Abstract
Natural killer (NK) cells have a dual role in human reproduction for maternal-fetal tolerance and protection from infection. During the ovarian cycle and pregnancy, peripheral NK (pNK) and uterine NK (uNK) cells dynamically change their proportions and cytotoxicities to prepare and accommodate invading trophoblast and maintain pregnancy. However, dysregulated pNK and uNK cell proportions and cytotoxic activities have been associated with aberrant spiral artery remodeling and trophoblast invasion, leading to implantation failures and recurrent pregnancy losses (RPLs). This review will focus on the role of NK cells in RPLs reviewing the ontogeny of NK cells, changes in pNK and uNK cell levels, and activities during the ovarian cycle, normal pregnancy, and RPL. In addition, the immunopathological role of NK cells in endometrial/decidual vascular development and killer immunoglobin-like receptor (KIR) and human leukocyte antigen (HLA)-C interactions are discussed.
Keywords
Natural killer cells, recurrent pregnancy loss, decidual natural killer, peripheral blood natural killer, natural killer cytotoxicityIntroduction
Human pregnancy is confronted by two major immune challenges: immune tolerance to maintain a semi-allogeneic fetus and immune activation to control infectious pathogens. Natural killer (NK) cells are the major innate immune cell population that participates in paradoxical and bi-directional immune responses at the maternal-fetal interface [1]. NK cells were initially described as large granular lymphocytes (LGLs) that can develop cytotoxicity without any prior exposure to exogenous antigens [2, 3]. In 1980, LGLs were demonstrated to be responsible for human NK activity [4]. NK cells are present in peripheral blood and tissue, including the uterus. In particular, uterine NK (uNK) cells occupy 54.6% (31.2–73.3%) of the decidual lymphocytes during early pregnancy [5], suggesting their role in human reproduction. uNK cells are involved in early defenses against pathogens and produce various cytokines and chemokines to regulate the uterine immune response. Additionally, NK cells have immune-regulatory roles and participate in angiogenesis and vascular remodeling during early pregnancy [6, 7]. Dysregulated NK cells have been implicated in different reproductive pathologies, including recurrent pregnancy loss (RPL), unexplained infertility, and preeclampsia [8–10].
RPL is defined as 2 or more clinical pregnancy losses by the American Society for Reproductive Medicine and European Society for Human Reproduction and Embryology [11, 12]. The estimated incidence of RPL was fewer than 5% of women for 2 consecutive pregnancy losses and 1 % for 3 or more RPL [13]. The progress of understanding principal pathologies for RPL had been modest until the elevated peripheral NK (pNK) cell proportions were reported in women with RPL in 1995 [8]. The discovery of NK cell pathology in women with RPL has initiated numerous studies exploring the role of NK cells in normal pregnancy, reproductive failures, and obstetrical complications related to NK cell pathology. With the advancement of molecular genetic technologies, NK cell pathologies have been documented in their proportion, cytotoxicities, cytokine and chemokine production, and receptor and gene expressions in the peripheral blood, endometrium, and decidua. In this review, the recent data exploring the role of NK cells in RPL are reviewed.
Ontogeny of NK cells
NK cells were originally postulated to only develop from the bone marrow; however, recent studies have suggested that they are able to develop and mature in secondary lymphoid tissues like the spleen and lymph nodes [14]. NK cells initiate their development as hematopoietic stem cells give rise to all leukocytes and red blood cells [15]. Common lymphoid progenitor (CLP) cells, which are fully committed to the lymphocyte lineage [16], differentiate into pre-NK progenitors (NKPs; preNK, preNKPs) under the influence of nuclear factor, nuclear factor interleukin 3 regulated (NFIL3) [17]. Contrarily, under the influence of GATA binding protein 3 (GATA3) [18] and inhibitor of DNA binding 2 (ID2), CLP differentiates into common helper innate lymphoid cell precursor (ChILP) cells (Figure 1) [19, 20].
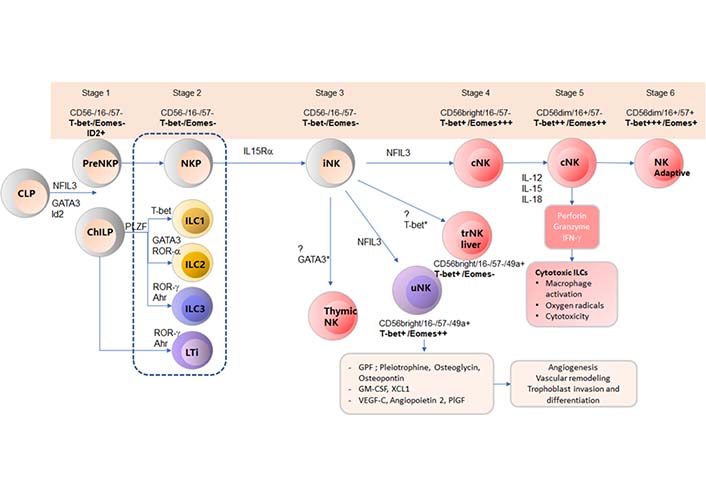
Ontogeny of NK cells. Each stage of NK cell development is characterized by various transcription factors and surface cell markers. NK cells start the differentiation with CLPs under the influence of either NFIL3 or GATA3 and ID2 to produce preNK and ChILP. Stage 1 NK cells include preNKP and ChILP and are characterized by CD56−/CD16−/CD57−/T-bet−/Eomes−/ID2+. PreNKP cells differentiate into NKP cells (stage 2). Stage 2 NK cells include NKP, ILC1, ILC2, ILC3, and LTi and are characterized by CD56−/CD16−/CD57−/T-bet−/Eomes−. Under the influence of IL-15Rα, NKP differentiates into stage 3 NK cells (iNK) that express CD56−/CD16−/CD57−/T-bet−/Eomes−. iNK cells differentiates into tissue-resident NK (trNK) cells with the influence of various transcription factors: thymic NK cells with GATA3, uNK cells (CD56bright/CD16−/CD57−/CD49a+/T-bet+/Eomes++) with NFIL3, liver NK cells CD56bright/CD16−/CD57−/CD49a+/T-bet+/Eomes−) with T-bet, and conventional NK (cNK) cells with NFIL3. Stage 4 NK cells (cNK): CD56bright/CD16−/CD57−/T-bet+/Eomes+++, under the influence of IL-12, IL-15, and IL-18 differentiate into stage 5 cNK cells that are able to secrete perforins, granzymes, and IFN-γ. Stage 5 NK cells CD56dim/CD16+/CD57−/T-bet++/Eomes++. The final stage of differentiation is into adaptive NK cells, stage 6 (CD56dim/CD16+/CD57+/T-bet+++/Eomes+) [16, 21–23]. ILC1: innate lymphoid cells type 1; Eomes: Eomesodermin; T-bet: T box transcription factor; IL-12: interleukin 12; IL-15Rα: IL-15 receptor α; IFN-γ: interferon-γ; iNK: immature NK; PLZF: promyelocytic leukemia zinc finger protein; LTi: lymphoid tissue inducer; ROR-α: retinoic acid related orphan receptor; Ahr: aryl hydrocarbon receptor; GPF: growth promoting factors; PIGF: placental growth factor
NK cells differentiate into 6 different developmental stages that can be identified by their unique expression of transcription factors such as T-bet, Eomes, and various cell surface markers (Table 1) [21, 22]. PreNKP express CD56−/CD16−/CD57−/T-bet−/Eomes−/ID2+ and subsequently differentiate into NKP cells (CD56−/CD16−/CD57−/T-bet−/Eomes−/CD122). Under the influence of IL-15Rα [24], NKP cells undergo a transformation into iNK (CD56−/CD16−/CD57−/T-bet−/Eomes−) cells. In mouse studies, iNK cells differentiate into trNK cells with the influence of various transcription factors, such as thymic NK cells with GATA3 [25], uNK cells (CD56bright/CD16−/CD57−/CD49a+/T-bet+/Eomes++) with NFIL3, and liver NK cells (CD56bright/CD16−/CD57−/CD49a+/T-bet+/Eomes−) with T-bet [16], and cNK cells with NFIL3 [26]. cNK cells are stage 4 NK cells characterized with CD56bright/CD16−/CD57−/CD49a+/T-bet+/Eomes+++. Stage 4 cNK cells further differentiate into stage 5 cNK cells with the influence of IL-12, IL-15, and IL-18 [27]. Stage 5 cNK cells (CD56dim/CD16+/CD57−/T-bet++/Eomes++) are able to secrete perforin, granzymes, and IFN-γ, that are involved in cytotoxicity [28]. The final stage of differentiation is development of adaptive NK cells (stage 6 NK cells, CD56 dim/CD16+/CD57+/T-bet++/Eomes+). Previously, NK cells were regarded as only being members of the innate immune system but recently, the presence of adaptive NK cells was elucidated.
