Abstract
Aim:
Atopic dermatitis (AD) is a pruritic, chronic inflammatory skin disease. Thymic stromal lymphopoietin (TSLP) is highly expressed in the epidermis of patients with AD and induces T helper 2 (Th2) immune responses and itching. Although the mechanism underlying the stimulus-induced TSLP production in normal keratinocytes has been intensively studied, whether the production capability of TSLP is naturally enhanced in epidermal cells in AD conditions remains unclear. Previous studies demonstrated that a deficiency of polyunsaturated fatty acid (PUFA) causes AD-like pruritic skin inflammation in special diet-fed hairless mice. The aim of the study was to examine the TSLP production capability of epidermal cells isolated from diet-induced AD mouse model and its mechanism.
Methods:
Epidermal cells were isolated from normal and AD mice and incubated under unstimulated culture conditions to assess spontaneous TSLP production. Messenger ribonucleic acid (mRNA) and protein levels of TSLP were determined by real-time polymerase chain reaction (PCR) and enzyme-linked immunosorbent assay (ELISA), respectively.
Results:
TSLP level was markedly increased in the skin of AD mice. When epidermal cells were isolated from AD mice and cultured without stimulation, Tslp gene expression was upregulated, and a large amount of TSLP protein was extracellularly released. Such TSLP overproduction was not observed in the epidermal cells of normal mice. TSLP overproduction in AD epidermal cells was almost completely inhibited by extracellular calcium chelation, interference with plasma membrane interaction of stromal interaction molecule 1 (STIM1), blockade of the calcium release-activated calcium (CRAC) channels Orai1 and Orai2, or treatment with a PUFA γ-linolenic acid (GLA).
Conclusions:
Epidermal cells isolated from AD mice can spontaneously produce TSLP through STIM/Orai-mediated calcium entry, and GLA may negatively regulate this TSLP production.
Keywords
Atopic dermatitis, thymic stromal lymphopoietin, epidermal cells, calcium entry, stromal interaction molecule, Orai, polyunsaturated fatty acidIntroduction
Atopic dermatitis (AD) is a chronic inflammatory skin disease characterized by itching, skin barrier dysfunction, and immune abnormalities [1–3]. T helper 2 (Th2)-type immune responses have been observed in skin lesions of patients with AD, and cytokines produced by Th2 cells, such as interleukin-4 (IL-4), IL-13, and IL-31, are considerably involved in AD development [4, 5]. However, the precise pathogenic mechanisms of AD are not fully understood. Currently, topical corticosteroids, immunosuppressants, biologics targeting Th2 cytokines, and Janus kinase inhibitors are commonly used to treat AD [6, 7], but there are still unmet needs in the treatment of AD [8, 9].
Thymic stromal lymphopoietin (TSLP), a cytokine with high homology to IL-7, is highly expressed in the epidermis of patients with AD [10]. TSLP acts on the TSLP receptors (TSLPRs) of dendritic cells, inducing T cell differentiation into Th2 cells [10], and the TSLPR of sensory neurons, inducing itching [11]. Mice overexpressing TSLP in the epidermis exhibit AD symptoms characterized by Th2 inflammation [12]. AD development in mice requires TSLP-TSLPR signaling in Langerhans cells [13, 14]. Therefore, TSLP is considered a critical factor in AD pathogenesis.
Environmental pathogens, allergens, and chemicals related to AD induce TSLP expression in epidermal cells through diverse mechanisms [15]. The three typical mechanisms are as follows: (1) TSLP gene expression is induced when normal human epidermal keratinocytes are stimulated with Toll-like receptor (TLR) ligands [16–18]. Proinflammatory cytokines [e.g., tumor necrosis factor-α (TNF-α)] and Th2 cytokines (e.g., IL-4) induce or enhance TSLP production [16–18]. TSLP gene expressions by such stimulations are mediated mainly via the activation of nuclear factor-kappa B (NF-κB) and are suppressed by glucocorticoids [15, 19]; (2) calcium-dependent signaling induces TSLP gene expression when the protease-activated receptor 2 (PAR2) is activated by agonists or proteases [11]. This TSLP gene expression is mediated through the activation of calcineurin and its downstream effector, the nuclear factor of activated T cells transcription factor [11]; (3) topical application of nuclear receptor agonists, including the vitamin D3 and retinoic acid receptors, causes TSLP production in the mouse skin by relieving retinoid X receptor (RXR)-mediated transcriptional repression of Tslp genes [20]. Since TLR ligand-induced TSLP gene expression in keratinocytes is suppressed by glucocorticoids but not by calcineurin inhibitors [21], the NF-κB- and calcium/calcineurin-mediated mechanisms are likely independent. On the other hand, RXR acts to repress IL-1β-induced TSLP gene expression by preventing NF-κB [22].
