Abstract
Mushrooms, due to their many medical, preventive, and nutraceutical purposes, as well as their reputation as a folk remedy, have long been an integral part of traditional cuisines. The therapeutic advantages of mushrooms may be attributed to their bioactive components, including polysaccharides (both low and high molecular weight), terpenoids, phenolic compounds, fatty acids, lectins, and glucans. The bioactive components have been discovered to possess various health advantages, including antibacterial, antifungal, anticancer, radical scavenging, cardiovascular, anti-hypercholesterolemia, and anti-diabetic effects. These effects have gained worldwide attention and stimulated interest in further investigating their potential applications. Functional foods have the dual purpose of serving as both nourishment and medication. They may assist in the management and prevention of health disorders that are not functioning optimally, as well as mitigate some adverse effects of life-threatening diseases. Further evaluation is necessary to fully understand the mechanisms via which mushrooms operate and improve their therapeutic properties. This review delves into the possible medicinal potential of mushrooms and the advantages they may provide to human health.
Keywords
Mushrooms, fungi, traditional medicine, nutraceuticalIntroduction
For a very long time, mushrooms have been a staple in human diets throughout the globe. They are also considered to be the primary untapped source of nutritional foods [1]. Traditionally recognized for their nutritional benefits, these substances are now gaining recognition for their significant therapeutic properties [2]. Thus, they find use as supplements, nutraceuticals, and mycotherapy products, in addition to being ingested as part of a normal diet. Although this technique is relatively new in the Western world, it has long been used in Asian countries to promote and maintain good physical well-being and treat ailments [3]. Medicinal mushrooms have a long history of beneficial health effects, and their current medicinal value is determined by the bioactive substances they contain (Figure 1). Edible mushrooms possess a highly sought-after combination of taste, scent, texture, and flavor. On the other hand, wild medicinal mushrooms have mostly been used in healthcare to address a range of health issues, from minor complaints to widespread diseases [4]. Nevertheless, it is difficult to categorize mushrooms as strictly edible or medical because some commonly consumed species possess therapeutic qualities, while other mushrooms utilized for medicinal reasons are also suitable for consumption [5]. Even though there are more than 2,000 kinds of mushrooms in the world, only a few of them are fit for human consumption or have nutritional value. The most commonly cultivated mushroom species is Agaricus bisporus, followed by Lentinus edodes and Flammulina velutipes [6]. Among the many metabolites found in mushrooms are steroids, phenolic acid, benzoic acid, anthraquinone, and terpenes. Meanwhile, the main metabolites consist of proteins, oxalic acid, and peptides [7]. In terms of nutrition, they have a high protein and amino acid content but are deficient in fatty acids [8]. Nevertheless, they contain a substantial quantity of vitamins (B1, B2, B12, C, D, and E), iron, phosphorus, copper, iodine, selenium, zinc, and ergosterol [9]. Therefore, they serve as an ideal source of current nourishment and enhance health via the combined benefits of bioactive substances [10–13]. These components control the development, balance of fluids, and health of bones in humans, and also act as effective nutraceuticals to enhance immune responses.
We may strengthen our immune system and protect ourselves from a host of diseases by making mushrooms a regular part of our diet. Mushrooms, like other fruits and vegetables, are inherently gluten-free and may be a tasty and healthy component of a gluten-free eating plan. β-glucans, which are present in several types of mushrooms, have significant immune-stimulating properties, enhance resistance to allergens, and may even have a role in the metabolic processes of fats and carbohydrates in the human body. The β-glucans found in oysters, shiitake, and split gill mushrooms are often regarded as the most potent [14, 15]. Mushrooms are a rich source of B vitamins which show a significant effect on the neurological system [16, 17].