NK cell ontogeny and related markers
NK cell type | Markers | Transcription factor for progression | Next stage in the progression | Source |
---|---|---|---|---|
CLP | IL-7Rα, CD127, Lin– | NFIL3, GATA3, Id2 | Pro-B, Pre-T, ILCs, CD122+ early NKP lineage | Abel et al. [21] |
PreNKP | CD56–/16–,57–/T-bet–/Eomes–/ID2+ | - | NKP | Abel et al. [21] |
NKP | CD56–/16–,57–/T-bet–/Eomes–/CD122 | IL-15Rα | iNK | Sojka et al. [16] |
iNK | CD56–/16–,57–/T-bet–/Eomes–/NK1.1/NKG2D | NFIL3 | cNK | Sojka et al. [16] |
iNK | CD56–/16–,57–/T-bet–/Eomes– | NFIL3 | uNK | Sojka et al. [16] |
iNK | CD56–/16–,57–/T-bet–/Eomes– | T-bet | trNK liver | Simonetta et al. [22] |
iNK | CD56–/16–,57–/T-bet–/Eomes– | GATA3, IL-15 | Thymic NK | Sojka et al. [16] |
uNK | CD56bright/16–, 57–/49a+/T-bet+/Eomes++ | - | - | Simonetta et al. [22] |
Thymic NK | CD127+ | - | - | Sojka et al. [16] |
trNK liver | CD56bright/16–,57–/49a+/T-bet+/EOMES–/DX5– | - | - | Sojka et al. [16], Simonetta et al. [22] |
cNK | CD56bright/16–,57–/T-bet++/EOMES+++/CD49a–/DX5+ | IL-12, IL-15, IL-18 | Stage 5 cNK | Sojka et al. [16], Simonetta et al. [22] |
Stage 5 cNK | CD56dim/16+,57–/T-bet++/EOMES++ | - | Adaptive NK | Abel et al. [21] |
Adaptive NK | CD56dim/16+,57+/T-bet+++/EOMES+ | - | - | Abel et al. [21] |
NKG2D: NK group 2, member D; Lin: Lineage; -: none
The majority of circulating NK cells are classified as stage 5 cNK cells (CD56dim/CD16+) [29], which occupy 10–15 % of peripheral blood mononuclear cells (PBMCs; over 90% of pNK cells), and the remainder (maximum 10% of the total circulating cNK cell pool) are classified as stage 4 cNK (CD56bright/CD16−) cells [14, 30, 31]. They are highly granular cells able to secrete perforin, granzyme, and IFN-γ without prior antigen encounter [32, 33]. In contrast, CD56brightCD16− NK cells, either stage 4 cNK or trNK cells, are poorly cytotoxic and produce various cytokines. trNK cells were shown to produce tumor necrosis factor-alpha (TNF-α) [34], IFN-γ [35], granulocyte colony-stimulating factor (G-CSF), and IL-3 [36], whereas circulating CD56brightCD16− NK were shown to produce IL-10, and granulocyte-macrophage colony-stimulating factor (GM-CSF) [37]. trNK cells have immunomodulatory roles and are abundant in secondary lymphoid tissues and various organs like the liver and uterus [38]. trNK cells differ in phenotype between organs; however, they often express the markers of tissue residency, including CD9, CD49a, or CD69 [16, 39]. In the uterus, trNK cells differ greatly from cNK cells in their ability to tolerate the allogenic embryo. Rather than responding with cytotoxicity to the invading semi-allogenic trophoblasts, uNK cells produce chemokines such as C-C motif chemokine ligand 1 (CCL1), chemokine C-motif-ligand-1 (XCL1) [40], CCL2 [41], CCL3, and CCL4 [42], that interact with C-X-C motif ligand 10 (CXCL10) and CXCL11 [43] to regulate the uterine immune environment. Moreover, uNK cells produce fetal growth-promoting factors and angiogenic factors, including vascular endothelial growth factor-C (VEGF-C), placental growth factor, angiopoietin-1 (ANG1) [44], ANG2, keratinocyte growth factor, platelet-derived growth factor (PGF)-BB, and transforming growth factor β1 (TGF-β1) [45]. With this capacity to secrete various growth-promoting factors, CD56bright uNK cells are believed to have a critical role in angiogenesis, vascular remodeling, trophoblast invasion, trophoblast differentiation, and modulating inflammation [46–48].
Peripheral blood NK cells: count and proportion
Normal ovarian cycle
During a normal ovarian cycle, the proportion of CD3−CD56+ and CD3−CD56dim pNK cells are increased during the mid- (16.43% ± 8.14%, 15.75% ± 7.88%) and late- (16.58% ± 7.22%, 15.91% ± 7.09%) luteal phases compared to the early follicular phase (13.39% ± 6.33%, 12.84% ± 6.22%; P < 0.05, respectively) [49, 50]. This was mirrored by an increase in the total peripheral lymphocytes and IL-4 cytokine production [50]. The change in CD3−CD56+ pNK cell proportions was mainly secondary to the increase in CD56dim pNK cell population, but the proportion of CD56bright pNK cells did not change significantly throughout the ovarian cycle [49].
Normal pregnancy
Previously, we reported that CD56+CD16+ pNK cells proportions, but not CD56+ pNK cells, in early pregnancy were significantly decreased in pregnant women (n = 22) compared to nonpregnant controls (n = 17) [8]. Contrarily, Shi et al. [51] reported that the percentage of CD3−CD56+ NK and CD3+CD56+ NKT cells in pregnant women (n = 18; 8–10 weeks) was higher than that in nonpregnant controls (n = 18; P < 0.05). Higuma-Myojo et al. [52] reported no significant differences in CD56dim and CD56bright pNK proportions in early pregnant women (n = 17) compared to nonpregnant women (n = 17) [52]. Since these studies investigated two independent cohorts (pregnant vs. nonpregnant women) and not one cohort in a longitudinal study design, the impact of normal pregnancy on NK cell proportions should be elucidated further.
With the advancement of pregnancy, pNK cell proportions and pNK counts continue to decrease throughout gestation [53, 54]. In a prospective study of normal pregnant women who delivered without any history of recurrent spontaneous abortion (RSA) or infertility (n = 122), CD56+ NK cell proportions (%) tend to decrease toward the end of pregnancy; however, changes were not statistically significant [6]. Mosimann et al. [54] reported in a study including 133 pregnant women that the total mean [standard deviation (SD)] number of CD56+16+ pNK cells was 232.6 × 106/L (149.5 × 106/L) in the first trimester, 183.4 × 106/L (108.1 × 106/L) in the second trimester, and 166.9 × 106/L (77.7 × 106/L) in the third trimester. There was a significant decrease in pNK cell count from the first trimester to the second trimester (P = 0.045); however, there were no differences between the second and the third trimester. Overall, a significant decrease was noticed in the total number (P = 0.009) and the proportions (P = 0.020) of pNK cells from the first to the third trimester of pregnancy [54]. The reduction of pNK cells is mainly due to the reduction of CD56dimCD16+ NK cells but not CD56brightCD16+ NK cells [54]. Contrarily, Kühnert et al. [55] reported 20–30% lower CD16+ pNK cell numbers and a slightly lower percentage in the first trimester [< 14 weeks gestation (WG)] pregnant women (n = 22) than in nonpregnant women. The NK levels of pregnant women stayed lower during pregnancy and recovered in the postpartum period. However, these changes were not statistically significant. Mahmoud et al. [56] and Veenstra van Nieuwenhoven et al. [57] reported significantly decreased NK cell counts during the third trimester as compared to nonpregnant controls, but this study did not include the first and second-trimester pregnant women. There were no differences in pNK cell numbers between Caucasian and Afro-Caribbean women in each trimester of pregnancy [54]. In summary, pNK cells seem to trend at higher levels in early gestations, decrease throughout pregnancy, and recover to the prepregnancy level in the postpartum period.
Functional characteristics of pNK cells are changed during pregnancy. IL-10+ and IL-10+/TGF-β− pNK cell proportions in nonpregnant women (2.6% ± 3.2%) were reported to be significantly lower than in early pregnant women (24.0% ± 13.3%, P < 0.0001), but IFN-γ+, IL-4+, IL-5+, IL-13+, and TGF-β+ pNK cells were not different between nonpregnant and early pregnant women [52]; however, Shi et al. [51] reported IFN-γ+ NK cells were significantly decreased in early pregnancy (P < 0.01). In general, NK-r1 cells (NKr1) of nonpregnant women (2.5% ± 3.6%) was significantly lower than that of early pregnant women (17.0% ± 10.3%; P < 0.0001) [52], but NK1, NK2, and NK3 cells were not different [52]. When pregnant women’s NK cells and lymphocytes were stimulated in vitro, type 1 cytokine production decreased with no change in type 2 cytokine production compared with nonpregnant women’s NK cells and lymphocytes [57]. Therefore, this suggests a type 1 to type 2 shift in the innate immune system during early pregnancy involves pNK cells.
NK subsets during pregnancy
Several studies have reported no change in the total number of pNK subsets (CD56dim, CD56bright), invariant NKT (iNKT), and NKT cells in the peripheral blood between nonpregnant and pregnant women in the different trimesters [58–60]. pNK cell subsets can be divided into type 1 and 2 subsets via their cytokine production profile [61]. In the third trimester of pregnancy, by using IL-18R antibodies to identify pan type 1 lymphocytes and suppression of tumorigenicity (STL2) antibodies to identify pan type 2 lymphocytes, type 1 NK cells were decreased, type 2 NK cells were increased, and there were overall decreased NK1/NK2 and NKT1/NKT2 ratios in normal pregnant women compared to nonpregnant women and women with preeclampsia [62].
