Abstract
This article provides an informative overview of the current situation and future trends in cervical cancer prevention. Cervical cancer remains a significant public health concern worldwide and is characterized by notable variations in both incidence and mortality rates between developed and developing countries. This underscores the importance of understanding the pathophysiology of cervical cancer, stressing the involvement of high-risk HPV types. The presence of supplementary risk factors facilitates the transition from infection to cancer. This review examines current preventive methods, including the success of HPV vaccines such as Gardasil and Cervarix, and the effectiveness of screening techniques, from cytology to HPV DNA testing. It noted the limitations faced by primary and secondary preventive measures, particularly in low-resource settings, which include access to vaccines and effective screening procedures. Emerging technologies in cervical cancer prevention, such as liquid-based cytology, molecular testing, and AI, promise to improve early detection and diagnosis accuracy and efficiency. The potential of precision medicine to customize treatment based on individual risk factors was discussed. It explores the innovation in genetic editing techniques, such as CRISPR/Cas9, in targeting HPV oncoproteins, the advent of immunotherapy, the role of tumor-infiltrating lymphocytes, and the prospects of biomarkers in improving early detection. Research and technological advancements are leading to transformative changes in cervical cancer prevention. These developments suggest a path toward improved screening, diagnosis, and treatment that could significantly reduce the global burden of the disease. However, realizing the full potential of these advances requires inclusive research and international collaboration to overcome access disparities, particularly in resource-limited settings.
Keywords
Cervical cancer, prevention, technological advances, prospectsIntroduction
Cervical cancer is the second most prevalent cancer among women worldwide, accounting for 20% of the overall cancer burden. In 2023, Cancer.Net® estimated 13,960 incident cases and 4,310 deaths among women from the disease [1]. By 2026, it is estimated that less developed nations will experience an increase of 528,000 new cases of cervical cancer, with emerging countries accounting for 85% of these instances. Furthermore, cervical cancer leads to approximately 266,000 annual fatalities, accounting for 8% of all cancer-related deaths [2]. In the United States, approximately 11,500 new cases of cervical cancer are diagnosed each year, leading to the unfortunate deaths of 4,000 women due to the illness [3]. However, developing countries have notably decreased the occurrence of cervical cancer by implementing cervical cancer screening programs [4]. Efforts to prevent cervical cancer, led by voluntary organizations and government initiatives, have been ongoing for many years, but their effectiveness has been limited. Initially centered on screening and treating precancerous lesions, recent progress has encompassed human papillomavirus (HPV) vaccination. In India, approximately 272.8 million women are eligible for cervical cancer screening, while 59.7 million girls are eligible for vaccination against the disease [5]. Cervical cancer can be caused by various factors, including HPV, smoking, multiple sexual partners, prolonged use of birth control tablets, and early initiation of sexual activity [6]. It is believed that the HPV virus contains nonenveloped DNA. A virus family spanning eight kilobases has been associated with diverse proliferative diseases that affect epithelial cells [7]. More than 200 HPV genotypes have been identified, with crucial implications for public health. The high-risk HPV genotypes, HPV 16, 18, 52, 56, 58, and 59, in particular, have a strong association with cervical cancer, making them highly significant. Of these, genotypes 16 and 18 account for approximately 70% of cervical cancer cases worldwide [8]. Notably, in developed nations, the effective adoption of cytology-based screening, HPV vaccination campaigns, and systematic HPV screening has dramatically lowered the incidence of cervical cancer. WHO’s plan to eradicate cervical cancer worldwide by 2130 was introduced in 2020 to achieve an annual incidence rate of fewer than 4 per 100,000 women worldwide [9]. The three main goals of the strategy are to vaccinate 90% of girls before the age of 15, to screen 70% of women between the ages of 35 and 45 with an HPV-based test, and to guarantee that 90% of women with cervical dysplasia receive proper care from qualified professionals [10]. This review provides an updated overview of the current status and new emerging prevention measures for cervical cancer.