Presently, there is a substantial study being conducted on the discovery, isolation, and categorization of the key bioactive polysaccharides found in mushrooms. Extracting useful compounds from mushrooms now makes use of a broad variety of both conventional and cutting-edge extraction techniques. Integrated extraction, subcritical water, enzyme-assisted, microwave-assisted, and pulsed electric field-assisted extraction are some of the novel methods for extracting bioactive components [18]. Medicinal mushrooms possess a wide range of pharmacological effects, including anti-inflammatory, anti-microbial, anti-oxidant, anti-cancer, anti-diabetic, immunomodulatory, anti-allergic, hepatoprotective, anti-hyperlipidemic, and prebiotic properties, etc. [19, 20] (Figure 2). The therapeutic effects of a medical fungus are largely identified by in vitro tests, often followed by in vivo experiments on animal models. These investigations together demonstrate the significant potential of mushrooms, fungal extracts, or chemical compounds [21, 22]. The many activities mentioned are caused by several bioactive metabolites found in both the mycelium and, more importantly, the fruiting body [23]. The biological impact of these metabolites depends on their chemical composition, while their distribution is determined by the specific fungus species. Multiple studies have extensively examined the diverse functions of medicinal mushrooms, emphasizing their significant potential for application in the medical field. However, a specific focus has been placed on investigating their antitumor and immunomodulatory characteristics, as cancer continues to pose a formidable obstacle [24]. The multifaceted anticancer properties of mushrooms mostly stem from the diverse groupings of distinctive chemicals that engage with malignant tissues and cells via a broad spectrum of biological mechanisms, yielding therapeutic outcomes [25]. Different varieties of mushrooms displaying anticancer profiles are shown in Figure 3 and clinical studies data of some anticancer mushrooms is discussed in Table 1 [26–31]. Analyzing the metabolism of cancer-prone cells is a common way to investigate the properties of employing bioactives derived from fungus for tumor therapy [32].
Clinical studies data on various mushrooms showing anticancer potential
S. No. | Mushroom | Cancer | Phase | Bioactive components | Result |
---|---|---|---|---|---|
1 | Agaricus bisporus | Breast cancer | Phase I completed | Polysaccharides, lectin | Reduced cancer by bringing down immunosuppressive factors. |
Prostate cancer | Phase Ib completed, n = 32 | Polysaccharides, lectin | |||
2 | Agaricus blazei | Multiple myeloma | Phase II completed, randomized clinical trial, n = 100 | Genistein | Patients with cervical, ovarian, or endometrial cancer who were having chemotherapy did not vary significantly from those who were not treated with regard to lymphokine-activated killer or monocyte activity. Furthermore, verum only showed improvement in a number of negative effects when combined with mushroom extract. |
3 | Lentinula edodes | Prostate cancer | Phase II completed, n = 74 | CombinedPolysaccharide(GCP) | In individuals with early-stage prostate cancer, mushroom extract did not lower prostate specific antigen by more than 50%. |
Hepatocellular carcinoma and hepatitis B & C | Phase II completed | Arabinoxylan | -- | ||
4 | Grifola frondosa | Breast and lung cancer | Phase I completed, n = 34 | Polysaccharides | In peripheral blood samples taken from postmenopausal breast cancer patients, maitake extracts had an effect on immunological stimulatory and inhibitory characteristics. |
5 | Omphalotus illudens | Thyroid cancer, metastatic or recurrent gastric cancer, recurrent or persistent epithelial ovarian cancer | Phase II completed | Illudin S semisynthetic derivative | -- |
6 | Trametes versicolor | Gastric cancer | Controlled trial, n = 60 | Krestin, PSK, PSP | Chemotherapy alleviated Qi and Yin deficit symptoms in gastric cancer patients. |
7 | Agaricus sylvaticus | Breast cancer | Randomized clinical trial, n = 46 | -- | Enhanced nutritional status with decreased side effects in stage II & III breast cancer patients, including nausea, vomiting, and anorexia. |
--: no description
The immune response was stimulated by polysaccharides isolated from Phellinus linteus, resulting in the suppression of tumor growth and pulmonary metastasis. These polysaccharides were found to be non-toxic to cancer cells. Studies have found that compounds derived from Ganoderma lucidum have demonstrated potential in fighting against cancer [33]. The β-D-glucans from Ganoderma lucidum have been found to have a significant impact on cancer cells, inhibiting their growth. Additionally, they have been shown to protect normal cells from free radicals and reduce damage to these cells [34]. The inclusion of vital and additional amino acids, along with organic substances including ectin, adustin, ribonuclease, and nicotine, has garnered interest in using mushrooms and their extract for cancer treatment [35–37].