Preconception women with RPL
Multiple studies have reported increased pNK cell proportions and numbers in women with unexplained RPL [8], unexplained infertility [9], and repeated implantation failures (RIFs) (Table 2) [63, 64]. In women with RPL, pNK cell proportions are associated with autoimmunity. Women with RPL and antiphospholipid antibodies (aPL) had a significantly higher proportion of CD56+ and CD56+/16+ pNK cells compared to RPL women without aPL [9]. Additionally, the proportion of CD56+ pNK cells in women with RPL and antiphospholipid syndrome (APS) was significantly higher than that of women with APS alone, RPL of endocrine etiology, RPL of anatomic etiology, and healthy controls [65]. High pNK cell proportions (cutoff > 12%) were present in 52% of RPL of unknown etiology [8] and 56% (> 16.4%) of women with RPL-APS [65]. Thus, a significant portion of women with RPL have NK cell pathology, and NK cells may induce the development of RPL in women with APS [65].
Summary of studies evaluating pNK cell cytotoxicity (pNKC) and proportions in women with a history of RPL
Study | N | Patients | NKC | NK cell proportion/counts |
---|---|---|---|---|
Aoki et al. [66] | 24 | ≥ 2 consecutive 1st-trimester RPL (24) vs. nonpregnant controls (44) | Increased preconception NKC with the association of miscarriage rates compared to controls (71% vs. 20%; RR 3.5, 95% CI = 1.8–6.5) | - |
Kwak et al. [8] | 116 | ≥ 3 RPL, 35 pregnant and 81 nonpregnant women. Nonpregnant controls (17) and pregnant controls (22) | - | Increased in CD56+ in nonpregnant RPL vs. nonpregnant controlsIncreased CD56+ and CD56+/CD16+ pNK cells in pregnant RPL vs. pregnant controls |
Emmer et al. [67] | 43 | > 2 RPL before 16 weeks (43), 37 healthy controls | No difference in nonpregnant RPL vs. controlsIncreased NKC during early pregnancy in the RPL group | No difference in CD56+ and CD56+/CD16+ pNK prepregnancy in RPL vs. controlsIncreased CD56+/CD16+ pNK during early pregnancy in the RPL group |
Morikawa et al. [68] | 113 | 113 nonpregnant women with a history of RPL. 39 controls who subsequently experienced a live birth | Elevated pre-conception NKC in women who miscarried vs. women who delivered (42.8% ± 15.8% vs. 32.1% ± 13.7%; P = 0.09) | CD56+ pNK cells (%) not related to cause and number of RPL |
Yamada et al. [69] | 66 | 66 pregnant women with a history of RPL, no controls included | NKC subsequently decreased in the first trimester in livebirth group 32.5% ± 12.3%, 28.1% ± 12.1%, 28.0% ± 11.8% at 4–5 WG, 6–7 WG, 8–9 WGMiscarriage group with normal karyotype had significantly higher NKC at 6–7 WG 41.2% ± 19% vs. 28.1% ± 12.1% | - |
Yamada et al. [70] | 113 | 113 nonpregnant women with a history of RPL > 2, no controls included | Increased preconception NKC in women who had a SAB or biochemical pregnancy vs. livebirth (47% vs. 33%) | Increased preconception pNK % in women who had a SAB or biochemical pregnancy vs. livebirth (17.1% vs. 13.1%) |
Shakhar et al. [71] | 67 | 38 primary RPL (pRPL)29 secondary RPL (sRPL) | Primary aborters had higher NKC than secondary aborters or controls | Primary aborters had higher pNK % and counts than secondary aborters and controls |
Michou et al. [72] | 99 | 25 RPL30 sporadic SAB33 infertile11 fertile controls | - | Decreased CD56+CD16–CD3– pNK cells in sporadic aborters and infertile women |
Perricone et al. [65] | 53 | APS with (25) and without RPL (28) | - | Increased CD56+ pNK % and counts in patients with RPL and APS than APS alone (15.18% ± 7.32% vs. 8.79% ± 4.68%, P ≤ 0.001) |
Wang et al. [73] | 85 | Nonpregnant women with > 2 consecutive SABs (85) and nonpregnant women controls (27) | - | No difference in peripheral CD56+CD16+ NK cells in controls vs. RPL (16.6 ± 6.0 vs. 16.5 ± 6.5), or in CD56+ 16 – (3.8 ± 1.7 vs. 4.4 ± 2.4) |
King et al. [74] | 104 | Nonpregnant women, with a history of ≥3 consecutive RPL in the mid-luteal phase (104) vs. controls (33) | - | Patients with RPL had higher pNK % (11.9% vs. 8.8%, P < 0.001) and lower CD56bright:CD56dim (0.54 vs. 0.09, P < 0.05) than controls |
Karami et al. [63] | 23 | 23, > 2 RPL patients36, healthy controls | Increased cytotoxicity in RPL vs. controls (32.14 vs. 10.74, P < 0.001) | Increased CD56dim pNK cell proportion in RPL vs. controls (12.94 vs. 5.37, P < 0.001) |
Lee et al. [75] | 95 | ≥3 idiopathic RPL before 20 weeks. 95 nonpregnant women29 fertile controls | RPL patients had increased levels of NKC compared to controls | RPL patients had increased CD56+ pNK cells (%) compared to controls |
Ahmadi et al. [76] | 78 | ≥3 RPL with the current positive pregnancy test38 treated with IVIg, 40 no treatment controls | IVIg treatment lowered NKC after 32 weeks of gestation (P < 0.0001) | IVIg treatment lowered NK cell percentage after 32 weeks of gestation (P < 0.0001) |
CI: confidence interval; NKC: NK cell cytotoxicity; SAB: spontaneous abortion; IVIg: intravenous immunoglobulin; RR: relative risk; N: sample size
Since the early studies were not performed during the window of implantation, Prado-Drayer et al. [77] investigated the pNK cells during the mid-luteal phase. The study revealed an increase in CD56+/CD16+/CD3− (13.9% ± 7.1% vs. 6.0% ± 2.9%, P < 0.002), CD56dim/CD16+/CD3− (6.7% ± 5.9% vs. 0.5% ± 0.7%, P < 0.003) and CD56+/CD69+intracellular/CD3− (3.3% ± 2.2% vs. 1.2% ± 0.8%, P < 0.007) pNK cell proportions (%) in women with RPL as compared to controls [77]. These findings suggest that during the mid-luteal phase, NK cells are activated in women with RPL, and preconception treatment should be considered in women with NK cell pathology. Another study performed during the secretory phase of the ovarian cycle revealed consistent findings: women with RPL had significantly higher CD56dim pNK cells (12.94%, ranges 8.29–23.81%) than controls (5.37%, 2.17−7.82%, P < 0.001) but no differences in CD56bright pNK cells (0.61%, 0.1–1.5% vs. 0.62%, 0.12–0.92%) [63]. Overall, women with a history of RPL have higher pNK cell levels than controls.
Women with an increased proportion of CD56+CD16+ pNK cells at the time of embryo transfer (ET) had an increased risk of in vitro fertilization (IVF) failure compared to women with normal pNK cell proportion [78], suggesting their role in implantation. Furthermore, preconception pNK proportions were significantly higher in the combined groups of women who miscarried normal fetal karyotype and biochemical pregnancies compared to women who delivered a liveborn infant [78]. Thus, it suggests that NK cell pathology is causally associated with miscarriages rather than the result of miscarriages. On the other hand, in women with RPL who miscarried genetically normal babies, pNK cell proportion was not significantly different from live birth [69, 78] indicating pNK cell increase is specific to the genetic profile of miscarriage.
Multiple NK cell-related biomarkers have been studied. King et al. [74] reported that in women with RPL, increased % pNK cells (P < 0.001) and decreased CD56bright:CD56dim ratio (P < 0.05) were significant when compared to controls. In women with a negative RPL screening, % pNK was the only significantly higher variable and highly specific for women with RPL (97.0%). 12.5% of women with RPL had a high % pNK (> 18%), compared with 2.9% of controls [74]. In a study of RPL women investigating activating and inhibitory receptors on NK cells, 12.3% of total CD56+ pNK cells expressed CD69 compared to 6.59% in normal controls (P < 0.005). Both CD56dim and CD56bright pNK cells have significantly higher CD69 expression compared to normal controls (P < 0.008, P < 0.04, respectively). Contrarily, CD94 expression on CD56+ pNK cells was significantly less in women with RPL compared to normal controls (P < 0.005) [79]. However, CD158a, CD158b, and CD161 expression on CD56dimCD16+, CD56brightCD16− pNK and CD56+/CD3+ NKT cells were not different between RPL and normal controls [80]. These findings suggest the unbalanced expression of activating and inhibitory receptors on pNK cells in women with RPL that lead to a hyperactivation status.
