Abstract
The immune system, whose nature lies in being a complex network of interactions, lends itself well to being represented and studied using graph theory. However, it should be noted that although the formalization of models of the immune system is relatively recent, the medical use of its signaling network structure has been carried out empirically for centuries in vaccinology, immunopathology, and clinical immunology, as evidenced by the development of effective vaccines, the management of transplant rejection, the management of allergies, and the treatment of certain types of cancer and autoimmune diseases. A network optimization analogy is proposed through the employment of the system dynamic formalism of causal loop diagrams (CLDs), where current network operations (also known as NetOps) in information technology (IT), are interpreted as immune NetOps in coronavirus disease 2019 (COVID-19) treatment. Traffic shaping corresponds to signaling pathway modulation by immunosuppressors. Data caching corresponds to the activation of innate immunity by application of Bacillus Calmette-Guerin (BCG) and other vaccines. Data compression corresponds with the activation of adaptative immune response by vaccination with the actual approved COVID-19 vaccines. Buffer tuning corresponds with concurrent activation of innate and adaptative or specialized immune cells and antibodies that attack and destroy foreign invaders by trained immunity-based vaccines to develop. The present study delineates some experimental extensions and future developments. Given the complex communication architecture of signal transduction in the immune system, it is apparent that multiple parallel pathways influencing and regulating each other are not the exception but the norm. Thus, the transition from empirical immune NetOps to analytical immune NetOps is a goal for the near future in biomedicine.
Keywords
Systems immunology, immune networks, network operations, cell signaling pathways, COVID-19Introduction
Since the creation of graph theory to solve the riddle of the seven Königsberg bridges, it has become an essential tool for the representation, and subsequent analysis of problems in various areas of basic sciences, engineering, telecommunications, human and social sciences [1], and more recently, in biomedical sciences [2]. Within the latter, applications in neurobiology, genomics, and transcriptomics, including physiological signaling, are fairly well developed and are an integral part of complex biological network analysis applications used throughout the world [3, 4]. However, it should be noted that although the formalization of models of the immune system is relatively recent, the medical use of its signaling network nature has been carried out empirically for centuries in vaccinology, immunopathology, and clinical immunology, as evidenced by the development of effective vaccines, the management of transplant rejection, the management of allergies, the treatment of certain types of cancer and autoimmune diseases, just to mention some of the most well-known applications.
The appearance of severe acute respiratory syndrome coronavirus 2 (SARS-CoV-2) at the end of 2019 and its exponential spread throughout the planet, causing the global pandemic, has led researchers around the world, both from the public and private sectors, universities, and research centers, to resume, adapt, develop and apply, a whole series of immunological regulation treatments to control coronavirus disease 2019 (COVID-19), with the development of messenger RNA (mRNA) technology vaccines being one of the most significant achievements without a doubt, but also resorting to strategies commonly used for the treatment of autoimmune diseases, human immunodeficiency virus (HIV) and cancer among others.
Network optimization analogy
In this article, an analogy is developed, based on the optimization of information technology (IT) networks, which gives rise to proposing an integrating panorama, from health data science, related to the management measures of COVID-19, which corresponds to the equivalent biomedical team of the network operations (NetOps), whose work is essential for the proper functioning and continuous improvement of the communication networks that are the basis of the information society of which we are currently immersed. In IT, NetOps focus on the philosophies, practices, and tools in building and operating networks to deliver and respond to application and service needs; in biomedical sciences, immune NetOps focused on the philosophies, practices, and tools in operating those networks of signaling pathways related with the activation of innate and/or adaptive immune responses and the Janus kinase (JAK)/signal transducer of activation (STAT) signaling networks through the use of immunogens and immunosuppressors [5, 6].
Four of the most used IT NetOps are used, namely, traffic shaping, data caching, data compression, and buffer tuning [7] (Table 1). In the present paper, each of them is defined and the corresponding equivalence, in terms of immune NetOps and its operator, is proposed. Given the nature of the COVID-19 pandemics, several authors have used systems thinking as a framework that can help to develop the most effective strategies to contain the virus keeping the mortality rate low while maintaining economic, social, and environmental goals when managing the outbreak worldwide [8].