Mushrooms were also found to produce various ribotoxic proteins as a defensive strategy. These proteins, which inhibit protein synthesis, include ribonucleases, ribotoxins, ribotoxin-like proteins (RL-Ps), and N-glycosylases. The therapeutic function of these enzymes is still being debated, despite their widespread distribution. They operate on the GAGA tetraloop at the level of the bigger rRNA’s single-stranded sarcin ricin loop (SRL), and the majority of them are site-specific enzymes that permanently change the same target, the ribosome. When ribosome interacts with eukaryotic or prokaryotic elongation factors (EF-G or EF-2), this loop stops the translation-inhibiting mRNA-tRNA translocation, which leads to cell death by apoptosis [38, 39]. Finding a diagnostic RNS fragment unconfined from the rRNA SRL loop may indicate when ribotoxic proteins modify the binding site of translocation factors on ribosomes. To be more precise, ribotoxins produced by ascomycetes fungi and RL-Ps isolated from edible mushrooms are precise ribonucleases that allow the hydrolysis of one phosphodiester bond in SRL (rat 28S rRNA numbering) [40–42]. This process releases a particular rRNA 3’-end fragment known as the α-fragment. Despite sharing a common target (the SRL loop), the catalytic mechanism is distinct in ribotoxins and RL-Ps, which are structurally distinct low molecular weight proteins (ranging from 13 kDa to 16 kDa). Two histidinyl and one glutamyl, specifically His50, His137, and Glu96 are amino acid residues needed for ribotoxin activity, according to the α-sarcin numbering system [43]. In contrast, RL-Ps have two aspartyl and one histidinyl, specifically Asp68, Asp70, and His77 according to the ageritin numbering system. The ribosome inactivating proteins (RIPs) are hydrolases that operate as glycosylases and hydrolyze N-glycosyl compounds. They are rRNA N-glycosylases (EC 3.2.2.22), which means they are also hydrolases (EC 3). Adenine (A4324, rat 28S rRNA numbering) was removed and an abasic (i.e., deadenylated) site was formed as a result of site-specific rRNA N-glycosylase activity towards ribosomes. The β-fragment is the result of the cleavage of the rRNA 3’-end fragment, which may happen as a result of acid aniline RNA treatment, which makes this site unstable [44, 45]. When Endo and Tsurugi [46] examined the effects of ricin on rat eukaryotic 28S rRNA, they were the first to record the rRNA N-glycosylase activity of plant RIPs. Various RIP that are able to release the β-fragment include calcaelin from Calvatia caelata [47], lyophyllin from Lyophyllum shimeji [48], marmorin from Hypsizygus marmoreus [49], mucoricin from Rhizopus delemar [50], volvarin from Volvariella valvacea [51] whereas RIP that are improperly classified as N-glycosylases includes bolesatine from Boletus satanas [52], flammin from Flammulina velutipes [53], flammulin from Flammulina velutipes [54], hypsin from Hypsizygus marmoreus [55], pleuturegin from Pleurotus tuber-regium [56], tricholin from Trichoderma viride [57], velin from Flammulina velutipes [53], and velutin from Flammulina velutipes [58].
Furthermore, the enhanced understanding of the molecular mechanisms underlying the development of tumors and their spread has opened up possibilities for the identification of novel therapeutic agents targeting aberrant molecular and biochemical pathways implicated in cancer [59]. Focusing on the anticancer effects of various mushroom types, this study seeks to provide a comprehensive overview of the many medicinal uses of mushrooms and their bioactive components.
Significance of bioactive compounds showing anticancer potential
Polysaccharides, proteins, lipids, phenolics, alkaloids, enzymes, folate, selenium, and organic acids are just a few of the bioactive substances found in mushrooms. The mushrooms contain various active chemical moieties that have been found to have potential anticancer properties which include lanosterol, ergosterol, ergosterol peroxide, lanostatriene diol, inotodiol, trametelonic acid, antroquinonol, cordycepin, hispolon, lectin, krestin, polysaccharide, sulfated polysaccharide, lentinan [60] (Figure 4). Mushroom polysaccharides are powerful chemicals with amazing capabilities, including anticancer and immunomodulatory actions. β-glucan is a kind of polysaccharide that typically has a glucose backbone connected by β-(1,3)-glycosidic bonds and maybe extra glucose residues linked by β-(1,6) connections. The generation of cytokines by β-glucan activates phagocytes and leukocytes, which in turn boost the immune system. Lentinan and lectins from Lentinula edodes have been shown to have cytotoxic effects on breast cancer cells in research [61]. Prescription anticancer drugs in Japan have been approved, including lentinan, schizophyllan, and krestin. Mushroom polysaccharides have been found to enhance the activity of natural killer cells, T cells, B cells, and macrophages, resulting in a heightened immune response [62, 63]. Cordycepin, also referred to as 3-deoxyadenosine, is a significant compound with potent anticancer properties found in Cordyceps species and works by inducing apoptosis through disrupted polyadenylation and binding to the nucleic acid binding site, resulting in the termination of DNA or RNA elongation. Studies have shown that hispolon, a polyphenol molecule, may enhance the efficacy of chemotherapy medications and has powerful anti-cancer characteristics [64, 65].