Twenty years after the inception of the study looking into NK cells and RPL, a meta-analysis including a total of 13 studies was performed. The study revealed that pNK cell percentages [standardized mean difference (SMD) 1.36; 95% CI = 0.04–2.69; P = 0.04] and numbers (SMD 0.81; 95% CI = 0.47–1.16, P < 0.00001) were significantly elevated in women with RPL, further supporting the initial findings [81]. A few studies have been reported following the meta-analysis, documenting an increase in pre-pregnancy pNK cell percentage and a decrease in the percentage of CD3+ lymphocytes in women with RPL [82]. Additionally, pRPL women had a higher absolute number and pNK cell percentages than sRPL women (defined as having > 1 prior live birth) [71, 83]. Toth et al. [83] reported that both pRPL and idiopathic pRPL (n = 393) showed significantly higher pNK cell absolute numbers and percentages than sRPL and idiopathic sRPL (n = 182) [83]. Subsequently, the same group reported that sRPL patients (n = 25) had significantly lower CD56+ and CD56dimCD16bright, CD56dimCD16brightNKG2D+, and CD56dimCD16brightNKP46+ pNK cell counts than controls who delivered a liveborn infant (n = 25). Hence, pRPL and sRPL may have different immune profiles and etiology. A possible role of adaptive NK cells in sRPL should be investigated further.
Recent advancements in molecular genetic studies contributed to the understanding of pNK cell immunopathology in RPL. Markedly increased proportion of CD56dim pNK cells in women with RPL were reported using single-cell RNA sequencing (scRNA-seq). CD56dim pNK cells have decreased expression of immunosuppressive genes such T cell immunoreceptor with Ig and ITIM domains (TIGIT), killer-cell lectin like receptor G1 (KLRG1), IL-32, and arachidonate 5-lipoxygenase-activating protein (ALOX5AP), while pro-inflammatory genes such as human leukocyte antigen (HLA)-B, nuclear receptor subfamily 4 group A member 1 (NR4A1), Jun proto-oncogene (JUN), JunB proto-oncogene (JUNB), nuclear factor kappa-light-chain-enhancer of activated B cells inhibitor alpha (NFKBIA), and mitogen activated protein kinase (MAP3K) were increased, indicating an overall enhanced pro-inflammatory systemic immune feature in women with RPL [84]. This finding was consistent with the previous study demonstrating type 1 immune shift in pNK cells of RPL and RIF women [85]. In general, the data support that women with a history of RPL have deranged levels of total pNK cells and their subsets with proinflammatory characteristics.
Pregnant women with RPL
During early pregnancy, women with a history of RPL have significantly higher proportions of CD56+CD16+ NK and CD19+ B cells in the peripheral blood than women with normal pregnancies [8, 67, 86]. In women with RPL, regardless of their baseline (nonpregnant) CD56+ pNK cell proportions, women who miscarried during the index pregnancy had significantly increased CD56+ pNK cell proportions during early pregnancy compared to women who delivered a liveborn infant while on immunotherapy [87].
In women with elevated preconception CD56+ pNK cells (> 12%), CD56+ NK cell proportion was significantly higher at 4 WG in women who miscarried than those who delivered a liveborn infant. In women with normal preconception CD56+ NK cells (< 12%), CD56+ pNK cell levels were significantly higher at 6 WG in women who miscarried than those who delivered a liveborn infant [8]. Therefore, pNK cell proportions reached the peak approximately 2 weeks earlier in women with higher baseline pNK levels (> 12%) and subsequently miscarried. Furthermore, increased proportions of pNK cells were correlated with reduced gestational age at the time of miscarriage in women with RPL-APS [65]. Emmer et al. [67] also reported that NK cell proportions (< 12%) are strongly associated with a subsequent liveborn. RPL women had higher pNK cell proportions at 8 WG and 12 WG than controls, mainly due to CD56+CD16+ cell levels. The increase was most noticeable at 8 weeks but was still maintained at 12 WG [67]. NK cell proportion in RPL women during early pregnancy was overall 4% higher than that of controls [67]. Thus, pNK cell proportions may indicate pregnancy outcomes during early pregnancy in women with RPL [8].
pNK cytotoxicity
NKC in the ovarian cycle
NKC generally reflects the functional status of NK cells rather than the absolute numbers or proportions. Souza et al. [88] reported that the NKC of healthy women with regular menstrual cycles (mean age 28.1, range 21–39; n = 30) during the follicular phase [median quartile amplitude (MQA) = 13.9 % + 14.2%] was higher than luteal phase (MQA = 10.8 % + 10.4%) evaluated by flow cytometry (P < 0.0001). Follicular phase NKC was not different from that of men and postmenopausal women [88]. Lee et al. [49] investigated NKC at early-follicular, late-follicular, early-luteal, mid-luteal, and late-luteal phases. In normal fertile women, NKC measured by flow cytometry [at effector:target (E:T) ratio of 50:1, 25:1, and 12.5:1] and chromium-51 (51Cr) release assay [lytic units (LU) at 20%], were significantly increased in the mid-luteal phase compared with the early and late follicular phase [49].
NKC in normal pregnancy
During pregnancy, NKC measured by 51Cr-release assay was increased in the first trimester compared to normal nonpregnant controls, subsequently decreased in the second and third trimester, and increased again for up to 1 month postpartum. The increase in NK activity during pregnancy is secondary to an increase in cytotoxicity of individual NK cells, whereas the subsequent decrease in NK activity is secondary to a decrease in the absolute number of NK cells [53, 89]. In other studies, NKC was reported to remain decreased at 6 months (9–40 weeks) postpartum [89, 90], which was influenced by unidentified postpartum plasma components [90] and returned to normal levels by 9 months.
Since CD69 triggers NKC, NK cell activation marker CD69 has been utilized to estimate NKC. There was an overall increase in CD69+ NK cells across gestation, which stems from the increase of CD56brightCD16+CD69+ NK cells [54]. T-cell immunoglobulin and mucin domain 3 (Tim3) significantly inhibited NKC toward trophoblast cell line HTR8 but not K562 cells via galectin-9, the ligand for Tim3, dependent pathway. In pregnant women during early pregnancy (8–12 WG), Tim3+ pNK cells (%) and serum TGF-β levels were significantly higher than in nonpregnant women [91], suggesting a delicate tolerance mechanism to inhibit NKC towards trophoblast during pregnancy.
Preconception NKC in women with RPL
NKC measures different characteristics of NK cells from the enumeration of pNK cells, and flow cytometric analysis of NKC is reproducible and precise to be applicable for clinical translation [92]. In idiopathic RPL patients, 45% had an increase in NK cell populations, and 38% had elevated NKC [75]. Numerous studies demonstrated that nonpregnant women with RPL had significantly elevated NKC by flow cytometry than nonpregnant controls (Table 2). Karami et al. [63] reported that the median NKC of RPL was 32.14% (15.32–76.14%) which was significantly higher than controls (10.74%, ranges 3.42–18.13%) [64]. Shakhar et al. [71] reported that women with pRPL had a higher NKC (P < 0.01) than controls but not the sRPL, explaining why women with sRPL do not benefit from allogeneic immunotherapy.
Sokolov et al. [93] found NKC against JEG-3 trophoblast cell line. RPL women had higher NKC in the luteal phase than in the proliferative phase, and the luteal phase NKC of women with RPL was significantly higher than that of fertile controls. Contrarily, nonpregnant fertile women have lower NKC during the luteal phase than in the proliferative phase [93].
The area under the curve (AUC) analysis demonstrated that NKC was highly specific for RPL (AUC = 0.649). The cutoff value for NKC at E:T cell ratio 25:1 was 23.8% [75]. Hence, increased NKC may be causally associated with pregnancy losses and predict pregnancy outcomes. Taken together, peripheral measurements of the NK cell population and NKC can be used as diagnostic and therapeutic parameters in patients with RPL.
NKC in pregnant women with RPL
In a prospective study of 66 pregnant women with RPL who subsequently delivered a liveborn infant, NKC was significantly decreased from 4–5 WG (mean ± SD, 32.5% ± 12.3%) to 6–7 WG (28.1% ± 12.1%) and 8–9 WG (28.0% ± 11.8%) [53]. Another study reported that differences in NKC of women with RPL at 8 WG were tapered off by 12 WG [50]. Additionally, NKC was higher in women who miscarried during the index pregnancy. In miscarried women with a genetically normal pregnancy, NKC (at 6–7 WG) was significantly higher than those who delivered. However, this was not seen in miscarried women with a genetically abnormal pregnancy [52]. This study suggested that the consequences of an increased NKC were specific for genetically normal pregnancy.
Increased NKC in women with RPL can be partly explained by the dysregulated Tim3-Galactin (Gal9) pathway. Tim3 could make NK cells secrete cytokines and sustain protective immunity and vascular remodeling while inhibiting NKC toward trophoblasts to maintain local tolerance. However, during early pregnancy (8–12 WG), pregnant women with RPL have significantly lower levels of Tim3+ pNK cells (%) and serum TGF-β concentration than normal pregnant women [91]. Hence, decreased Tim3+ pNK cells may induce increased NKC to trophoblasts in women with RPL.