Increased TSLP production capability in epidermal cells may also be involved. Interestingly, a cell line that overproduces TSLP has been established. In the mouse keratinocyte cell line KCMH-1, Tslp gene expression is constitutively activated, and high amounts of TSLP are produced even under unstimulated culture conditions [23]. Both glucocorticoid dexamethasone (DEX) and an RXR agonist inhibit TSLP overproduction in KCMH-1 cells [23].
A unique diet-induced AD mouse model has been previously reported. AD-like skin inflammation develops when HR-1 hairless mice are fed a special diet (named HR-AD) [24]. In addition, TSLP and Th2 cytokines increased in the skin of HR-AD-fed mice [25]. A previous study revealed that diet-induced AD is mainly due to polyunsaturated fatty acid (PUFA) deficiency [26]. Our recent preliminary experiment also showed that topical application of an n-6 PUFA γ-linolenic acid (GLA) almost completely suppressed TSLP production in the skin of HR-AD-fed mice (M. Fujii, unpublished observation); however, the mechanism is still unclear.
Although, as mentioned above, the mechanism underlying the stimulus-induced TSLP production in normal keratinocytes has been intensively studied, whether the production capability of TSLP is naturally enhanced in epidermal cells in AD conditions remains unclear. Therefore, the present study compared the TSLP production capability of epidermal cells isolated from normal and AD mice. Furthermore, the mechanism of TSLP production in AD epidermal cells was analyzed pharmacologically.
Materials and methods
Animals
Four-week-old female hairless mice (Hos:HR-1) were purchased from Hoshino Laboratory Animals (Saitama, Japan). All mice were maintained with free access to food and water and housed at 22°C ± 1°C under a 12-h light/dark cycle.
Drugs
The following drugs were used: DEX (Wako Pure Chemical Industries, Osaka, Japan), ethylene glycol tetraacetic acid (EGTA, Dojindo Laboratories, Kumamoto, Japan), xestospongin C (XestC, Wako Pure Chemical Industries), 1-(5-chloronaphthalenesulfonyl)homopiperazine hydrochloride hydrochloride (ML-9, TOCRIS Bioscience, Bristol, UK), YM58483 (also known as BTP2, TOCRIS Bioscience), and GLA (Sigma-Aldrich, St. Louis, MO, USA). All other reagents not described were of commercially available reagent grade.
Diet-induced AD model
Hairless mice were fed a special diet (HR-AD diet; Norsan, Yokohama, Japan) for more than 12 weeks to induce AD-like symptoms. Signs of disease were confirmed by gross observation and measurement of skin hydration and transepidermal water loss using Corneometer® CM825 and Tewameter® TM210 (Courage + Khazaka Electronic, Cologne, Germany), respectively. Mice fed a standard laboratory diet (MF; Oriental Yeast Industry, Tokyo, Japan) were used as normal control.
Skin Tslp mRNA expression
After the mice were euthanized by cervical dislocation, dorsal skin samples were collected and immersed in RNAlater® (Ambion, Austin, TX, USA) at 4°C for 24 h and then at –80°C until use. Total RNA was isolated using TriPureTM isolation reagent (Roche Diagnostics Japan, Tokyo, Japan) and TissueLyser LT (Qiagen, Hilden, Germany). The quality and quantity of the RNA were assessed by measuring the absorbance at 260 nm and 280 nm using Ratio Beam Spectrophotometer U-5100 (Hitachi, Tokyo, Japan). Reverse transcription was performed using the PrimeScriptTM reagent kit (Takara Bio, Otsu, Japan) and Takara polymerase chain reaction (PCR) thermal Cycler MP (Takara, Shiga, Japan). Real-time PCR amplification was performed using SYBR® Premix Ex TaqTM II (Takara Bio) with specific primers for β-actin (ID: MA050368) and TSLP (ID: MA080506) using Applied BiosystemsTM 7500 Real-time PCR System (Applied Biosystems, Foster City, CA, USA). The expression level of Tslp messenger ribonucleic acid (mRNA) was standardized to β-actin (Actb) mRNA.