Pathophysiology of cervical cancer
Understanding the pathology of cervical cancer requires recognizing that while infection with high-risk HPV is a crucial factor, it alone is insufficient for the development of the disease. Cervical cancer is broadly classified into two types based on the cell type: squamous cell carcinoma, representing 80–90% of cases, and adenocarcinoma [11]. Adenocarcinomas form in the glandular cells of the upper part of the cervix (endocervix), whereas squamous cell carcinoma begins in the cells covering the lower part of the cervix (ectocervix). From a morphological perspective, it is well understood that normal cervical tissue gives way to precancerous stages, including mild [cervical intraepithelial neoplasia grade (CIN1)], moderate (CIN2), and severe neoplastic (CIN3) lesions, and finally, squamous cell cervical cancer. Differentiating between lesions that do not progress (productive lesions) and those that are CIN2+ and have a higher risk of developing cervical cancer can be complex. Various molecular biomarkers, including specific proteins, DNA methylation patterns, and microRNAs (miRNAs), are currently being investigated for their potential to differentiate between these lesions and monitor women with CIN2+ lesions [12], as depicted in Figure 1.
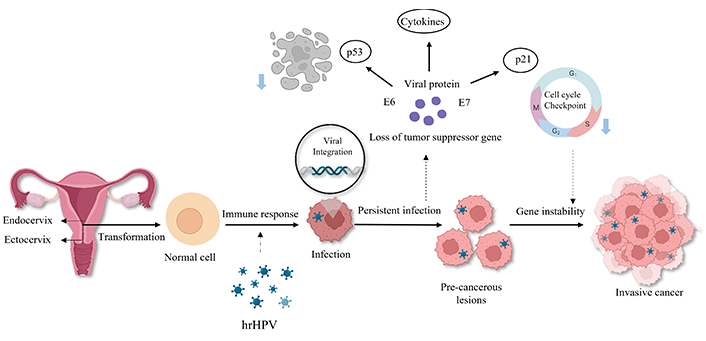
Pathophysiology of cervical cancer. In the regular cells, when affected by the action of high-risk HPV (hrHPV), precancerous cells are formed, which reduces gene stability, leading to the cause of cervical cancer. Created with BioRender.com
Some low-risk HPV types, particularly types 6 and 11, lead to the development of anogenital warts, known as condylomata acuminata. While there are over half a million identified cases of HPV annually, the majority are low-grade infections that typically resolve spontaneously within two years. High-grade lesions and cancers develop further in the presence of additional carcinogenic risk factors. Within HPV DNA, oncoproteins E6 and E7 disrupt the essential host cell cycle; E6 hinders the tumor suppressor protein p53, while E7 disrupts retinoblastoma protein (pRB) function. Additionally, the E5 protein may contribute to immune evasion. These factors play a significant role in HPV-related neoplasia, including in primary vaginal cancer. Oxidative stress and miRNAs are believed to be involved in cervical carcinogenesis. Further research is necessary to understand these factors’ interactions [13].
Current methods for preventing cervical cancer
According to recommendations from the WHO and other guidelines, HPV testing, particularly the Pap test, is considered a secondary procedure. If positive or abnormal results occur, the subsequent HPV DNA test procedure will include colposcopy and a related biopsy [14]. When there is a discordance between HPV test results and cytological examination findings, it is advisable to conduct a repeat HPV DNA test after one year, with subsequent tests recommended at 12–24 months. Additionally, for histological diagnoses of CIN2, patients should be presented with surgical options, followed by targeted ongoing monitoring [15]. In developed nations, cervical cancer screening and detection programs rely on cytology-based services, particularly Pap smears. The implementation of organized cytology-based cervical screening programs in Europe, North America, Australia, and Japan has played a pivotal role in significantly reducing the occurrence of cervical cancer in these regions over the past five decades [16]. According to the numerical model established by strategy developers, adopting and implementing the proposed approach is anticipated to lead to a 42% reduction in the median incidence rate by 2045 and an even more substantial decline of 97% by the year 2120 [17].
Primary prevention
Primary prevention includes providing information on safe sexual behaviours and promoting vaccination against HPV [18]. The initially licensed vaccine, Gardasil by Merck in Pennsylvania, was introduced in 2006 to protect against HPV types 6, 11, 16, and 18. Another vaccine, Cervarix, licensed in 2009 by GlaxoSmithKline in Belgium, focuses on HPV types 16 and 18. These vaccines have been implemented in over 80 countries and have demonstrated short-term safety, immunogenicity, and effectiveness in preventing HPV infections and associated precancerous lesions. Empirical data indicate that therapeutic vaccines may cross-protect against closely related HPV varieties. Even though it is less successful in treating chronic illnesses, Australia’s national HPV vaccination program shows significant promise in preventing high-grade cervical abnormalities, as seen by a decline in these conditions within three years of its introduction [19]. The current information suggests that the effectiveness of the two vaccine doses may be comparable to that of the three vaccines. This finding has significant implications for regions where vaccine utilization is constrained by financial considerations [20]. Therapeutic vaccines, which go beyond prophylactic vaccines, are designed to eradicate HPV infections by triggering T-cell-mediated immunity against the E6 and E7 antigens of the virus. In the clinical trials, these vaccinations have proven effective [21].