NKC has been utilized in clinical settings for the diagnosis and treatment of women with RPL. In a recent analysis of NKC, the receiver operating characteristic (ROC) curve analysis of NKC significantly differentiated pregnant women with RPL from normal women [75], demonstrating its value as a biomarker. When NKC and proportions were controlled before ET and throughout the pregnancy with personalized immunomodulatory therapy, the live birth rates per ET cycle and per pregnancy were significantly increased in women with RPL compared to the historical controls [94].
Uterine NK cells
Normal ovarian cycle: endometrial NK cells
In the uterine endometrium, uNK cells compose the predominant leukocyte population. CD56+ uNK cells have been shown to increase their population density within the endometrium starting towards the end of the window of implantation [95]. CD56brightCD16− uNK cells are the major NK cell subset and account for 20% of lymphocytes in the proliferative phase, increase to (40–50%) in the secretory phase, and reach a maximum (70–80%) in the early pregnancy decidua [96, 97]. The abundance of uNK cells suggests that they play a critical role in the implantation and maintenance of pregnancy.
A detailed analysis of decidual cells by mass cytometry revealed 13 separate clusters of cells identified by mass spectrometry. Of the 13 clusters, 5 main decidual innate lymphoid cell types including decidual NK1 (dNK1), dNK2, dNK3, ILC3, and decidual proliferating NK cells (dNKp), were identified in addition to 2 subtypes of pNK cells (CD49alow/Eomesint/T-bethi(++)/CD16+/CD57+ and CD49alow/Eomesint/T-bethi(++)/CD16+/CD57−) [94].
dNK cells
There are potentially 7 different NK cell subsets in the human decidua [98]. The three main subsets of dNK cells are dNK1, dNK2, and dNK3. They are CD56brightCD16− NK cells, co-expressing the tissue-resident marker CD49a [99]. dNK1 cells are the most prevalent and express CD39, cytochrome P450 26A1 (CYP26A1), and beta-1,4-N-acetyl galactosaminyltransferase 1 (B4GALNT1), whereas dNK2 express annexin A1 (ANXA1) and integrin β2 (ITGB2), and dNK3 cells express CD160, KLRB1, and CD103 [100]. Compared to dNK2, or dNK3 cells, dNK1 cells express higher levels of killer immunoglobin-like receptors (KIRs), contain more cytoplasmic granules and enzymes involved in glycolysis [100], and express receptors for HLA-C and -G [101, 102]. The interactions between the dNK1 receptors and HLA-C and HLA-G are involved in trophoblast invasion, vascular remodeling, and maintenance of a local immune tolerance [103, 104]. Following the dNK1 cells, dNK2 and dNK3 cells are the next prevalent subsets associated with chemokine production to interact with maternal dendritic cells and fetal extravillous trophoblasts (EVTs) [98].
dNK cells have a scavenger role in clearing senescent decidual cells to improve the implantation environment [105]. When uNK cells were exposed to embryo culture media of women who failed to implant, uNK cell killing of senescent decidual cells was inhibited, and the media contained high levels of hyaluronan and low levels of hyaluronidase 2. When this media was supplemented with recombinant hyaluronidase 2, uNK cell function was restored [106]. This suggests that dNK cells are involved in sensing low-grade embryos and subsequently rejecting implantation.
Following implantation, dNK cells release angiogenic growth factors including ANG1, Ang2, TGF-β1, and VEGF-C that is associated with spiral artery remodeling during the first trimester [45]. Subsequently, at 12–14 WG, dNK cells aid in trophoblast invasion by potentially increasing matrix metallopeptidase 9 (MMP-9) levels and decreasing apoptosis [107]. Controlling the degree of trophoblast invasion into the maternal decidua is critical for pregnancy outcomes, where excessive invasion can lead to placenta accreta, and inadequate invasion can lead to preeclampsia, preterm labor, and recurrent miscarriages [108]. dNK cells are a source of IFN-γ that have mechanisms of action, including EVT apoptosis and reducing active MMP-2 to limit trophoblast invasion [109]. Additionally, dNK cells can inhibit T helper type 17 (Th17) in an IFN-γ-dependent manner. The accumulation of Th17 in the decidua contributes to fetal loss in a murine model, which was subsequently reported in patients with RPL. By dampening Th17 responses at the maternal-fetal interface, dNK cells have a role in local immune tolerance [110]. Finally, dNK cells are involved in spiral artery remodeling. In humans, spiral artery remodeling occurs in a trophoblast-independent and a trophoblast-dependent state [111]. dNK cells are involved in the trophoblast-dependent phase, where they are able to mediate vascular smooth muscle cell (VSMC) apoptosis [112].
NK cell trafficking
The origin of the uNK cells stems from various sources, including recruitment and differentiation of CD56brightCD16− pNK cells, CD56dimCD16+ pNK cells [113, 114], originating from iNK precursors within the decidua, and maturation from decidual hematopoietic CD34+ precursors [115]. Furthermore, the trafficking, differentiation, and retention of NK cell subsets during different times of a menstrual cycle implies unique functions for each subpopulation of NK cells throughout pregnancy.
From mouse studies, there are two waves of NK cell accumulation. During the first wave, accumulation of NK cells occurs when the endometrium remodels during the decidualization process and is characterized by extracellular matrix breakdown, embryonic invasion, and angiogenesis [116]. Uterine trNK cells predominate during decidualization [117]. During placentation, the second wave of NK cell accumulation occurs as cNK cells are recruited from the periphery and begin remodeling spiral arteries [118]. At the maternal-fetal-decidual interface, CXCL12 expression by trophoblasts induces specific migration of CD16− NK cells [119].
Following the influx of pNK cells, these cells can be further differentiated into dNK cells. The differentiation process involved the interaction of glycodelin-A (GdA) binding to CD16−CD56bright NK cells via its sialylated glycan yielding enhanced expression of dNK markers, CD9 and CD49a. GdA is a product of the secretory endometrial glands that first appear 3 days after the luteinizing hormone (LH) surge and is not present in the proliferative endometrium [120]. Once differentiated, dNK cells regulate angiogenesis and trophoblast invasion via VEGF and insulin-like growth factor binding protein 1 (IGFBP-1) secretion [121]. Hypoxia with TGF-β1 and a demethylating agent increased NK cell expression of KIR and dNK cell markers CD9 and CD49a [122]. The culminating effect of an increased expression of CD9 includes reduced cytotoxicity, a capacity to secrete VEGF [122], and promotion of placentation by uNK cells.
IL-15 is directly involved in the post-ovulatory recruitment and maturation of uNK cells, which is essential for Th2 cytokine production [43, 123]. Additionally, IL-18, via the action of ANG2, affects the destabilization of the spiral arteries allowing for implantation [124–126]. In the human endometrium, IL-18 expression increases during the implantation window [126] and plays a major role in maternal vascular remodeling. However, high concentrations of IL-15 and IL-18 act as pro-inflammatory Th1 cytokines, reflected by the local production of IFN-γ and TNF-α [127, 128]. This, in turn, activates uNK cells to become cytotoxic and subsequently not conducive to implantation. TNF-related weak inducer of apoptosis (TWEAK) offers protection from the effects of the Th1 dominant environment during implantation [129]. A high local endometrial expression of TWEAK along with its receptor can neutralize the effects of IL-18 expression and impairs the maturation of uNK cells, preventing cytotoxicity [129]. Additionally, TWEAK and its ligand, Fn-14, are able to act as immune regulators for the Th1/Th2 cytokine balance in the endometrium [130]. With the downregulation of the cytotoxic effects of NK cells, fetal tolerance is supported for the establishment of a pregnancy.
uNK in normal pregnancy
Over- or under-invasion of trophoblast may lead to a variety of obstetrical complications, including preeclampsia, fetal growth restriction, preterm labor, spontaneous miscarriages, and placental abruption [131]. The depth of the trophoblast invasion requires adequate regulation of the uterine immune environment controlled by uNK cells and other immune effectors. CD56brightCD16− uNK cells comprise the majority of the lymphocytes in the endometrium and progressively increase their density throughout the first trimester. uNK cells are a potent producer of cytokines, including TNF-α, IL-2, IL-4, IL-8, IL-10, GM-CSF, IL-1β, colony stimulating factor-1 (CSF-1), leukemia inhibitory factor (LIF), and IFN-γ [132], which play a crucial part in implantation. uNK cells balance the depth of trophoblast invasion and assist tissue remodeling and placental development at the maternal-fetal interface by interacting with EVT [133].
During the first trimester, the proportions of CD56+, CD56dim, and CD56bright dNK cells are significantly increased compared to those of the peripheral blood. Additionally, the proportions of CD56+/programmed death-ligand 1 (PD-L1)+ and CD56dim/PD-L1+ dNK cells were significantly higher than CD56+/PD-L1+ and CD56dim/PD-L1+ pNK cells of the first-trimester (P < 0.03 respectively) [134]. However, there were no differences in these subsets in the peripheral blood between nonpregnant and first-trimester pregnant women [134]. Increased PD-L1 expression on dNK cells was speculated to contribute to the downregulation of cytotoxic T cells at the maternal-fetal interface.