Skin TSLP protein levels
Skin samples were homogenized in RIPA lysis buffer containing protease inhibitor cocktail (Roche, Basel, Switzerland). The resulting suspension was centrifuged at 18,000 × g for 10 min at 4°C, and the supernatant was collected and stored at –20°C until use. TSLP protein concentration was determined using Mouse TSLP Quantikine enzyme-linked immunosorbent assay (ELISA) Kit (R&D systems, Minneapolis, MN, USA) and MultiskanTM FC microplate reader (Thermo Fisher Scientific, Waltham, MA, USA). The TSLP concentration of each sample was standardized to the total protein content determined by Lowry’s method.
Isolation and culture of epidermal cells and measurement of TSLP mRNA and protein
After the mice were euthanized by cervical dislocation, the dorsal skin was removed and immersed in Dispase® II (Roche) for 18 h at 4°C. The epidermal sheet was separated and incubated in 0.25% trypsin (Gibco, Grand Island, NY, USA) for 1 h at 37°C to obtain single cells. Trypsin activity in the suspension was inactivated by adding fetal bovine serum (FBS; Nacalai Tesque, Kyoto, Japan). The cells were washed with phosphate-buffered saline, centrifuged, resuspended in Dulbecco’s modified Eagle medium (DMEM) containing FBS (except for experiments for GLA treatment), and incubated at 37°C for 0–24 h at a cell density of 5 × 105 cells/mL per well. The medium volume per well was 1 mL. For drug treatment, DEX (from 10–10 mol/L to 10–6 mol/L), EGTA (4 mmol/L), XestC (1 µmol/L), ML-9 (250 µmol/L), or BTP2 (250 µmol/L) was added in FBS-containing DMEM with or without 0.01% dimethyl sulfoxide (DMSO). GLA (from 10–10 mol/L to 10–4 mol/L) was added in 0.002% DMSO-containing FBS-free DMEM. After incubation, the cell pellets and supernatants were collected by centrifugation. RNA and protein were extracted from the cell pellets, and mRNA and intracellular protein levels of TSLP were determined as described above.
Statistical analysis
Data were analyzed using GraphPad Prism (version 8.0; GraphPad Software, San Diego, CA) and are represented as individual values and mean ± standard error (SE). for each group. Statistical differences were determined using an unpaired t test, one-way analysis of variance (ANOVA) with Dunnett’s multiple comparisons test, or two-way ANOVA with Bonferroni’s or Dunnett’s multiple comparisons test. Differences were considered significant at P < 0.05.
Results
Increase in TSLP expression in the skin of diet-induced AD model mice
As reported previously [24], special diet-fed hairless mice manifested AD-like symptoms, whereas mice fed a standard diet exhibited no gross abnormalities (Figure 1A). TSLP mRNA and protein levels were significantly increased in the skin of AD mice (Figure 1B and 1C).