The network optimization analogy
IT NetOps | Definition | Immune NetOps | Immune operators |
---|---|---|---|
Traffic shaping | Traffic shaping is a strategy to improve the performance and management of traffic in a network | JAK/STAT signaling pathway modulation | Immunosupressors |
Data caching | Data caching is a process that stores multiple copies of data in a temporary storage location—or cache—so they can be accessed faster | Activation of trained immunity/innate immunity | BCG/influenza/pneumococcal vaccine |
Data compression | Data compression is the process of encoding, restructuring data in order to reduce its size | Activation of adaptative immunity/immune memory | COVID-19 approved vaccines |
Buffer tuning | Buffer tuning or network buffer, corresponds with the smallest unit for effective communication between subtasks | Concurrent activation of innate and adaptative immune responses/immune fitness | New type of vaccines/TIbV |
BCG: bacillus Calmette-Guerin; TIbV: trained immunity-based vaccines
Subsequently, to develop the proposed immune NetOps, we employ the causal loop diagrams (CLDs) formalism [9]. Briefly, the existence of feedback loops within the system indicates two-way relationships between actions and consequences. There are two types of feedback loops: reinforcing (R) and balancing loops (B). Reinforcing loops are responsible for the creation of exponential growth or decay in the system, whereas balancing loops will balance a system until an equilibrium has been achieved. Moreover, visualizing concepts through CLDs makes it easier to understand when we are dealing with complex correlations and casualties.
Analogy interpretation regarding COVID-19
Traffic shaping
Severe COVID-19 disease is closely related with dysregulation of local and systemic immune responses. Thus, since the beginning of the pandemic at the end of 2019, various clinical treatments have been used seeking to i) alleviate the symptoms of patients with severe COVID-19 and ii) save the lives of those patients in critical condition hospitalized in intensive care units, being one of the most successful, the application of immunomodulators such as Baricitinib and Ruxolitinib, which act on the signaling networks associated with the JAK/STAT complex, here considered as a strategy to improve the performance and management of traffic in the JAK/STAT signaling network, and which have proven to be effective in the treatment of cytokine storm syndrome, common in patients with severe COVID-19 [10, 11].
The underlying logic of this clinical strategy can be represented, in a general way (Figure 1), as the action of two associated balanced processes, the first to the control of the state of hyper inflammation due to the cytokine storm syndrome, by the administration of the regulator, and the second, associated with viremia control given the clinical improvement of the patient.
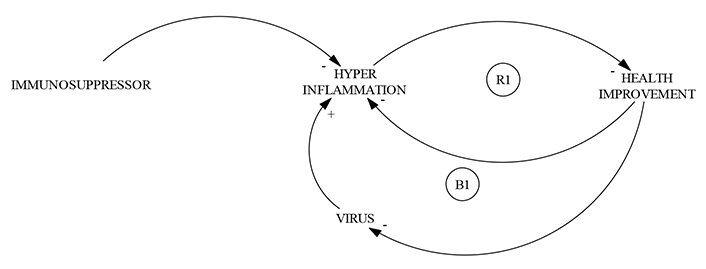
CLD representation of immunosuppressors action in COVID-19. CLD suggests that the action of immunosuppressors in COVID-19 implies the coupling of two cycles, a reinforcing (R1) and a balancing (B1) cycle. CLD is inspired by Hasselbalch et al. [11], who describes the action of SARS-CoV-2 as a mediator of interferon deficiency and hyper inflammation in severe COVID-19 patients. A reinforcing loop between hyper inflammation and health improvement (R1) is important in the evolution of disease thus, the role of immunosuppressors is crucial to diminishing the severity of COVID-19. All CLDs of the present paper were drawn with Vensim PLEx32
Recent advances in the pathophysiology of the SARS-CoV-2 infection have indicated these patients might experience a cytokine release syndrome (CRS), characterized by increased interleukin-6 (IL-6), IL-2, IL-7 and IL-10 levels among others [12]. Therefore, the treatment of cytokine storm has been proposed as a critical part of rescuing severe COVID-19. The mechanism of immunoregulation of hyperinflammation by intervening in the JAK/STAT system (Figure 2), is represented by a CLD with a reinforcing cycle (R1) and six balance cycles (B1–B6), which are carried out in the cytoplasm and into the nucleus of the cell, illustrating the complexity of the process and explaining why sometimes, there are contradictory results of these therapies in COVID-19. The dominance of the reinforcing loop (R1) (Figure 2), indicates a critical role in this initial step of the immunomodulators treatment which can be illustrated by the fact that immune cells constantly patrol the body via the bloodstream, and migrate into multiple tissues where they face variable and sometimes demanding environmental conditions. Nutrient and oxygen availability can vary during homeostasis, and especially during an immune response, creating fine-tuning conditions determining the fate of the treatment in each patient, enhancing the necessity to perform sensitive tests to quantitatively measure JAK-STAT1/2 and JAK-STAT3 signaling pathway activity in immune cells and blood samples, which can contribute to solutions for the COVID-19 crisis in severe patients [13, 14].