The study using microarray analysis revealed that dNK cells are quite distinct from pNK cell subsets. Early pregnancy (6–12 WG) dNK cells have 278 differentially expressed genes that showed at least a threefold change (P ≤ 0.001) compared to pNK cells. The majority of these genes are overexpressed by dNK cells. The observed differences might be due to 1) the distinct lineage of dNK cells and 2) the maturation of pNK cells in the decidual microenvironment. Overexpressed genes in dNK cells include CD9 and other tetraspanins [integrins, NKG2C, NKG2E, Ly-49L, KIRs, galectin-1, and placental protein 14 (PP14)], all of which could have an immunomodulatory function during pregnancy [135]. Furthermore, term dNK cells have the different gene and protein expression patterns compared to pNK and first-trimester dNK cells, suggesting term dNK cells are a distinctive type of NK cells. Functionally, degranulation response to K562 and polymalate (PMA)/ionomycin is increased, but a capacity to respond to human cytomegalovirus (CMV) infected cells is lower in term dNK cells than pNK and first-trimester dNK cells. In addition, term dNK cells are not skewed to recognize HLA-C while they were previously in the first trimester [136].
uNK cells in women with RPL
CD56+ uNK cell count in mid-luteal phase endometrium was significantly higher in women with RPL compared to fertile controls (P < 0.0001). CD56+ uNK cell counts were positively correlated with CD56 messenger RNA (mRNA) expression by quantitative real-time polymerase chain reaction (qRT-PCR; r2 = 0.43, P = 0.0137) [137]. The consistent findings were reported in pregnant women with RPL. CD56+ dNK cell count was higher in women who had spontaneous early pregnancy loss (mean ± SD, 57.55 ± 1.79) than in women who underwent termination of pregnancy (50.9 ± 3.46; difference of 6.65, 95% CI = 4.76–8.54, P < 0.0001) [138]. However, contradictory results have also been reported. Moreover, women with idiopathic sRPL showed significantly higher uNK levels compared to patients with idiopathic pRPL (mean ± SD/mm2 288.4 ± 239.3 vs. 218.2 ± 184.5, P = 0.044) but not pRPL or sRPL [83], suggesting possible divergent immune mechanisms for pRPL and sRPL.
The controversies in uNK cell enumeration studies are due to multiple reasons, including the timing of the tissue sampling, sample size, patient population, and variations in technical approach and interpretation. The enumeration of uNK cells by immunohistochemistry can be affected by various factors, such as duration of tissue fixation, the embedding and sectioning processes, selection of areas for assessment, the definition of immunopositive cells, inclusion or exclusion of blood vessels, and therefore, the reported uNK cell densities have been very different by various centers [81, 139]. The standardization of the evaluation method should be established to develop a meaningful clinical test for uNK cell measurement.
A recent study using scRNA-seq revealed that dNKp and dNK1 subsets were decreased by 50% in women with RPL (P < 0.01), whereas the proportion of dNK3 cells was significantly increased by approximately 1-fold (P < 0.05) in women with RPL compared to normal pregnant controls. Additionally, dNK4 subsets are uniquely accumulated in women with RPL. dNK4 subsets are enriched with Fc gamma receptor IIIa (FCGR3A)/CD16 and placenta-specific 8 (PLAC8), indicating an active cytotoxic status of these cells [84]. Additionally, a unique proinflammatory CD45+CD16+ dNK subset is substantially accumulated in the decidua of RPL. These changes indicate that dNK subsets that support embryo growth are diminished proportionally, while the ratio of cytotoxic over immune-active dNK subsets is significantly increased in women with RPL [84]. These changes in dNK cells might be inherited from pNK cells. When pNK cells from RPL were converted to induced dNK cells under hypoxia, IL-15, and TGF-β conditions, KIR2DL1 and Ig-like transcript 2 (ILT-2) expression on induced dNK cells were lower in women with RPL than in healthy controls. In addition, induced dNK cells from RPL were more sensitive to necrosis and produced a lower amount of VEGF than controls [140].
In women with RPL and RIF, endometrial gene expression was significantly different from that of peripheral blood; 2.3 fold more CD56, 2 fold less NKp46, 3 fold less CD16A, 4 fold less NKp30, and 10 fold less CD3ε expressions [141]. Lédée et al. [142] reported that endometrium from RPL patients had 2,477 differentially expressed genes compared to fertile controls, mainly involving DNA transcription and expression, implicating the extent of the preconception dysregulation. Furthermore, cytokine production and response to the cytokines in the CCL pathway were more active in dNK cells of RPL than in controls. There was up-regulated expression of inflammation-related genes such as CD69 and IFN regulatory factor 9 (IRF9) in dNK3 cells, increased CXCL8 and IFN induced protein with tetratricopeptide repeats 3 (IFIT3) in dNK4 cells, while anti-inflammation genes were down-regulated, including serine protease inhibitor Kazal-type 2 (SPINK2) and amphiregulin (AREG) in dNK1 cells from RPL patients than controls [84]. In women with RPL, C-X-C chemokine receptor 4 (CXCR4)+ dNK cells with enhanced immunomodulatory potential and their ability to induce Th2 differentiation were diminished [143]. CXCR4+CD56bright dNK cells are known to promote Th2 shift in an IL-4-dependent manner, can be recruited from peripheral blood, and be reprogramed by trophoblasts. Therefore, women with RPL have proinflammatory dNK cell subsets with limitations to induce a Th2 shift.
Angiogenesis
Normal pregnancy
The uterus is the only human organ capable of rapid, repeated cycles of epithelial regeneration and angiogenesis in the body [144]. During pregnancy, rapid angiogenesis and remodeling of the maternal spiral uterine arteries (SUAs) occur throughout the decidualization and placentation phases [145]. Many studies have linked insufficient angiogenesis or vascular remodeling to intrauterine growth restriction (IUGR), RPL, RIF, or hypertensive disorders of pregnancy [146, 147]. The role of uNK cells in the progression of SUA remodeling is well-established, though the molecular mechanisms underlying this require further study.
Angiogenesis and vascular remodeling in the uterus before and in early pregnancy require two carefully orchestrated steps: decidualization and placentation. SUA elongates during the proliferative phase and forms a rich capillary plexus beneath the epithelium [148]. During decidualization, SUA destabilizes as VSMCs begin to migrate away from the SUA, the lumen dilates, and vascular permeability increases [118]. After implantation, SUA endothelial cells (ECs) are replaced by endovascular trophoblasts [148–150]. The invasion of the endovascular trophoblasts accumulates and forms a trophoblast plug that allots only the passage of plasma, causing an oxygen gradient between the maternal and incoming fetal system [151].
The two subpopulations of uNK cells contribute differently to uterine angiogenesis and vascular remodeling. RNA sequencing and mouse studies have shown trNK cells are responsible for the initial vascular destabilization and angiogenesis during decidualization [152]. Growth factors such as Ang-1 and Ang-2, TGF-β, VEGF, and osteopontin, regulate this destabilization [23, 149]. The leakiness of the vasculature in this phase allows for the influx of cNK cells into the implantation site, which contributes to SUA remodeling, likely through secretion of IFN-γ [153]. Additional studies have suggested that cell-cell interactions between uNK cells and the maternal endothelium may contribute to placentation. Ephrin-B2, a marker of arterial VSMCs and ECs, is expressed by SUA and uNK cells before pregnancy [154]. As pregnancy progresses, SUA loses ephrin-B2 and upregulates its receptor, EphB4 [154]. Blood vessels expressing either ephrin-B2 or EphB4 adopt the characteristics of arteries or veins, respectively [155, 156]. As the placenta reorganizes and SUA dilates, it is likely that uNK cells and endovascular trophoblasts utilize ephrin-B2 to induce SUA remodeling and orchestrate placentation (Figure 2).
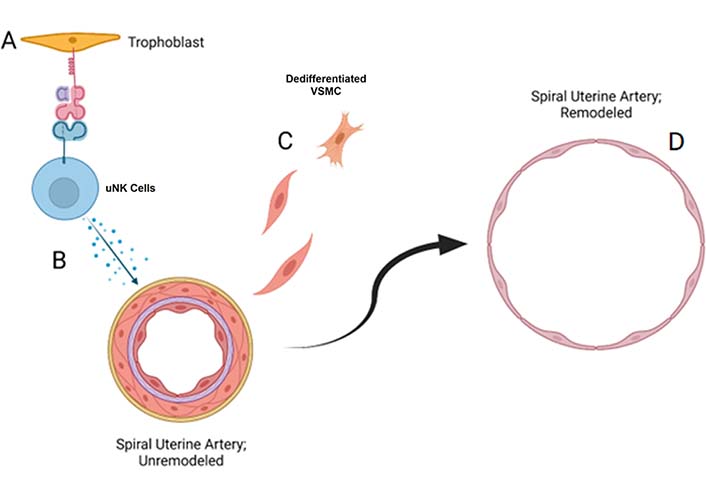
uNK cells induce spiral uterine artery remodeling through secretion of proangiogenic cytokines. (A) NK cells in decidua communicate with EVT cells through HLA-G-KIR2DL4 interactions; (B) dNK cells secrete various proangiogenic factors that increase vascular permeability and induce dedifferentiation of VSMCs; (C, D) SUAs have lost the vascular smooth muscle coat and are dilated in early pregnancy
Dysregulated angiogenesis of women with RPL
In women with RPL, a significantly increased uNK cell density was noticed when compared to controls [137]. The number of uNK cells was positively correlated with vascular myocytes detected by anti-smooth muscle myosin (SMM) and CD31+ vascular endothelial cells. Spiral arteries of these women had increased vascular wall thickness, increased vascular density, and decreased luminal diameter than fertile controls, suggesting the increased number of uNK cells may contribute to a deranged spiral artery remodeling and lead to RPL [137]. This was further supported as women with RPL showed increased density of uNK cells in correlation with formation of blood vessels (P = 0.005, r = 0.5), and spiral arterial smooth muscle differentiation (P = 0.01, r = 0.5) [146].