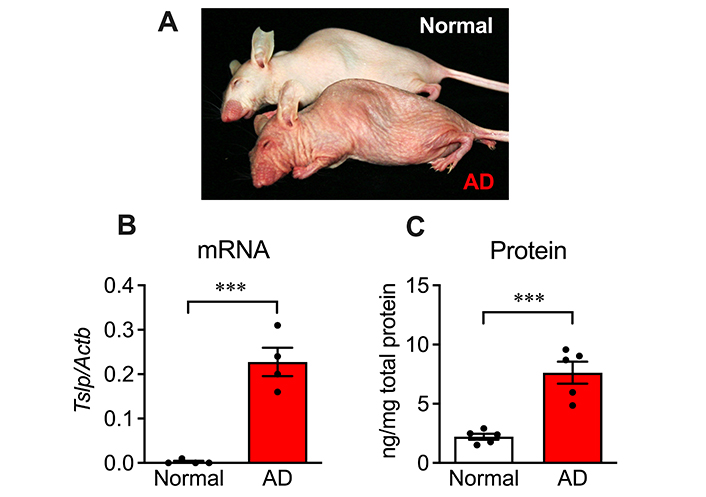
Increased TSLP production in diet-induced AD model mice. (A) Gross appearance of mouse fed with normal diet (back) or special diet (front) for 11 weeks. Special diet-fed mice exhibited AD-like symptoms; (B) TSLP mRNA level and (C) protein level in the skin of normal and AD mice. n = 4 (for normal) or 5 (for AD). *** P < 0.001, unpaired t test. Data represent individual values and mean ± SE
Spontaneous overproduction of TSLP in the epidermal cells of AD mice
Using the AD model, we investigated whether TSLP production capability is altered in epidermal cells. Epidermal cells were isolated from the skin of normal and AD mice and incubated under unstimulated culture conditions, and then mRNA and intracellular and extracellular protein levels of TSLP were determined (Figure 2A). In normal epidermal cells, Tslp mRNA levels gradually increased until 12 h and remained constant; however, TSLP protein was hardly detectable in both intracellular and extracellular fractions (Figure 2B and 2C). In contrast, in AD epidermal cells, Tslp mRNA expression was detected at the beginning of cultivation, peaked at 3 h, and then decreased drastically (Figure 2B). Intracellular TSLP protein was detected at the beginning of cultivation, and its levels increased transiently after 6 h (Figure 2C). Extracellular TSLP protein levels gradually increased and reached approximately 7,000 pg/mL per well at 24 h (Figure 2C). These results indicate that epidermal cells of diet-induced AD model mice spontaneously produce TSLP even under unstimulated conditions in vitro.
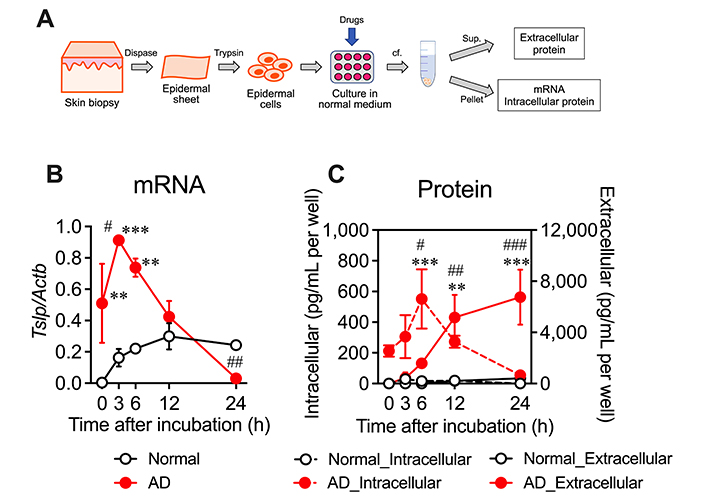
Spontaneous overproduction of TSLP in the epidermal cells of AD mice. (A) Protocol for determination of TSLP mRNA and protein levels in isolated and cultured epidermal cells. Epidermal cells were isolated using dispase and trypsin and incubated in 1 mL normal medium per well, and then mRNA and intracellular and extracellular protein levels of TSLP were determined; (B) mRNA and (C) intracellular and extracellular protein levels during 24-h cultivation. n = 5. ** P < 0.01 and *** P < 0.001 vs. normal. Two-way ANOVA with Bonferroni’s multiple comparisons test. # P < 0.05, ## P < 0.01, and ### P < 0.001 vs. 0 h. Two-way ANOVA with Dunnett’s multiple comparisons test. Data represent mean ± SE. sup.: supernatant; cf.: centrifugation
TSLP production in AD epidermal cells may be caused by STIM/Orai-mediated calcium entry
We pharmacologically examined the mechanism of TSLP production in AD epidermal cells. A glucocorticoid DEX (10–10–10–6 mol/L) did not significantly affect the increased TSLP mRNA and protein levels in AD epidermal cells (Figure 3A and 3B), suggesting that this TSLP production does not involve mechanisms that glucocorticoids can regulate.