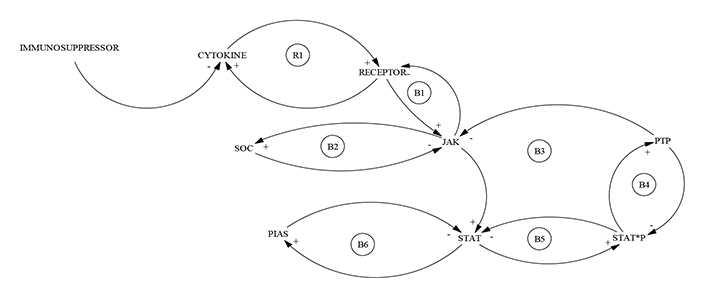
CLD representation of traffic shaping in COVID-19 by controlling JAK/STAT pathways. CLD description was based on current knowledge of regulation of JAK/STAT signaling pathways in the immune system, following Shuai and Liu [13]. It seems clear that the regulation of the JAK/STAT signaling pathway represents a high-level challenge since it involves at least six balanced sub-cycles (B1–B6), which makes the system quite robust, suggesting that intervening in them is a difficult task. Hence, therapies based on the use of immunosuppressants in COVID-19 must be applied under very careful clinical supervision
Data caching
Caching data is a process that stores multiple copies of data or files in a temporary storage location—or cache—so they can be accessed faster, which corresponds in our model, to the activation of innate immunity and its associated trained immunity. In humans, after the skin, the innate component (e.g., epithelial and phagocytic cell enzymes, phagocytes, inflammation-related serum proteins, surface and phagocyte granule antimicrobial peptides) of the immune system is, without a doubt, one of the most effective mechanisms for the control of pathogenic microorganisms [15]. The case of the coronavirus is not the exception either, since more than 80% of those infected with the SARS-CoV-2 virus do not develop the disease or present mild symptoms, a fact that is attributed to the adequate action of the innate immune response mechanisms [16, 17]; which is why, from the perspective of NetOps, the induction of these mechanisms is an object of basic and clinical research of primary importance.
Also related to immune data-caching, recent epidemiological studies have shown that, in addition to disease-specific effects, vaccines against infectious diseases have nonspecific effects on the ability of the immune system to handle other pathogens; for example, there is evidence in animal models that an initial infection or vaccination with bacteria (for example, BCG) protects athymic nude mice against a lethal infection with Candida albicans [18]. Moreover, the increased susceptibility to severe disease in the elderly and individuals with co-morbidities (risk factors) argues for an initial defect in anti-viral host defense mechanisms; therefore, the long-term boosting of innate immune responses could induce heterologous protection against infections in these patients [19, 20] (Figure 3).