In pregnant women with RPL (5–7 WG), increased pNK cell proportion was correlated with decreased uterine radial artery blood flow [157]. Women who miscarried the index pregnancy had significantly higher uterine radial artery resistance index at 8 WG than women who delivered a liveborn infant [158]. The association of pNK cell proportion and uterine radial artery blood flow might be explained by pNK cell trafficking to the uterus, but further studies are needed.
The study evaluating IL-15, IL-18, and CD56+ uNK cells via endometrial biopsy correlated the factors with uterine artery dopplers in patients with RIF via IVF. The uterine artery pulsatility index was negatively correlated with IL-18, suggesting cytokine involvement in vascular remodeling. In addition, CD56+ uNK cells were correlated with IL-15 and IL-18, indicating that there is local recruitment and activation of uNK cells [159]. Additionally, the ratio of IL-15/Fn-14 mRNA expression reflected uNK cell activation and maturation, and the ratio of IL-18/TWEAK mRNA ratio was related to angiogenesis and Th1/Th2 balance. Abnormal ratios of IL-15/Fn-14 and IL-18/TWEAK mRNA expression and CD56+ uNK cell counts were reported in 81.7% of RIF patients [160].
TWEAK is a member of the TNF superfamily that has been shown to be expressed on immune cells, including NK cells [161], and is able to influence angiogenesis when combined with its receptor Fn-14 [162]. The study culminating IL-18, TWEAK, IL-15, Fn-14, and CD56+ uNK cells suggested that a high IL-18/TWEAK ratio reflects excessive uNK recruitment, whereas a low IL-15/Fn-14 ratio may indicate uNK depletion [130], alluding to potential markers for uterine receptivity. Taken together, women with RPL have excessive NK cell infiltration that induces dysregulated angiogenesis and a deranged endometrial gene profile that leads to failed pregnancies.
Killer immunoglobulin-like receptors
KIR function in normal pregnancy
dNK cells have different receptor expression profiles [163] than pNK cells, with abundant expression of KIRs. KIRs are a family of transmembrane glycoproteins that are expressed on the surface of NK cells [164] and have a multitude of diversity subcategorized into allelic polymorphism, genotype, ligand, expression, and function. The importance of certain maternal KIR and fetal HLA-C ligand combinations may be more favorable to reproductive success due to the differential overall signals that NK cells receive. KIRs have been shown to be regulators in the development, tolerance, and activation of NK cells [31]. KIRs are able to bind major histocompatibility complex (MHC) class 1 molecules, including HLA-A, HLA-B, and HLA-C. Their role is to protect healthy “self” cells and render targets susceptible to NK cell attacks of “non-self” cells [165]. KIRs have also been shown to bind to trophoblasts that express other non-classical MHC class 1 molecules, including HLA-C, HLA-E, and HLA-G [166]. HLA-C is recognized by the KIR family, of which expression is induced in utero [167]. HLA-E has a high affinity for inhibitory receptor CD94 on uNK cells that leads to inhibition of NK killing [168], and HLA-G is able to bind leukocyte immunoglobulin-like receptors (LILR) B1-Fc and increase the production of IL-6 and IL-10 [169]. Finally, a soluble form of HLA-G is able to bind to endosomal KIR2DL4 that allowing for the activation of NK cells for cytokine and chemokine secretion to promote a proinflammation and a proangiogenic response (Figure 3) [170].
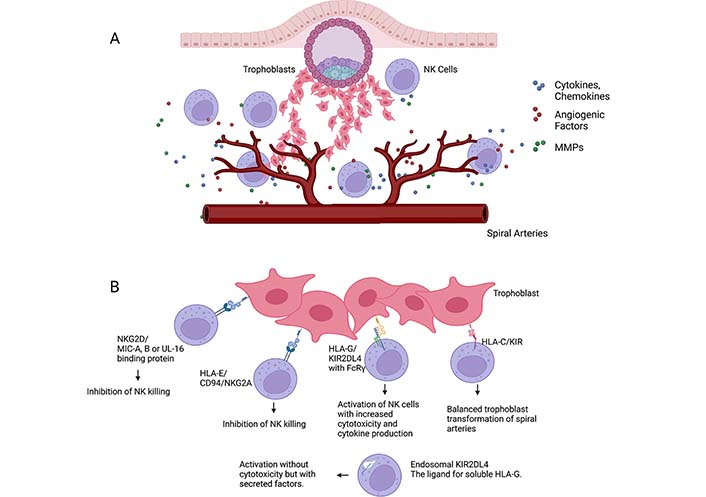
NK cell interactions. A) NK cells in decidua interact with invading trophoblast and secrete various cytokines, chemokines, angiogenic factors, and enzymes for tissue remodeling, such as matrix metalloproteinases (MMPs). NK cells in decidua secrete angiogenic factors (VEGF-C, PGF, ANG1, ANG2), chemokines (CCL1, XCL1, CCL2, CCL3, CCL4), cytokines (IL-15 and IL-18) and MMP-9; B) NK cell receptor interactions with HLA-C, HLA-G, and HLA-E on trophoblasts. HLA-E/CD94/NKG2A interactions lead to inhibition of NK killing. HLA-G/KIR2DL4 with FcRγ leads to activation of NK cells with increased cytotoxicity and cytokine production. HLA-C/KIR interactions allow for a balanced trophoblast transformation of spiral arteries, whereas endosomal KIR2DL4/HLA-G allows for a proinflammatory and proangiogenic response. Additionally, dNK cells interact with trophoblast cells via their NKG2D receptor and MHC class I chain-related (MIC) proteins A and B, or UL-16 binding proteins (ULBPs), inhibiting dNK cytotoxicity. FcRγ: FC receptor γ-chain
When bound to dNK cells, KIRs have been shown to either express inhibitory or activating effects, where inhibitory signals prevent NKC. Inhibitory KIRs include KIR2DL1, KIR2DL2, KIR2DL3, KIR2DL5, KIR3DL1, KIR3DL2, and KIR3DL3 [171] that bind MHC class 1 molecule, HLA-C [172]. Contrastingly, activating receptors, including KIR2DS1, KIR2DS2, KIR2DS3, KIR2DS4, and KIR2DS5 [171], transduce their signals through transmembrane proteins including CD3, FCERIy, and DAP12 [173]. As HLA-C on EVT interacts with different KIRs on dNK cells, immune tolerance is allotted to occur via the inhibitory KIR receptors leading to a reduced risk of pregnancy complications [103].
Besides the HLA-C and KIR interaction, the interaction between NK cell receptor NKG2D and its ligands, MIC proteins A and B, or ULBPs have been shown to inhibit dNK cytotoxicity [174]. When NKG2D is bound to its ligands, it promotes NK cell activation, secretion of IFN-γ, and degranulation [175] to eliminate damaged or infected cells. MIC is constitutionally expressed in the human placenta [176] and released in a soluble form by syncytiotrophoblast-derived extracellular vesicles during pregnancy. MIC from the placenta has been previously shown to be increased in levels in maternal blood in conjunction with decreased expression of NKG2D receptors on PBMCs [103]. The soluble MIC molecules were able to interact with NKG2D receptors and subsequently inhibit the NKG2D-dependent cytotoxic response suggesting another mechanism for fetal immune escape and decreased NKC.
RPL and KIR
The role of KIRs and HLA-C matching has been studied as a potential etiology of RPL. Hiby et al. [177] reported that in patients with RPL, maternal expression of the KIR AA haplotypes lacking activating KIRs was significantly higher, and HLA-C2 frequency was significantly higher in male partners [177]. Particularly, the frequency of the activating KIR2DS1 (the activating KIR for HLA-C2 group) was significantly lower in women with RPL [P = 0.00035, odds ratio (OR) = 2.63, 95% CI = 1.54–4.49]. Contrarily, maternal KIR B haplotype and fetal C1 homozygosity are protective [177]. Thus, KIR2DL2 maintains proper inhibition of NK cell responses against HLA-C C1/C1 fetal cells. In contrast, the weak inhibitor KIR2DL3 may not properly inhibit NK cell response against allogeneic HLA-C2 ligands, resulting in miscarriage [178].