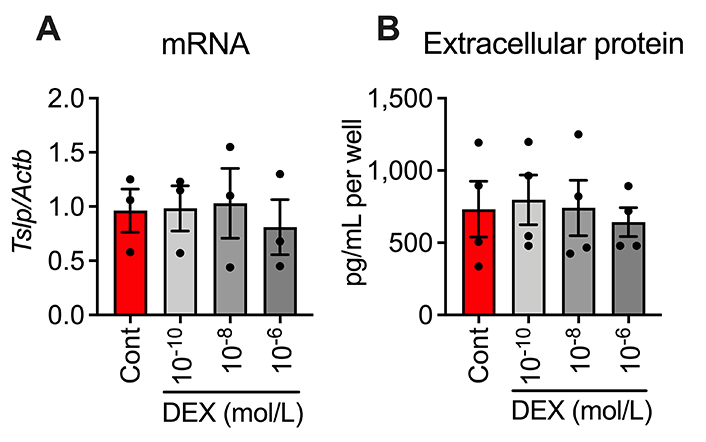
Effects of DEX on increased (A) TSLP mRNA level and (B) extracellular protein level in AD epidermal cells at 6 h after cultivation. n = 3 (for mRNA) or 4 (for extracellular protein). Data represent individual values and mean ± SE. Cont: control
The effect of a calcium chelator EGTA was investigated to clarify the involvement of extracellular calcium influx. Adding EGTA into the medium inhibited the increases in mRNA and intracellular and extracellular protein of TSLP by 59.9%, 76.3%, and 97.3%, respectively (Figure 4A–C). This result indicates that TSLP production in AD epidermal cells depends on extracellular calcium influx.
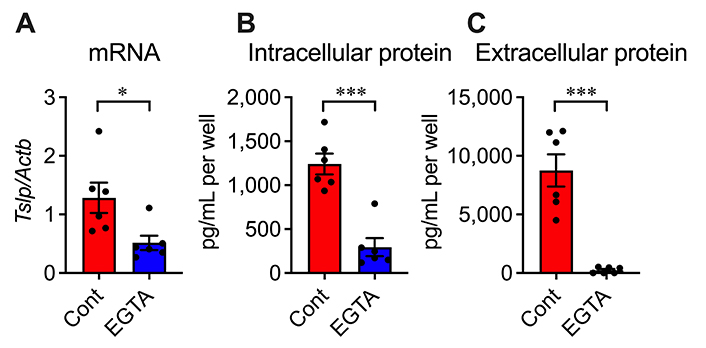
Effects of EGTA on TSLP overproduction in AD epidermal cells at 3, 6, and 24 h after cultivation, respectively. (A) TSLP mRNA level; (B) intracellular protein level; (C) extracellular protein level. n = 6. * P < 0.05; *** P < 0.001. Unpaired t test. Data represent individual values and mean ± SE
Therefore, we investigated the calcium influx pathway responsible for TSLP overproduction. Store-operated calcium entry (SOCE) is the major calcium influx mechanism in non-excitable cells, including epidermal keratinocytes [27–29]. A calcium sensor in the endoplasmic reticulum (ER), stromal interaction molecule 1 (STIM1), and a calcium release-activated calcium (CRAC) channel in the plasma membrane, Orai1, are essential components of SOCE [30–32]. Treatment with a STIM1 inhibitor ML-9 or a CRAC channel inhibitor BTP2 markedly suppressed the increased TSLP protein levels in the supernatant of AD epidermal cells (Figure 5). Inositol 1,4,5-trisphosphate (IP3) receptor-mediated calcium release from the ER triggers STIM/Orai-mediated SOCE [33, 34]. However, XestC, a selective inhibitor of IP3 receptors, did not significantly affect TSLP production in AD epidermal cells (Figure 5). These results suggest that STIM/Orai-mediated, but IP3 receptor-independent, calcium entry contributes to TSLP overproduction.