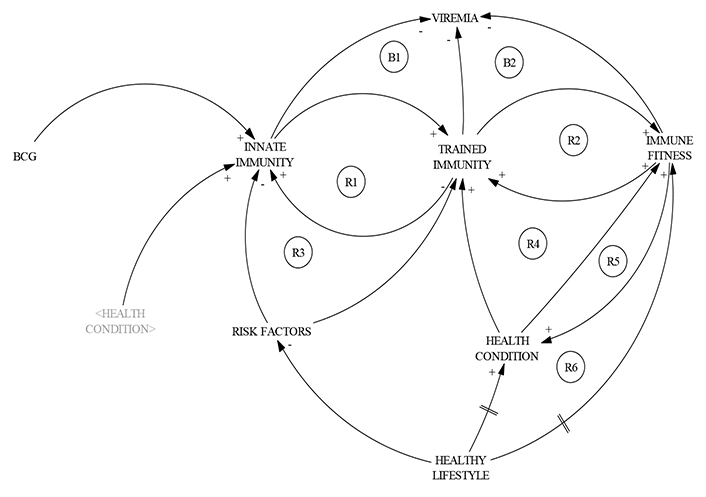
CLD representation of immune data caching. Regulation of innate immunity is the analogy of data caching. This CLD was inspired in Bekkering et al. [19], Burgos-Salcedo et al. [16], and Klement [8]. Cross activation of innate immunity by BCG, influenza, and meningococcal vaccines requires the action, predominantly, of reinforcing sub-cycles (R1–R6), involving, as a baseline, good health conditions derived from a healthy lifestyle. The symbol (//) represents a time delay in the action between two components of the system
Regarding a healthy lifestyle, recent research indicated that optimizing vitamin D blood levels could offer a solution approach that promises a heavily reduced fatality rate as well as solving the public health problem of counteracting the general vitamin D deficiency [21]. On the other hand, taking into account risk factors, an overwhelming preponderance of cases and deaths is observed within the elderly population, especially in those with pre-existing conditions and comorbidities. Immunosenescence and inflamm-aging are considered key features of the aging immune system wherein accumulation of senescent immune cells contributes to its decline and simultaneously, increased inflammatory phenotypes cause immune dysfunction.
On the other hand, a challenge with specific microbial stimuli induces long-lasting epigenetic changes in innate immune cells that result in their enhanced response to a second challenge by the same or unrelated microbial insult, a process referred to as trained immunity. This opens a new avenue in vaccinology to develop TIbV, defined as vaccine formulations that induce training in innate immune cells [22].
Data compression
In IT, data compression is the process of encoding, restructuring, or otherwise modifying data, to reduce its size. This process can be equated, in immunology, with one of the most outstanding aspects of the specific or adaptive immune response, which is the development of immunological memory mechanisms, and without a doubt, effective vaccines are a major operator in these immune NetOps. Significant advances in cutting-edge vaccine technologies over the past decade have resulted in two main types of SARS-CoV-2 vaccines now being approved for emergency use, an unprecedented achievement in modern medical science. The approved vaccines developed by Pfizer and Moderna use mRNA technology and lipid nanoparticle (LNP) delivery systems, while the approved formulations by AstraZeneca, and Johnson & Johnson, contain DNA delivered within non-replicating recombinant adenovirus (AdV) vector systems [23]. Immune data compression by vaccination occurs through two mechanisms (Figure 4). First, in modern immunology it is a well-known fact that effective DNA, RNA, recombinant, and particulate vaccines are made to recognize, not a huge number of antigens of the pathogen but, a relatively small set of antigenic determinants, as the spike protein of coronavirus, conferring long term neutralizing immunity against SARS-CoV-2 through the second impressive compression mechanism, the establishment of a lineage of specific memory B and T cells.
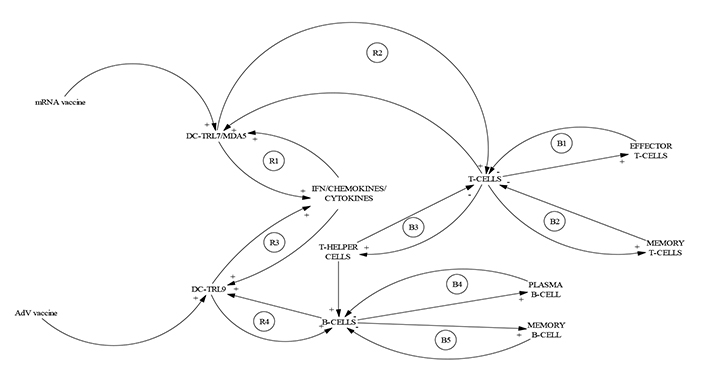
CLD represents the immune data compression generated by COVID-19 effective vaccines. Immune data compression can be represented by the very effective modes of action of COVID-19 vaccines. The CLD graph was taken from Teijaro and Farber [18], and illustrates how vaccines from different technological platforms induce a complex set of signaling pathways involving the action, initially, of reinforcing sub-cycles (R1–R4) and after, the activation of balancing sub-cycles (B1–B5) leading to the establishment of the epitome of data compression: the B and T memory cells
Buffer tuning NetOps
When vaccination against smallpox was introduced around 200 years ago, positive side effects such as protection against measles, scarlet fever, and whooping cough, among others, were noticed [24]. These and many other clinical observations pointed to a long-lasting non-specific collateral benefit associated with these vaccines, regardless of specific priming and subsequent clonal selection of T and B lymphocytes specific for the nominal antigens present in the vaccine [25–27]. Considering that IT buffer tuning consists in operating the network to construct the smallest information unit for effective communication between subtasks, immunologists shortly could use a strategy based on the implementation of the concurrent activation of innate and adaptative immune responses to obtain an immunocompetent state in vaccinated individuals designing a new type of biologicals, for example, TIbV, to confer long-lasting protection with a “buffer” capacity, not only by collateral benefits against other pathogens but generating durable patterns of adaptative immune responses, induced by the multiple-function vaccines (M-vaccines), which stimulates the innate immune compartment in the host (Figure 5) and thereafter, the specific immune compartment.
