the human body, such as changes in temperature, pH, nutrient absorption, transport, and differentiation.
The continuous progression of cancer is associated with failed immune surveillance. Whether the microenvironment is conducive to antitumor cells, that is, whether activated γδ T cells can relieve local immunosuppression, will directly affect their effector response. Hence, early assessment of the host’s immune status, detection characteristics of cell metabolism, and in-time monitoring of tumor development are indispensable in γδ T cell immunotherapy to reverse the small number of γδ T cells in peripheral blood after infusion, as well as γδ T cell anergy induced by activation.
Tumor-infiltrated cells often exhibit disordered metabolisms, such as hypoxia, lactic acid accumulation, or glucose consumption. Therefore, they can easily become polarized into undesirable phenotypes. The recent demonstration of distinct metabolic programming in murine γδ T cell subsets may affect their lineages and functions in the TME and their intratumoral activities. Pro-tumoral γδ T cells showed high lipid uptake and intracellular lipid storage. Conversely, glucose supplementation enhanced the antitumor functions of γδ T cells [155]. Not all cancer types are susceptible to γδ T cells. The establishment of a susceptibility database is recommended for γδ T cell therapy by summarizing the commonalities of benign prognoses in clinical treatment. This database would be helpful for choosing whether to accept γδ T cell immunotherapy and extending it into precision immuno-oncology (IO), helping the medical community customize individualized therapeutics. For example, the use of high-throughput sequencing, also known as next-generation sequencing (NGS), can distinguish tumor heterogeneity and phenotypic complexity [156]. When incorporated with epigenetic regulation, bioinformatics analysis, clinical phenotypic analysis, comprehensive multidisciplinary analysis, and interpretation, we will have the ability to choose the most suitable scheme and provide an accurate diagnosis for patients.
Overall, how do we accurately judge the host’s immune status? Traditionally, it is difficult to diagnose early hematological cancer solely by analyzing the number of inflammatory cells or malignant lymphocytes in peripheral blood. Currently, immune repertoire sequencing (IR-Seq) is a more promising criterion [157]. This method is very sensitive and accurate in calculating the mutation load of antigen determinants and assessing immune status. It can also professionally analyze suppressive T cell subsets. IR-Seq is expected to build an efficient and reliable immune map database for healthy people and patients. Promising results have been achieved in the follow-up of early diagnosis, evaluation of treatment effects, and recurrence tracking for leukemia and lymphoma. Likewise, IO biomarker methods are available to analyze tumor mutational burden or IFN features [158]. The complexity of the immune system requires the integration of multiple methods to achieve a standardized predictive value.
The success of γδ T cell adoptive immunotherapy predominantly depends on the balance between effector and regulatory cells. Further studies are needed to systematically reveal the contrasting roles of γδ T cells in various diseases. We hope that burgeoning immune strategies based on γδ T cells can truly benefit patients and provide thriving opportunities for the treatment of tumors.