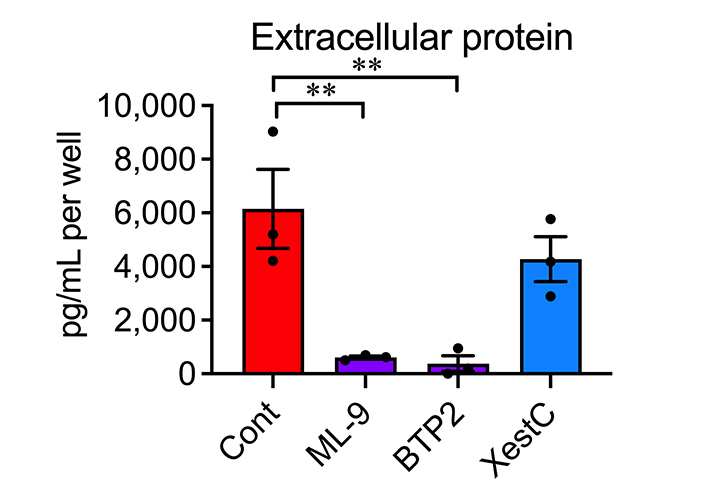
Effects of ML-9, BTP2 and XestC on TSLP overproduction in AD epidermal cells. Extracellular protein levels of TSLP at 24 h after cultivation were determined. n = 3. ** P < 0.01, one-way ANOVA with Dunnett’s multiple comparisons test. Data represent individual values and mean ± SE
GLA may negatively regulate TSLP production in AD epidermal cells
We examined whether a PUFA GLA affects TSLP production in AD epidermal cells. Because of the PUFA protein binding, cells were cultured in FBS-free DMEM. Even under this culture condition, TSLP mRNA and intracellular and extracellular proteins were increased (Figure 6A–D), although these changes were slightly blunted and variable than under FBS-containing conditions. A dose-response study showed that 10–4 mol/L of GLA significantly inhibited the increased TSLP production (Figure 6A) and suppressed the increased Tslp mRNA (Figure 6B). Moreover, GLA inhibited the increased intracellular and extracellular TSLP proteins; however, the differences were insignificant with the unpaired t test (Figure 6C and 6D). This result suggests that GLA negatively regulates TSLP production in AD epidermal cells.
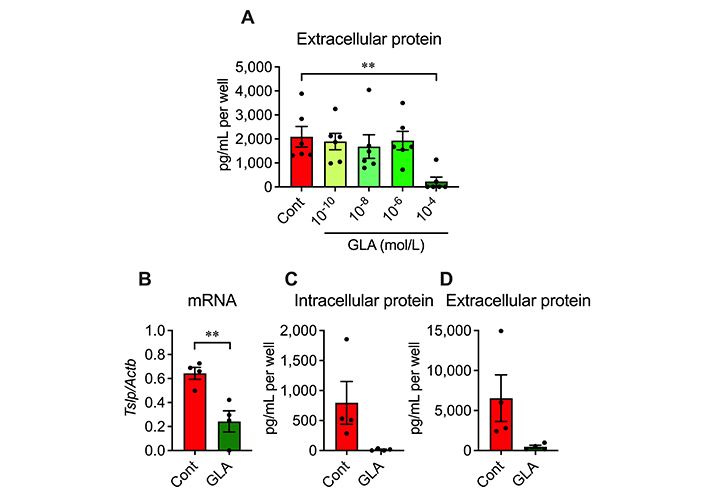
Effects of GLA on TSLP overproduction in AD epidermal cells. (A) Extracellular protein level of TSLP at 24 h after cultivation. n = 6. ** P < 0.01, one-way ANOVA with Dunnett’s multiple comparisons test; (B–D) TSLP mRNA and intracellular and extracellular protein levels at 3, 6 and 24 h after cultivation, respectively. (B) TSLP mRNA level; (C) intracellular protein level; (D) extracellular protein level. n = 4. ** P < 0.01, unpaired t test. Data represent individual values and mean ± SE
Discussion
In the present study, we used AD model mice to examine the production mechanism of TSLP in AD conditions. Epidermal cells were isolated from normal and AD mice, and their TSLP production capabilities were compared. Interestingly, even under unstimulated culture conditions, the Tslp mRNA level was significantly increased in the epidermal cells of AD mice, and a large amount of TSLP protein was extracellularly released. Such TSLP overproduction was not observed in the epidermal cells of normal mice (Figure 2). Thus, the present study reveals that the epidermal cells of our AD model mice can spontaneously produce TSLP in vitro.