Secondary prevention
Secondary prevention is the process of identifying precancerous lesions before they become cancerous by screening asymptomatic individuals or by performing definitive tests in symptomatic or screen-positive patients. Numerous options are available for cervical cancer screening. According to observational studies, implementation of a routine cervical cancer screening program has been shown to reduce the incidence of invasive cervical cancer and cancer-related deaths. Several methods used for screening preinvasive diseases include (1) cervical cytology, including both liquid-based and conventional approaches, (2) direct visual inspection, (3) visual inspection with acetic acid, (4) visual inspection with acetic acid, (5) visual inspection with Lugol’s iodine, (6) HPV DNA testing, (7) speculoscopy, and (8) polar probes [22]. Other screening methods are typically employed in specific project settings.
Limitations of primary and secondary prevention
Global disparities in access to HPV vaccines pose a significant obstacle to practical primary prevention efforts. Although vaccination programs have succeeded in certain regions, substantial gaps persist, notably in resource-poor countries, particularly in Asia and Latin America [23]. The challenges in these areas are compounded by issues related to secondary prevention, which involve early therapy and screening of precancerous lesions. In low- and middle-income countries (LMICs), secondary prevention faces hurdles due to reliance on screening methods that are often ineffective. For instance, dependence on cytology (Pap smears) or visual inspection is hindered by limited availability, restricted access to health facilities, and subpar pathology services in these regions [24]. The success of both primary and secondary prevention depends on factors such as population coverage and resource constraints. The effectiveness of prevention programs is impeded by limited access to healthcare facilities, inadequately trained providers, and a shortage of essential resources, particularly in LMICs [25]. These challenges underscore the urgent need for comprehensive strategies addressing not only vaccine accessibility but also the improvement of screening methods and overall low- and middle-income countries healthcare infrastructure to achieve meaningful progress in HPV prevention worldwide.
Emerging technologies in cervical cancer prevention
Emerging technologies for cervical cancer prevention include novel screening techniques such as HPV DNA testing, thermal ablation for treating precancerous lesions, and digital health interventions. These advancements aim to enhance the accuracy, efficiency, and cost-effectiveness of identifying women at risk for cervical cancer while also refining treatment and care. For instance, thermal ablation, endorsed by the WHO, employs heat beams to eradicate precancerous cervical lesions, offering significant potential for cervical cancer prevention. Additionally, digital health interventions are being explored for streamlined treatment and care of cervical cancer. These emerging technologies promise to advance cervical cancer prevention and care globally [26]. An overview of emerging technologies for cervical cancer prevention is presented in Table 1.
Overview of the emerging techniques in the prevention of cervical cancer
Technology | Description | Application | References |
---|---|---|---|
Thermal ablation | Utilizes heat beams to eradicate precancerous cervical lesions. | Significant potential for cervical cancer prevention | [26] |
Liquid-based cytology and automation | This enhances case adequacy and lab productivity, facilitates HPV testing, and is compatible with automated analysis. | Enhanced screening efficiency | [27–29] |
Point-of-care HPV molecular test | Crucial role in allowing the clinical research team to offer post-test counseling to women who tested positive for HPV. | Self-sampling and high-resolution microendoscopy technologies | [31, 33] |
Molecular testing advancements | Genomic techniques analyze oncogene and tumor suppressor gene profiles at the DNA level, focusing on clinically relevant biomarkers associated with HPV infections. | Integration of high-risk HPV DNA detection into prevention efforts, potential for improved risk assessment and triage | [36, 37] |
Artificial intelligence (AI) | AI enhances accuracy and efficiency in interpretation, aids non-invasive differentiation of cervical cancer, and supports triaging of HPV-positive individuals. | Real-time and reliable diagnosis, automated recognition of cervical precancer and cancer | [38–42] |
Precision medicine | Tailoring prevention and treatment strategies based on the genetic, environmental, and lifestyle characteristics of each patient. | Genomic techniques for genotype-phenotype associations, digital health interventions for personalized care and support | [47–49] |
CRISPR/Cas9 application | Utilized for molecular diagnosis, identifying cancer susceptibility genes, treating cervical cancer tumors in mice, targeting HPV and PD-1 for potential human trials. | Potential as a versatile tool for research and therapeutic purposes in cervical cancer | [66–68] |
RNA interference (RNAi) | Suppression of target genes, reduction in E6 and E7 mRNA levels, apoptosis in cancer cell lines. | Promising as a genetic engineering technique for treating cervical cancer | [69] |
Biomarkers | HPV DNA testing, miRNAs, proteins, HPV E6/E7 oncogene transcripts, p16INKa/ki-67, M-CSF, VEGF, DNA methylation, PD-L1 status, mismatch repair status, tumor mutational burden status. | Potential in enhancing early detection and prognosis, further research needed for clinical applicability | [70–73] |
PD-1: programmed death 1; PD-L1: programmed death ligand 1; M-CSF: macrophage colony-stimulating factor; VEGF: vascular endothelial growth factor
Liquid-based cytology and automation
Liquid-based cytology (LBC) and automation have significantly impacted cervical cancer screening. LBC, which changes how samples are prepared, has enhanced case adequacy and laboratory productivity. It has additionally facilitated HPV testing, enabling the assessment of mild abnormalities, monitoring post-treatment progress, and initial screening with a subsequent referral for cytology, especially in populations that have received HPV vaccinations. LBC is also compatible with automated analysis of cervical cytology samples. However, certain studies have indicated that automated analysis may have reduced sensitivity compared with manual examination [27].