Abbreviations
Ab: | antibody |
ADCC: | antibody-dependent cell-mediated cytotoxicity |
ADO: | adenosine |
ALD: | alendronate |
ALN-L: | liposomal alendronate |
AML: | acute myeloid leukemia |
AMP: | adenosine monophosphate |
ANG-2: | angiopoietin-2 |
APCs: | antigen-presenting cells |
Apo A-I: | apolipoprotein A-I |
ATP: | adenosine 5’-triphosphate |
BsAb: | bispecific antibody |
BTN: | butyrophilin |
BTN3A: | butyrophilin 3A |
CAFs: | cancer-associated fibroblasts |
CAR: | chimeric antigen receptor |
CAR-T: | chimeric antigen receptor-modified T |
CCR7: | chemokine receptor 7 |
CD: | classification determinants |
CDR 1/2: | complementarity determining region 1/2 |
CRC: | colorectal cancer |
CTLA-4: | cytotoxic T-lymphocyte-associated protein 4 |
CTLs: | cytotoxic T lymphocytes |
CXCL12: | C-X-C motif chemokine ligand 12 |
DAC: | demethylating agent decitabine |
DCs: | dendritic cells |
DNAM-1: | DNAX accessory molecule-1 |
ECM: | extracellular matrix |
EGF: | epidermal growth factor |
ERK: | extracellular signal-regulated kinase |
FasL: | Fas ligand |
FL: | follicular lymphoma |
FOXP3: | forkhead box P3 |
GBC: | gallbladder cancer |
G-CSF: | granulocyte-colony stimulating factor |
GM-CSF: | granulocyte-macrophage colony-stimulating factor |
H2O2: | hydrogen peroxide |
HAVCR2: | hepatitis A virus cellular receptor 2 |
HER2: | human epidermal growth factor receptor 2 |
HIV: | human immunodeficiency virus |
HLA: | human leukocyte antigen |
HMB-PP: | (E)-4-hydroxy-3-methyl-but-2-enyl pyrophosphate |
ICOS: | inducible T-cell costimulatory |
iDCs: | immature dendritic cells |
IFN-γ: | interferon-γ |
IgM: | immunoglobulin M |
IL-2: | interleukin-2 |
IL-4R: | interleukin-4 receptor |
IPP: | isopentenyl pyrophosphate |
LAG-3: | lymphocyte activation 3 gene |
MAPK: | mitogen-activated protein kinase |
mDCs: | mature dendritic cells |
MDSC: | myeloid-derived suppressor cell |
MHC: | major histocompatibility complex |
MSCs: | mesenchymal stem cells |
N-BPs: | aminobisphosphonates |
NCR3: | natural cytotoxicity receptor-3 |
NF-κB: | nuclear factor-kappaB |
NK: | natural killer |
NKG2D: | natural killer group 2D |
NKRs: | natural killer receptors |
pAgs: | phosphoantigens |
PAM: | pamidronate |
PBMCs: | peripheral blood mononuclear cells |
PD-1: | programmed cell death protein 1 |
PDAC: | pancreatic ductal adenocarcinoma |
PDGF: | platelet-derived growth factor |
PD-L1: | programmed death ligand-1 |
PGE2: | prostaglandin E2 |
PTGER: | prostaglandin E2 receptor |
pVC: | L-ascorbic acid 2-phosphate |
ROS: | reactive oxygen species |
SDF1: | stromal cell-derived factor 1 |
STAT3: | signal transducer and activator of transcription 3 |
TAAs: | tumor-associated antigens |
TAMs: | tumor-associated macrophages |
TANs: | tumor-associated neutrophils |
TCR: | T cell receptor |
Tfh: | follicular B helper T |
TGF-β1: | transforming growth factor β1 |
Th1: | T-helper 1 |
TILs: | tumor-infiltrating lymphocytes |
TIM-3: | mucin domain-containing molecule 3 |
TLRs: | Toll-like receptors |
TME: | tumor microenvironment |
TNF-α: | tumor necrosis factor-alpha |
TRAIL: | tumor necrosis factor-related apoptosis-inducing ligand |
ULBP-4: | UL16-binding protein 4 |
V:: | variable |
VC: | vitamin C |
VEGF: | vascular endothelial growth factor |
Vγ: | γ-chain variable region |
Vδ1: | δ-chain variable region 1 |
Declarations
Author contributions
YW wrote the manuscript. YX helped to edit the figure. HC, JZ and WH revised the manuscript. All authors contributed to the article and approved the submitted version.
Conflicts of interest
The authors declare that they have no conflicts of interest.
Ethical approval
Not applicable.
Consent to participate
Not applicable.
Consent to publication
Not applicable.
Availability of data and materials
Not applicable.
Funding
This work was supported by the National Natural Science Foundation of China (81972866, 31970843, 82071791 and U20A20374), Chinese Academy of Medical Sciences Initiative for Innovative Medicine (2021-1-I2M-005 and 2021-1-I2M-035) and Chinese Academy of Medical Sciences Basic Research Expenses (2018PT31052). The funders had no role in study design, data collection and analysis, decision to publish, or preparation of the manuscript.
Copyright
© The Author(s) 2022.