We next pharmacologically examined the mechanism of TSLP production in AD epidermal cells. Glucocorticoids inhibit TSLP production via suppression of NF-κB signaling pathway. TSLP production induced by stimulation with TLR ligands, Th2 cytokines, or TNF-α is suppressed by glucocorticoids at concentrations higher than 10–10 mol/L [21, 35]. Moreover, glucocorticoids at concentrations less than 10–7 mol/L inhibit spontaneous TSLP production in KCMH-1 cells [23]. However, even at 10–6 mol/L, glucocorticoid DEX did not affect the increased expression and production of TSLP in AD epidermal cells (Figure 3). These findings suggest that the mechanism of TSLP production in AD epidermal cells is distinct from the well-studied NF-κB activation-mediated mechanism; however, to clarify whether NF-κB signaling is involved in this TSLP production, it is necessary to compare the degree of NF-κB activation and the effect of DEX on it between epidermal cells from normal and AD mice.
Alternatively, altered expression and activity of glucocorticoid receptors (GR) may contribute to steroid resistance to TSLP production in AD epidermal cells. The activity of GR is regulated by the balance of two isoforms, GRα and GRβ, GRα is the classic receptor responsible for glucocorticoid actions, whereas GRβ acts as a dominant negative isoform against GRα [36]. In the blood cells of patients with AD, GRα and GRβ are decreased and increased, respectively [37, 38]. Notably, TSLP expression is increased in the epidermis of keratinocyte-specific GR knockout mice [39].
TSLP expression and production in AD epidermal cells were almost completely blocked by extracellular calcium chelation (Figure 4), indicating the involvement of a calcium-dependent mechanism. Wilson et al. [11] demonstrated that PAR2-evoked TSLP production in keratinocytes is calcium-dependent: activating PAR2 triggers calcium release from IP3-sensitive stores and subsequent SOCE, leading to TSLP production. However, the mechanism underlying the activation of calcium signaling by the “unstimulated” AD epidermal cells remains unclear. In contrast, epidermal keratinocytes can sense extracellular calcium to initiate various cellular responses without stimuli. For example, in the epidermal differentiation process, although keratinocytes proliferate in low calcium concentrations (e.g., 0.03 mmol/L), the proliferation stops, and the differentiation program is initiated when the calcium concentrations increase to above 1 mmol/L [40]. Furthermore, G protein-coupled calcium-sensing receptors and E-cadherin-catenin complex play a role in calcium-induced epidermal differentiation [40]. The molecule and mechanism that trigger calcium signaling responsible for TSLP overproduction in AD epidermal cells require further investigation.
Like EGTA, ML-9 and BTP2 almost completely inhibited TSLP production in AD epidermal cells (Figure 5). ML-9 inhibits SOCE by preventing puncta formation and translocation of the ER calcium sensor STIM1 [41]. Although this compound is an inhibitor of myosin light chain kinase (MLCK), the inhibitory effect of ML-9 on SOCE appears unrelated to MLCK inhibition [41]. In contrast, BTP2 inhibits CRAC channel activity through Orai1 and Orai2 [42]. PAR2-evoked TSLP production in keratinocytes was blocked by small interfering RNA (siRNA) targeting STIM1 and Orai1 but not Orai2 [11]. These results indicate that STIM1- and Orai1-mediated calcium entry partially participates in TSLP production in AD epidermal cells. Moreover, BTP2 inhibits transient receptor potential canonical (TRPC) channels [43]. Thus, the role of Orai2 and TRPC channels in TSLP production in AD epidermal cells cannot be excluded.
A PUFA GLA also prevented TSLP production in AD epidermal cells (Figure 6), although the mechanism remains unclear. GLA is converted to dihomo-GLA (DGLA). Prostaglandin D1, a metabolite of DGLA, inhibits TSLP expression in keratinocytes stimulated by phorbol 12-myristate 13-acetate [44]. Therefore, some GLA metabolites may suppress TSLP production in AD epidermal cells.