Nevertheless, LBC is equally sensitive to conventional cytology and provides additional advantages such as enhanced screening efficiency [28]. Moreover, ongoing research on using deep learning to classify whole-slide images of LBC specimens has shown high performance in evaluating neoplastic cervical LBC images [29]. This highlights the potential of automation and advanced technologies further to enhance the accuracy and efficiency of cervical cancer screening.
Point-of-care HPV molecular test
Point-of-care (POC) testing plays a crucial role in allowing the clinical research team to offer post-test counseling to women who test positive for HPV, which is an essential step in comprehensive screening and care. A recent study conducted in Peru documented in-depth interviews with these women, revealing that many experienced fear upon learning of their positive HPV test results, particularly regarding the transmission and potential consequences of HPV infection [30]. However, they found relief, knowing that simple and quick outpatient treatments were available at the primary-care level.
Self-sampling and high-resolution microendoscopy (HRME) technologies represent advanced POC testing methods. Self-sampling offers convenience, cost-effectiveness, and increased screening uptake, potentially reducing the disparities in cervical cancer rates [31]. Challenges remain in establishing cost-effective and ideal triage strategies for HPV testing of self-collected samples [32]. HRME, a POC diagnostic tool, allows real-time imaging of cervical lesions, aiding the identification of precancerous lesions and early cervical cancer. According to a study by Benjamin [33], in lower-resource settings, where colposcopy and pathology services are frequently scarce, HRME imaging may offer a low-cost, accurate, POC substitute for directed cervical biopsies and colposcopy for the diagnosis of cervical dysplasia.
Additionally, the Abbott RealTime High-Risk HPV assay detects 14 high-risk HPV genotypes, including HPV 16 and 18, and provides clinically meaningful results for cervical cancer screening [34]. Moreover, the Cobas HPV test is a valuable tool for detecting high-risk HPV genotypes in cervical specimens, aiding patient management decisions based on the presence or absence of HPV. This test is indicated for various scenarios, such as screening patients with ASC-US results and adjunctively screening women aged ≥ 30 to assess high-risk HPV presence [35]. Implementing internal controls in self-collected samples enhances the applicability of high-risk HPV testing as a primary screening tool, potentially increasing participation rates and improving screening outcomes.
Molecular testing advancements
Advancements in molecular testing for cervical cancer have resulted in a significant shift in screening and prevention methods. Genomic techniques now allow for the analysis of oncogene and tumor suppressor gene profiles at the DNA level, shedding light on the underlying molecular mechanisms of cervical cancer. These molecular screening tests primarily focus on clinically relevant biomarkers associated with HPV infections, the primary sources of cervical cancer [36]. The integration of high-risk HPV DNA detection into cervical cancer prevention efforts has been steadily increasing, offering potential benefits for better risk assessment and triage of HPV infection.
These developments hold promise in revolutionizing cervical cancer screening programs. For example, a “molecular” Pap test involving liquid biopsy from exfoliated cells is envisioned to provide a flexible format adaptable to evolving technological platforms. Molecular testing, which includes assessing viral and cellular CpG methylation levels, can generate cumulative risk scores for individuals with HPV infections, leading to improved risk stratification and possibly reducing the burden of HPV-related cancers [37].