The association of KIR2DS1 and HLA-C2 was further elucidated and an association of maternal KIR2DS1 with an increased frequency of maternal HLA-C2 was reported in women with RPL (HLA-C2 allele frequency 0.5159 in KIR2DS1+ vs. 0.3684 in KIR2DS1− women, P = 0.014) [179]. KIR2DS1 and HLA-C2 ligands contribute to functional NK cell education and regulate NK cell function [178, 180, 181]. Hence, the extent of dNK cell response can be determined by the KIR ligand on the trophoblasts and the maternal HLA-C background responsible for NK cell licensing and education. The 2DS1+ NK cells with inhibitory KIR for self-HLA-class I was commonly noncytotoxic, and 2DS1+ NK cells from HLA-C2 homozygous donors were frequently tolerant to HLA-C2 [178]. Therefore, the prevalence of maternal HLA-C2 among KIR2DS1+ women with RPL may lead to the hyporesponsiveness of NK cells in the decidua leading to impaired placentation. Contrarily, activation of KIR2DS1+ dNK cells by HLA-C2 increases the production of GM-CSF to enhance trophoblast migration which may be beneficial for placentation [182].
In the in-depth study evaluating KIR2DL2 (inhibitory KIR) and HLA-C genotypes (C1C1 vs. C2C2), partners of women with RPL have increased frequency of HLA-C C2C2 and lower expressions of HLA-C C1C1. This combination subsequently leads to the KIR2DL2+ NK cells not being exposed to its ligand and not being “educated.” The lack of education leads to low NK functional activity and subsequent pregnancy loss [183]. However, the impact of hypofunctional NK cells was abated with immunotherapy, using prednisone and intravenous immunoglobulin G. There were no differences in maternal HLA-C genotype and allele frequencies between KIR2DL2+ and 2DL2− women with RPL who delivered a liveborn infant with immunotherapy [183]. Thus, it is plausible that strong inhibitory KIR2DL2 inhibits NK cell responses against HLA-C1 fetal cells properly. In contrast, the weak inhibitor KIR2DL3 may fail to properly inhibit NK cell response against allogeneic HLA-C2 ligand, resulting in miscarriage. Interestingly, David et al. [181] reported that KIR2DL2 recognizes HLA-C2 allotypes, and activation of NK cells could increase the missing-self response of poorly educated C1−KIR2DL2+ and KIR2DL3+ NK cells [181]. This mechanism might explain how an infant is rescued from unfavorable KIR2DL2 and fetal HLA-C2 matches.
Multiple studies have been reported regarding HLA-C and KIR typing in women with RPL (Table 3). Ozturk et al. [184] reported that the KIR Bx genotype frequency was significantly higher, and the frequency of the AA genotype was significantly lower in RPL patients compared to controls (P = 0.014). Additionally, KIR2DL1 with both partners being HLA-C C2/C2 was higher in controls than RPL women (P = 0.0004, OR = 0.28, 95% CI = 0.13–0.58), while KIR2DS2 with both partners being HLA-C C1/C1 was higher in RPL women than controls (P = 0.002, OR = 2.83, 95% CI = 1.47–5.40) [185]. However, Witt et al. [186] reported no association between maternal KIR expression and RPL [186]. The following Table 3 summarizes previous studies.
KIR and HLA-C combination and RPLs
References | AA/Bx frequency | KIR | KIR/HLA combination |
---|---|---|---|
Yang et al. [183] | No difference | No difference | KIR2DL2 with maternal C2; a higher risk |
Dambaeva et al. [179] | No difference | No difference | KIR2DS1 with maternal C2; a higher risk |
Alecsandru et al. [187] | AA type has a higher risk of miscarriage after DET vs. AB or BB haplotypesNo difference in SET | No difference | No paternal or egg donor study |
Wang et al. [188] | - | Decreased KIR2DL1/S1 | - |
Ozturk et al. [184] | Increased Bx genotype | No difference | - |
Faridi et al. [185] | No difference | No Difference | KIR2DL1 with both partners HLAC2/C2; decreased riskKIR2DS2 with both parent’s HLA C1/C1; increased risk |
Nowak et al. [189] | No difference | No difference | HLA-C1C2 women with KIR AA and HLA C2C2 partners, the trend towards increased chances of a successful pregnancy |
Hiby et al. [177] | - | - | KIR AA women; increased risk only when the fetus has more C2 than the mother |
Faridi et al. [190] | Increased BB genotype | No difference | - |
Vargas et al. [191] | - | Increased (5 or 6 out of 6) activating KIR repertoire in women with RPL. No difference in gene frequency | - |
Hiby et al. [192] | AA genotype increase | KIRS2DS1 decrease | HLA-C2 increase |
Hong et al. [193] | No difference | KIR2DL2 increase | No difference |
Wang et al. [194] | - | KIR2DS1 increase | KIR2DS1 negative women with decreased HLAC2 |
Flores et al. [195] | AA genotype increase | KIR2DL2 decrease | - |
DET: double ET; SET: single ET
Conclusions
Pregnancy is a condition where a balance is needed between the immune tolerance of a semi-allogeneic fetus and immune activation to control infectious pathogens. NK cells are one of the primary cell populations participating in these immune responses with varying degrees of regulation. Regulation can be through differentiation, recruitment, cytokine production, interactions with EVT, and hormonal changes (Figure 3). Instability in NK cell regulation and the disrupted balance may lead to RPL and other obstetrical complications. Women with RPL have dysregulated pNK cell counts, proportions, cytotoxicities, gene expression patterns, and differential proportions of dNK cell subsets. This results in abnormal angiogenesis, vascular remodeling, and abnormal uterine blood flow patterns. These characteristics have already been translated into clinical medicine and applied for the diagnosis and treatment of RPL. Specific targeted treatment at the particular time of conception or pregnancy can be offered to control the NK cells mediated pathology. Intravenous immunoglobulin G, glucocorticoids, cyclosporin A, and vitamin D have been utilized to control NK cells for women with RPL [94, 196, 197]. In conclusion, women with RPL have pro-inflammatory type 1 shift in pNK and dNK cells and increased NKC in pNK. Further characterization of pNK and dNK cells concerning reproduction shed light on the understanding of NK cell function and advancement of clinical management for women with RPL.
Abbreviations
ANG1: | angiopoietin-1 |
APS: | antiphospholipid syndrome |
CCL1: | C-C motif chemokine ligand 1 |
ChILP: | common lymphoid progenitor differentiates into common helper innate lymphoid cell precursor |
CI: | confidence interval |
CLP: | common lymphoid progenitor |
cNK: | conventional natural killer |
CXCL10: | C-X-C motif ligand 10 |
dNK1: | decidual natural killer 1 |
Eomes: | Eomesodermin |
ET: | embryo transfer |
EVTs: | extravillous trophoblasts |
GATA3: | GATA binding protein 3 |
GM-CSF: | granulocyte-macrophage colony-stimulating factor |
HLA: | human leukocyte antigen |
ID2: | inhibitor of DNA binding 2 |
IFN-γ: | interferon-γ |
IL-12: | interleukin 12 |
IL-15Rα: | interleukin-15 receptor α |
ILC1: | innate lymphoid cells type 1 |
iNK: | immature natural killer |
KIRs: | killer immunoglobin-like receptors |
LTi: | lymphoid tissue inducer |
MHC: | major histocompatibility complex |
MIC: | major histocompatibility complex class I chain-related |
MMP-9: | matrix metallopeptidase 9 |
mRNA: | messenger RNA |
NFIL3: | nuclear factor interleukin 3 regulated |
NK: | natural killer |
NKC: | natural killer cell cytotoxicity |
NKG2D: | natural killer group 2, member D |
NKPs: | natural killer progenitors |
OR: | odds ratio |
PD-L1: | programmed death-ligand 1 |
pNK: | peripheral natural killer |
preNKPs: | pre natural killer progenitors |
pRPL: | primary recurrent pregnancy loss |
RIFs: | repeated implantation failures |
RPLs: | recurrent pregnancy losses |
SD: | standard deviation |
sRPL: | secondary recurrent pregnancy loss |
SUAs: | spiral uterine arteries |
T-bet: | T box transcription factor |
TGF-β1: | transforming growth factor β1 |
Th17: | T helper type 17 |
Tim3: | T-cell immunoglobulin and mucin domain 3 |
TNF-α: | tumor necrosis factor-alpha |
trNK: | tissue-resident natural killer |
TWEAK: | tumor necrosis factor-related weak inducer of apoptosis |
uNK: | uterine natural killer |
VEGF-C: | vascular endothelial growth factor-C |
VSMC: | vascular smooth muscle cell |
WG: | weeks gestation |
Declarations
Author contributions
TL wrote the first and final draft of the manuscript and the sections of the paper. Involved in writing various sections of the manuscript. JKK contributed to the conception and design of the paper. Additionally, she was involved in writing the final draft of various sections. KW wrote the section regarding angiogenesis. LA, AT, UG, SD and KB contributed to manuscript revision, read, and approved the submitted versions.
Conflicts of interest
The authors declare they have no conflicts of interests.
Ethical approval
Not applicable.
Consent to participate
Not applicable.
Consent to publication
Not applicable.
Availability of data and materials
Not applicable.
Funding
Not applicable.
Copyright
© The Author(s) 2022.