Interestingly, arachidonic acid (AA), converted from DGLA, induces calcium entry through arachidonate-regulated calcium (ARC) channels comprising the Orai1 and Orai3 subunits [45, 46]. Furthermore, activation of ARC channels is store-independent but requires the minor pool of STIM1 located in the plasma membrane [47]. However, GLA does not inhibit calcium currents through ARC channels but slightly induces it in HEK293 cells [48]. However, the responsiveness of ARC channels to PUFA may be altered in “PUFA-deficient” and “diseased” epidermal cells derived from our AD model mice. We have shown that diet-induced AD is attributed mainly to PUFA deficiency [26]. In normal skin, topical AA induces inflammation, but oral [26] and topical (M. Fujii, unpublished observation) AA ameliorated skin inflammation in our AD model. Further studies are needed to determine whether ARC channels are involved in TSLP overproduction and its suppression by GLA in AD epidermal cells.
The limitation of the study is that the preparation of epidermal cells from model mice requires much time, and thus this assay is unsuitable for drug screening. Furthermore, it remains unclear whether the mechanism identified in this study applies to human AD. However, most studies on the mechanism of TSLP production have used normal cells or cell lines upon stimulation in vitro [11, 16–18, 23], and few have used primary cultured cells from diseased tissues. Therefore, our findings may help elucidate the intrinsic mechanism of TSLP production in human AD skin.
In conclusion, our study shows that epidermal cells isolated from our AD model mice can spontaneously produce TSLP in vitro. STIM/Orai-mediated calcium entry contributed to this TSLP overproduction. Given that in our model, AD was induced by PUFA deficiency, PUFAs, including GLA, may play a regulatory role in calcium signaling and TSLP production in AD conditions. Further elucidation of the mechanism underlying TSLP production in AD epidermal cells can help elucidate the pathogenic mechanism of AD and develop new therapeutic agents.
Abbreviations
AA: |
arachidonic acid |
AD: |
atopic dermatitis |
ARC: |
arachidonate-regulated calcium |
CRAC: |
calcium release-activated calcium |
DEX: |
dexamethasone |
DGLA: |
dihomo-γ-linolenic acid |
DMEM: |
Dulbecco’s modified Eagle medium |
EGTA: |
ethylene glycol tetraacetic acid |
ER: |
endoplasmic reticulum |
FBS: |
fetal bovine serum |
GLA: |
γ-linolenic acid |
GR: |
glucocorticoid receptors |
IL-4: |
interleukin-4 |
IP3: |
inositol 1,4,5-trisphosphate |
ML-9: |
1-(5-chloronaphthalenesulfonyl)homopiperazine hydrochloride hydrochloride |
mRNA: |
messenger ribonucleic acid |
NF-κB: |
nuclear factor-kappa B |
PAR2: |
protease-activated receptor 2 |
PCR: |
polymerase chain reaction |
PUFA: |
polyunsaturated fatty acid |
RXR: |
retinoid X receptor |
SE: |
standard error |
SOCE: |
store-operated calcium entry |
STIM1: |
stromal interaction molecule 1 |
Th2: |
T helper 2 |
TLR: |
Toll-like receptor |
TSLP: |
thymic stromal lymphopoietin |
TSLPR: |
thymic stromal lymphopoietin receptors |
XestC: |
xestospongin C |
Declarations
Acknowledgments
We thank Editage (www.editage.com) for English language editing.
Author contributions
MF: Conceptualization, Investigation, Writing—original draft, Writing—review & editing. SK, AU, MS, RM, and YY: Investigation, Writing—original draft. TN and SO: Writing—review & editing, Supervision. All authors read and approved the submitted version.
Conflicts of interest
The authors declare that they have no conflicts of interest.
Ethical approval
All animal studies were approved by the Ethics Committee of Animal Research of Kyoto Pharmaceutical University (approval number: 16-12-054) and performed following the guidelines for the proper conduct of animal experiments (Science Council of Japan, 2006).
Consent to participate
Not applicable.
Consent to publication
Not applicable.
Availability of data and materials
Not applicable.
Funding
This work was supported by JSPS KAKENHI [16K09000] and by Takeda Science Foundation. The funders had no role in study design, data collection and analysis, decision to publish, or preparation of the manuscript.
Copyright
© The Author(s) 2023.