Artificial intelligence in screening interpretation
Artificial intelligence (AI) is used to transform cervical cancer screening by enhancing its accuracy and interpretation efficiency. The increasing prevalence of AI-based medical diagnostic applications offers several advantages, including faster processing times, reduced reliance on specialized personnel, and elimination of subjective biases. AI holds the potential for non-invasive differentiation of cervical cancer from other conditions, and advancements in AI technologies are expected to significantly improve predictive capabilities significantly, thereby maximizing enhancements in cervical cancer screening and diagnosis, refining staging systems, and ultimately improving patient outcomes [38].
AI has already been utilized in diagnostic imaging of the cervix, particularly in the transformation zone where most lesions occur, allowing for real-time and reliable diagnosis [39]. When used alongside human colposcopic evaluation, the AI interpretation of cervical images can be a valuable support tool [40]. Furthermore, AI-assisted visual evaluation has the potential to assist in triaging HPV-positive individuals, whereas enhanced visual assessment of the cervix by clinicians, aided by AI, could elevate screening accuracy [41]. Recent AI developments show promise in automating the recognition of cervical precancers and cancers with a high degree of objectivity. In particular, AI algorithms crafted to analyze images taken during visual inspection with acetic acid have shown noteworthy levels of sensitivity and specificity [42]. These developments have the potential to significantly improve the accuracy and efficacy of cervical cancer screening, which will ultimately reduce the effects of the disease and support international efforts to eradicate it.
HPV persistence
Persistent HPV infection is a significant predictor of the development of cervical diseases, exceptionally high-grade CIN, and cervical cancer. Studies have consistently shown that the persistence of HPV infections, especially HPV 16, is strongly associated with a higher risk of CIN2/3 [43]. In a recent study, Bogani [44] further emphasized that HPV persistence is one of the most important predictors of risk of CIN2+ recurrence, with the risk increasing as HPV persistence rises. Viral load is a crucial determinant of persistence, as higher viral loads are linked to longer infection durations and an increased risk of developing precancerous lesions.
Persistent infection with high-risk HPV types is a crucial risk factor for cervical cancer, highlighting the importance of monitoring and managing persistent infections to prevent disease progression [45]. The cumulative risk of developing cervical cancer following persistent carcinogenic HPV infections also increases with age, underscoring the need for continued surveillance and timely intervention for persistent infections [46]. Understanding the dynamics and persistence of HPV infection is crucial for assessing the impact of HPV vaccines, developing effective screening strategies, and improving clinical management.
Preventing cervical cancer with precision medicine
Tailoring prevention and treatment strategies for cervical cancer prevention involve customizing approaches according to each patient’s genetic, environmental, and lifestyle traits. Genomic techniques have been employed to identify oncogene and tumor suppressor gene profiles at the DNA level, offering insights into cervical cancer’s molecular mechanisms. Enhancing our understanding of genotype-phenotype associations in both human and viral contexts is crucial for implementing precision medicine in the screening and treatment of CIN3 and cervical cancer [47], thereby enabling extrapolation, deterrence, and patients’ initial intervention [48].
Digital health interventions are also investigated to support precision medicine in cervical cancer care. For instance, the development of mobile health applications aims to educate users about cervical cancer, increase awareness, and enhance access to care [49]. These interventions can potentially improve patient outcomes by providing personalized care and support.
Individualized risk evaluation
Individualized risk evaluation for cervical cancer entails assessing specific risk factors in individual patients to gauge their chances of developing the disease. Numerous online tools and applications have been designed to offer personalized risk evaluations for cervical cancer. For instance, the My CancerIQ tool for cervical cancer risk assessment prompts users to inquire about factors influencing their cervical cancer risk, such as age, smoking habits, and family cancer history. Subsequently, the tool computes the user’s risk by referencing studies involving individuals aged ≥ 40 years with no prior cancer history [50]. Apart from online tools, precision medicine strategies utilizing genomic applications and digital health interventions can also offer individualized risk evaluations for cervical cancer. For instance, genomic applications can identify oncogene and tumor suppressor gene patterns at the DNA level, thereby offering insights into the molecular mechanisms underlying cervical cancer [51]. Similarly, mobile health applications and other digital health interventions can deliver tailored education and support to cervical cancer patients.
In general, individualized risk assessment for cervical cancer plays a crucial role in identifying individuals with a heightened risk of the disease and implementing suitable screening and preventive measures. The use of online tools, precision medicine methods, and digital health interventions holds promise for enhancing personalized risk assessment and supporting global efforts to eliminate cervical cancer.