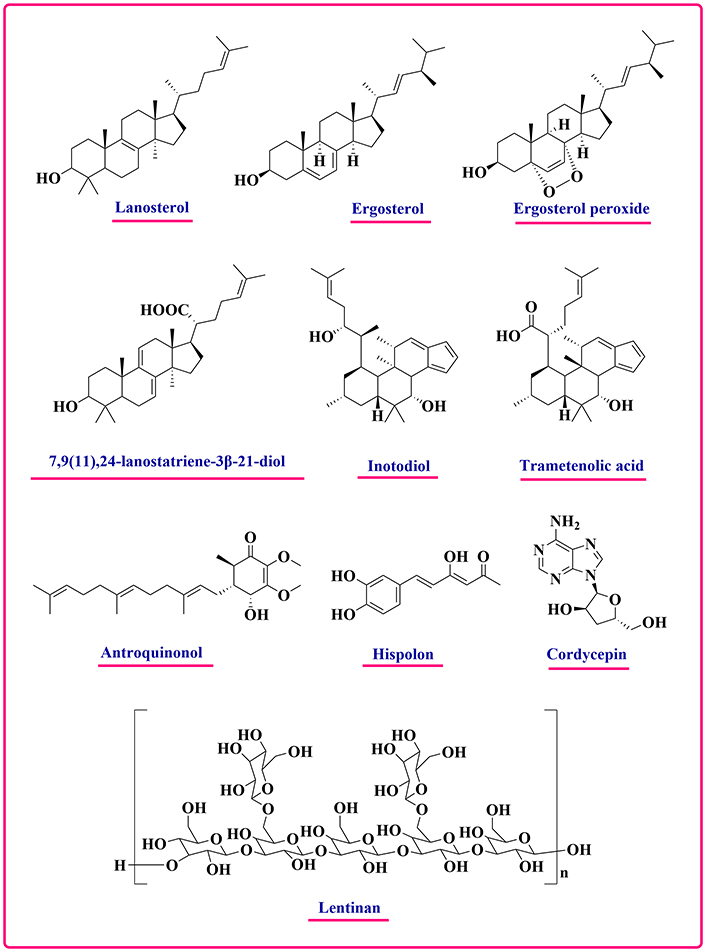
Chemical structures of some important bioactive constituents in mushrooms showing anticancer potential [2, 60]
Note. Adapted from “Narrative review: bioactive potential of various mushrooms as the treasure of versatile therapeutic natural product” by Chopra H, Mishra AK, Baig AA, Mohanta TK, Mohanta YK, Baek KH. J Fungi (Basel). 2021;7:728 (https://www.mdpi.com/2309-608X/7/9/728). CC BY.
Mechanism of action
Despite the lengthy history of mushroom use as a medicine in Asia, its global popularity for the treatment of many diseases, including cancer, has only recently grown in the last few decades. Mushrooms’ tremendous culinary and medicinal potential is due to the bioactive substances they contain. To improve cancer therapy success rates, it is essential to understand the molecular pathways that drive cancer initiation and development and the molecular targets of bioactive compounds generated by mushrooms. All the pathways that have been targeted for curing cancer or overcoming multidrug resistance are highlighted in Figure 5.
Anticancer role of mushrooms
Boobalan et al. [66] developed carbon dots using oyster mushrooms as a source. One possible use for them is as colorimetric sensors to detect Pb2+ ions. The C-dots may be used as a fluorescent probe to detect DNA by taking advantage of the electrostatic interaction between ctDNA and the dots. When tested on MDA-MB-231 breast cancer cells, the dots demonstrated anticancer properties. Cell blebbing and chromatin condensation were two of the morphological changes brought about by the addition of C-dots. The nuclear area division was confirmed by the Hoechst 33342 staining of cancer cells [66]. Water-soluble polysaccharides were used by Zeng et al. [67] to embellish the selenium nanoparticles, which were then extracted from the mushroom fungus. Despite the passage of thirteen weeks, the nanoparticles remained stable, with their particle size remaining within the range of 91 nm to 102 nm. There was a greater degree of vulnerability to nanoparticles shown by the cells that were found in the stomach cancer. The study showed that nanoparticles triggered apoptosis via the activation of caspase and mitochondria-mediated pathways [67]. In their work, Shomali et al. [68] used the MTT assay to assess how the ethanol extract of Marasmius oreades affected HT-29, MCF-7, and MDA-MB-231 cells. According to Zeng and Zhu’s 2018 research [69], HLP-1-1 and HLP-2-1 derived from Helvella leucopus shown anti-cancer characteristics when tested against HepG2 cells. A human colon cancer cell line, Caco-2, has been demonstrated to be susceptible to the secondary metabolites of Ganoderma applanatum. Metabolites increased glutathione levels and caused structural and cosmetic alterations. Metabolite treatment was also associated with a dramatic increase in the Bax/Bcl-2 ratio. The size of the solid Ehrlich tumor was shown to reduce after 5 days of exposure to metabolites, according to Elkhateeb et al. [70].