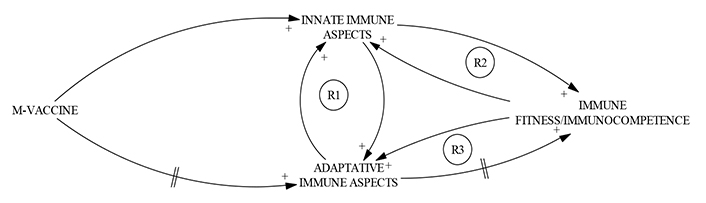
CLD represents immune buffer tuning by concurrent activation of innate and adaptative immune responses by an M-vaccine. The development of new types of vaccines with the capacity to activate; concurrently, the innate and adaptative immune compartments of the immune system is understood here as equivalent to the IT operation of buffer tuning, given the role of this NeTOp in enabling effective communication between well-defined sub-tasks. The CLD was inspired in Sánchez-Ramón et al. [22]. The action of two delay loops (//) can be noted, with the first representing the time lapse between vaccination and the development of the specific immune response, and the second representing the time necessary to generate a state of effective immunocompetence
Conclusions
CLDs functioned as a tool to illustrate the principle of causality of NetOps involved in the management of COVID-19 on the human metabolism and form the basis for the systems analysis approach to study those different treatments actually in use in the global effort to control the pandemic. Such CLDs are instruments for problem identification and problem resolving by breaking down a comprehensive system into fragments to enhance its comprehensibility. Also, visualizing concepts make it much easier to understand complex correlations and casualties (i.e., cause and effect mechanisms). The problem (in this case, immune systems that have difficulties coping with COVID-19) stands at the center of the systems analysis.
Given the complex communication architecture of signal transduction in the immune system, it is apparent that multiple parallel pathways influencing and regulating each other are not the exception but the norm. For delineation of this crosstalk and the revelation of all essential components of signaling cascades downstream of the respective receptor, single-cell proteomics coupled with network-based approaches will be instrumental. Also, a combination for clinical trial success and personalized treatment could be the integration of single-cell technologies—such as fluorescence-activated cell sorting (FACS) or cytometry by time of flight (CyTOF)—with population-level omics, such as proteomics. Taking a deep dive into a novel, mass spectrometry-based plasma proteomics solution for the unbiased discovery of clinically relevant biomarkers will be one of the most useful future directions for biomedical research.
The final central idea, and certainly an urgent necessity, is to make a transition from empirical or quasi-empirical immune NetOps to analytic immune networks operations. The present paper is an initial step in this direction; future developments must include detailed dominance loop analyses and system dynamic modeling of different scenarios inside each type of immune NetOps in COVD-19 and other diseases.
Abbreviations
BCG: | bacillus Calmette-Guerin |
CLDs: | causal loop diagrams |
COVID-19: | coronavirus disease 2019 |
IL-6: | interleukin-6 |
IT: | information technology |
JAK: | Janus kinase |
mRNA: | messenger RNA |
NetOps: | network operations |
SARS-CoV-2: | severe acute respiratory syndrome coronavirus 2 |
STAT: | signal transducer of activation |
TIbV: | trained immunity-based vaccines |
Declarations
Acknowledgments
The author fully acknowledges the intellectual assistance of CIINAS Corp.
Author contributions
The author contributed solely to the work.
Conflicts of interest
The author declares that he has no conflicts of interest.
Ethical approval
Not applicable.
Consent to participate
Not applicable.
Consent to publication
Not applicable.
Availability of data and materials
Not applicable.
Funding
Not applicable.
Copyright
© The Author(s) 2022.