Targeted therapies based on genetic profiling
Cervical cancer is a prevalent gynecological cancer. Tailored therapies based on genetic analysis have emerged as promising avenues for treating this condition. Various innovative strategies have been devised to combat cervical cancer, including gene therapy, antiangiogenic medications, immunotherapy, and combination treatments. However, gene-targeted therapy faces significant challenges related to drug delivery mechanisms, emphasizing the critical need to develop safe gene vectors and delivery methods to ensure the success of gene therapy. The treatment of cervical cancer has been approached from a variety of perspectives, including targeting mutation compensation [52]. Genomic profiling of advanced cervical cancer has shown promise for anticipating treatment responses. Genetic variations in crucial genes, such as PIK3CA, PTEN, and ERBB3; pathways, such as PI3K/AKT; and tumor mutational burden (TMB) could be novel indicators for predicting responses to cervical cancer treatment. It’s worth noting that cervical squamous cell carcinoma and adenocarcinoma exhibit distinct molecular profiles and immune characteristics, implying the need for tailored treatments based on tumor subtype. PTEN has emerged as a prognostic indicator for the survival of cervical cancer patients, offering valuable insights into the development and application of diagnostic and predictive tools. Nevertheless, comprehensive prospective studies with larger sample sizes must validate these findings [53].
Promising research directions
Recent advances in genetic editing techniques for cervical cancer treatment have shown significant promise. New findings indicate that innovative genome-editing systems, notably CRISPR/Cas9-based methods, offer the potential for treating cervical cancer by precisely targeting HPV and its related oncogenes, E6 and E7 [54]. Numerous studies have demonstrated the effectiveness of CRISPR/Cas9 in halting cell growth and preventing tumor formation in cervical cancer, both in laboratory settings and in living organisms [55]. Furthermore, utilizing CRISPR/Cas9 to target HPV E6 and E7 has revealed promising therapeutic approaches for managing cervical cancer [56]. These advancements imply that genetic editing systems, particularly CRISPR/Cas9, have considerable potential in developing efficient and targeted cervical cancer therapies. Further research and clinical trials are necessary to thoroughly evaluate the safety and effectiveness of these approaches in clinical practice.
Immunotherapy in cervical cancer prevention
Immunotherapy targeting HPV oncoproteins has emerged as a promising novel approach for treating cervical cancer. One significant advantage of this method is its ability to selectively focus on malignant cervical epithelial cells and dysplastic precancerous cells expressing HPV oncoproteins. This approach has drawn considerable attention, leading to multiple breakthroughs in the laboratory and clinical settings. These advancements encompass the development of adoptive T-cell therapy, vaccines, and checkpoint inhibitors tailored for cervical cancer. While the effectiveness of these immunotherapies varies, many are currently being evaluated in clinical trials [57].
Multiple-phase clinical trials have assessed the efficacy of immune checkpoint inhibitors (ICIs) and tumor-infiltrating lymphocytes (TILs) in treating cervical cancer. These trials have resulted in enhanced clinical outcomes using these therapeutic approaches. [58]. ICIs operate by releasing brakes on the immune system, including programmed death 1 (PD-1), programmed death ligand 1 (PD-L1), PD-L2, and cytotoxic T-lymphocyte-associated protein 4 (CTLA-4) [59]. By inhibiting T-cell activity, PD-L1 is frequently observed on the surface of antigen-presenting cells and TILs and is thought to contribute to developing and maintaining HPV infection. Although PD-L1 expression is infrequent in normal cervical tissue, its prevalence increases in the presence of HPV infection and cervical cancer. Thus, PD-1 and its ligands are suitable targets for blocking to restore T cell-mediated killing and enhance the immune response against HPV-infected or cancerous cells [60–62]. FDA-approved ICIs targeting PD-1/PD-L1, such as pembrolizumab and nivolumab, have demonstrated efficacy in treating cervical cancer, particularly in cases of PD-L1 positive solid tumors and metastatic/recurrent conditions [59]. CTLA-4, another checkpoint protein receptor, negatively regulates T cell activation, suppressing the immune system. Inhibiting CTLA-4 using agents such as ipilimumab has demonstrated the ability to alleviate the immune suppression linked to HPV-driven cancers. However, when used alone, it did not have a significant impact on tumor response in cervical cancer [63]. The combination of ipilimumab with chemoradiotherapy has the potential to improve the immune response against tumors in high-risk cervical cancer patients prone to disease recurrence. Moreover, regardless of PD-L1 status, the combination of PD-1 and CTLA-4 receptor inhibitors such as ipilimumab and nivolumab has demonstrated sustained clinical efficacy in metastatic cervical cancer. The documented side effects of this combined therapy have been manageable and are consistent with previous observations noted in treatments that involved combining nivolumab and ipilimumab [64].