Kosanić et al. [71] examined how varying metal concentrations impact the anticancer properties of Lactarius deliciosus and Macrolepiota procera. A545, LS174, and HeLa cell lines were used to assess the anticancer activity. Results indicated that Macrolepiota procera was much more effective against cancer in the A549 and LS174 cell lines, but HeLa cells were more vulnerable to Lactarius deliciosus [71]. The Chaga mushroom (Inonotus obliquus) contains bioactive compounds such as lanosterol, 7,9(11),24-lanostatriene-3β-21-diol, ergosterol, inotodiol, ergosterol peroxide, and trametenolic acid (Figure 4). Using the prostate cancer cell line PC3 and the breast cancer cell line MDA-MB-231, these compounds have shown anticancer capabilities. The IC50 values for ergosterol (9.82 ± 0.98 µg/mL), ergosterol peroxide (38.19 ± 1.67 µg/mL), trametenolic acid (63.71 ± 3.31 µg/mL), and 7,9(11),24-lanostatriene-3β-21-diol (73.46 ± 0.64 µg/mL) were found to be against PC3. However, the IC50 values for inotodiol and lanosterol were more than 100 μg/mL, indicating that they were ineffective [25, 72, 73]. Kim et al. [74] extracted the heteropolysaccharides from Lactarius deliciosus, which showed anticancer potential. Shin et al. [75] investigated the correlation between the consumption of mushrooms and the reduction of breast cancer risk in a sample of 358 female participants with breast cancer and 360 cancer-free women (control group) in Korea. The study indicated that a greater intake of mushrooms was associated with a lower risk of breast cancer, particularly among premenopausal women. It was observed that this connection may be stronger in women with hormone receptor-positive tumors [75]. Park et al. [76] documented the extraction of proteins from the Cordyceps militaris (CMP). The proteins that were separated and kept apart exhibited enzymatic activity similar to that of trypsin, a kind of serine protease. The protein can hinder the growth of Fusarium oxysporum and also has a harmful effect on human breast and bladder cancer cells [76]. Niu et al. [77], observed a polysaccharide derived from Agaricus blazei inhibited the formation of new blood vessels in living organisms, thereby demonstrating the ability of polysaccharides obtained from edible mushrooms to prevent the development of cancer [77]. Baker et al. [78] documented the presence of anti-tumor, immune-modulating, and anti-metastasis characteristics in Phellinus linteus. The inonotsuoxides A, inotodiol, trametenolic acid, and lanosterol were successfully isolated from the mushrooms by Nakata et al. [79], who demonstrated their anticancer effectiveness in vivo. Mushrooms with therapeutic properties, namely Pleurotus pulmonaris, Phellinus rimosus, Pleurotus florida, and Ganoderma lucidum, possessed significant antioxidant and anti-tumor effects. According to Ajith and Janardhanan [80], these sources are known to include beneficial substances that may fight against tumors and serve as antioxidants. They also have the ability to prevent the development of cancer and mutations [80]. The polysaccharide extract from Pleurotus ostreatus, when dissolved in water, has been shown to have properties that promote cell death (apoptosis) and inhibit cell growth (proliferation) in HT-29 cells of colon cancer, as reported by Lavi et al. in 2006 [81]. Button mushrooms (Agaricus bisporus L.) have been shown to possess significant promise in the reduction of breast cancer. This is due to their ability to decrease aromatase activity and the production of estrogen, as demonstrated by both in vivo and in vitro investigations [82].
Oxidative stress and antioxidant potential of mushrooms
An imbalance between reactive oxygen species (ROS) and antioxidant defenses generated by living organisms leads to oxidative stress [83–85]. Superoxide anion, H2O2, and OH radical are examples of ROS. Many believe that the damage that ROS due to DNA, proteins, and lipids is a major cause of aging and worsened aging illnesses [86–88]. A wide range of devastating human illnesses, including Alzheimer’s, Parkinson’s, cancer, neurological disorders, and metabolic disorders, may develop in response to elevated levels of oxidative stress. When antioxidant levels inside the body are inadequate, it may be essential to consume more antioxidants from outside sources to alleviate oxidative stress [89, 90]. Numerous mushrooms displaying antioxidant potential are discussed in Figure 6 [91] whereas various mechanisms for the antioxidant potential of mushrooms are as mentioned in Figure 7 [92]. Several mushrooms have had their fruiting bodies, mycelium, and broth isolated for their antioxidant components [85].
Ascorbic acid, carotenoids, ergothioneine, flavonoids, glycosides, phenolics, polysaccharides, and tocopherols are some of the mushroom components that are known to have high antioxidant capabilities [92–97]. The antioxidant chemicals found in mushrooms have been studied and measured using a variety of spectrophotometric assays such as UV-Vis spectroscopy, FT-IR, NMR, HPLC, GC, and other techniques [98–103]. Everyone knows that mushrooms have antioxidant properties. The electron spin resonance, erythrocyte hemolysis, chelation of ferrous and cupric ions, methods based on the transfer of electrons and hydrogen atoms, and monitoring the activity of catalase, glutathione peroxidase, and superoxide dismutase are some of the ways that mushroom extracts’ antioxidant activity is measured. There is evidence that rufoolivacin, rufoolivacin C, rufoolivacin D, and leucorufoolivacin may scavenge DPPH radicals. The phenolics included in Ramaria flava help neutralize DPPH and OH free radicals [104–108].
Future prospects
To understand cancer physiology, several screening and diagnostic methodologies have been applied, while plentiful tools and techniques have been used to extract bioactive components from natural sources. However, researchers and even pharma giants are hesitant to investigate treatments based on natural products because of the complex development and validation procedures and higher failure rates during the translational phase (90% failure rates) [109]. Aspects contributing to failures are toxicity, pharmacokinetics, and transportation issues. As a result, strong empirical evidence during the preclinical phase is required. Available clinical research data across the globe indicate that mushrooms can regulate the growth/proliferation of cancerous cells and might potentially be used for therapeutic purposes. Crude extracts of mushrooms are seldom used in conventional/traditional medicine because of their intricate nature and ambiguous mode of action, due to which it is very difficult to identify the active chemical moiety responsible for the desired anticancer potential [110]. To resolve such cases, bioassay-guided purification can be an important tool to isolate the molecules accountable for the anticancer properties that should be beneficial in generating promising therapeutic candidates. Furthermore, the attainment of the goals of possible anticancer drug discovery necessitates the presence of more compelling experimental therapeutic data and ongoing endeavors. Bioinformatic techniques may be sound and effective in the process of identifying potential and safe anticancer drugs. Although this will help standardize treatment, complications may arise from interactions among different bioactive components [111, 112].
Conclusions
By stimulating and/or controlling the immune system, bioactive compounds in mushrooms may limit the metastasis and progression of cancer cells. This is achieved via influencing the maturation, differentiation, and proliferation of immune cells. If anticancer and antioxidant molecules produced from mushrooms are to effectively inhibit cancer and enhance the quality of life for cancer patients, it is crucial to understand the processes by which they work. There has been a dearth of clinical research investigating the potential advantages of medicinal mushrooms in immunomodulation, anticancer activity, and minimizing the adverse effects of conventional therapies. Therefore, larger sample sizes, more traditional mushroom preparations, and longer follow-ups are all necessary for more refined clinical trials on mushrooms that may have anticancer benefits. In addition, additional studies should look at the possibility of medicinal mushrooms as a cancer preventive when included in a balanced diet.
Abbreviations
RIPs: | ribosome inactivating proteins |
RL-Ps: | ribotoxin-like proteins |
ROS: | reactive oxygen species |
SRL: | sarcin ricin loop |
Declarations
Author contributions
MJN: Conceptualization, Writing—original draft, Writing—review & editing.
Conflicts of interest
The author declares no conflicts of interest.
Ethical approval
Not applicable.
Consent to participate
Not applicable.
Consent to publication
Not applicable.
Availability of data and materials
Not applicable.
Funding
Not applicable.
Copyright
© The Author(s) 2024.