Treating cervical cancer through genetic methods
Emerging evidence suggests that innovative genome-editing systems and genetic techniques capable of targeting and eliminating HPV E6 and E7 genes hold promise as effective strategies for treating cervical cancer. Examples of such approaches include the CRISPR/Cas9 system and RNA interference (RNAi) [65].
CRISPR/Cas9 application in cancer treatment
CRISPR/Cas9, an innovative gene editing technology, is promising for the research and treatment of cervical cancer. It has been utilized for various purposes, such as molecular diagnosis, identification of cancer susceptibility genes, and detection of pathogenic genes associated with cervical cancer. The high sensitivity and precise targeting capability of CRISPR-based molecular diagnostic technology makes it suitable for early cancer screening. Nevertheless, it is crucial to recognize the possible dangers linked with CRISPR/Cas9, as research indicates that its utilization could elevate the risk of cancer owing to double-stranded breaks [66]. However, there have been significant advancements in the use of CRISPR/Cas9 for the treatment of cervical cancer. Researchers have successfully targeted and treated mouse cervical cancer tumors using CRISPR/Cas9 alongside “stealth” nanoparticles, resulting in a 100% survival rate among treated mice [67]. This breakthrough research signifies a significant advancement and opens the door to potential human gene therapy trials for cervical cancer.
Furthermore, studies have shown the beneficial effects of CRISPR/Cas9 targeting HPV and PD-1, demonstrating a synergistic antitumor effect on cervical cancer both in laboratory settings and living organisms [68]. The detailed repair mechanism of CRISPR/Cas9 is depicted in Figure 2. These findings underscore the potential of CRISPR/Cas9 as a versatile tool for research and therapeutic purposes in cervical cancer.
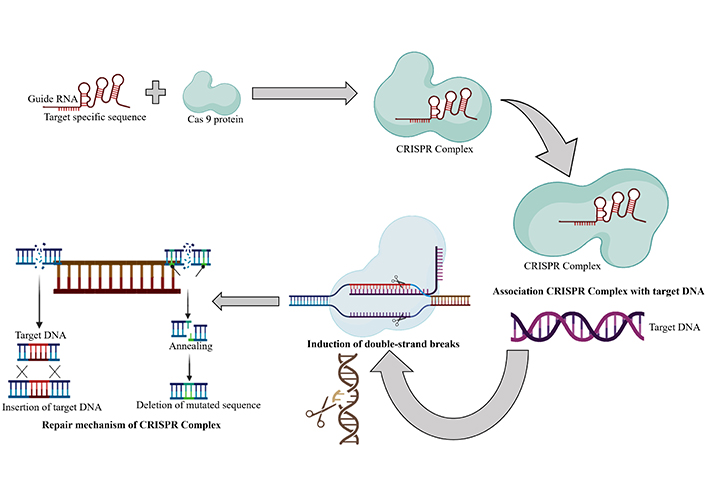
The repair mechanism of CRISPR/Cas9. The repair mechanism of CRISPR/Cas9 involves the association of target DNA with the guide DNA, followed by the induction of double-strand breaks. The deletion or the insertion step is then carried out to eliminate the mutation. Created with BioRender.com
RNAi as a cervical cancer treatment
RNAi, employing short hairpin RNA (shRNA), is a genetic engineering technique that durably suppresses the expression of target genes over extended periods. In a previous study, human cervical cancer cell lines were genetically modified using an Adeno-associated viruses (AAV) vector containing a shRNA designed to target HPV-16 E6/E7. This intervention led to a significant decrease in E6 and E7 mRNA levels. In every cell line under investigation, the reduction in mRNA levels was linked to higher expression of p53, p21, and pRB, which, in turn, caused concentration-dependent apoptosis. Furthermore, a significant decrease in tumor volume was observed in in vivo xenograft models using transduced mice targeting E6/E7 with shRNA. No macroscopic changes were observed, such as edema, inflammation, or weight changes [69].
Biomarkers used in cervical cancer
Cervical cancer is a significant global health issue emphasizing the critical need for early detection to ensure effective treatment. Biomarkers hold promise for enhancing the initial detection and prognosis of cervical cancer, and numerous studies have explored their utility in screening, diagnosis, and prognosis. For instance, HPV DNA testing has been employed as a principal screening method for cervical cancer [70]. Additionally, research has identified miRNAs, proteins, and HPV E6/E7 oncogene transcripts as potential biomarkers for detecting early-stage cervical cancer [71], exhibiting high sensitivity and specificity for distinguishing patients from healthy individuals [72]. Other biomarkers, such as p16INKa/ki-67, macrophage colony-stimulating factor (M-CSF), vascular endothelial growth factor (VEGF), and DNA methylation, have also demonstrated potential in identifying premalignant cervical neoplasia, as shown in Figure 3.
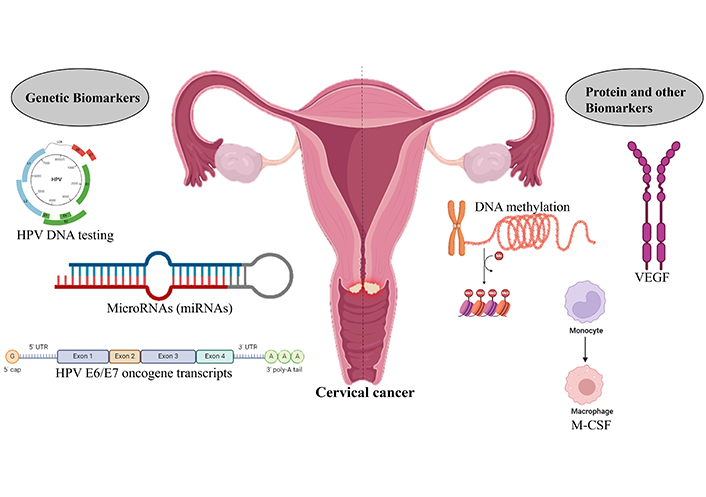
Biomarkers associated with the identification of cervical cancer. Biomarkers such as genetic and protein biomarkers are used to identify the presence of cervical cancer. Created with BioRender.com
Moreover, biomarker testing has proven valuable in determining the PD-L1 status, mismatch repair status, and TMB status, providing insights into the potential role of immunotherapy or checkpoint inhibitors in recurrent cases [73]. These findings underscore the potential of biomarkers for enhancing the early detection and prognosis of cervical cancer. Nonetheless, further research is necessary to validate these biomarkers and to assess their clinical applicability in routine practice.
Anti HPV vaccination
A significant health risk is associated with HPV-related lesions of the lower genital tract, including cervical, vaginal, vulvar, and anal lesions. Nonavalent HPV vaccination, which covers 94.8% of the development of lower genital tract dysplasia, has demonstrated potential in preventing HPV-related lesions [74]. This vaccine is effective in preventing HPV infections in young individuals, which can lead to cervical and other anogenital cancers. Even after treatment, anti-HPV vaccination can prevent new infections and potentially reduce the risk of developing cervical diseases. However, it is essential to note that the vaccine is prophylactic and does not treat HPV-related diseases or infections. The effectiveness of the vaccine may be reduced in individuals with risk factors for HPV infection or disease, such as multiple lifetime sex partners or immunocompromising conditions [75]. The impact of vaccines on the development of HPV-related lesions after treatment is still an area of ongoing research, and more studies are needed to understand its potential role in this context fully.
Minimally invasive surgery
Recent evidence indicates a shift in practice following the LACC (Legal Aid and Consultancy Centre) trial results, which demonstrated that minimally invasive surgery was inferior to traditional open surgery for early-stage cervical cancer [76]. This shift is reflected in the decreased utilization of minimally invasive procedures, such as robotic surgery, for radical hysterectomies, with recommendations now favoring open surgery because of its better outcomes and lower risk of cancer recurrence.
A study by Giannini [77] further confirmed these findings, showing that minimally invasive surgery resulted in a lower incidence of lymphocytic than traditional open surgery, improving quality of life and reducing morbidity. Additionally, a study found that both minimally invasive surgery and conventional laparotomy had similar effects in reducing the levels of circulating biomarkers. Despite the benefits of minimally invasive surgery, concerns regarding the higher risk of cancer recurrence and mortality have prompted a re-evaluation of its role in the treatment of early-stage cervical cancer [78].
Perspectives for the future
The outlook for cervical cancer research and technology appears promising, focusing on overcoming current challenges and capitalizing on emerging opportunities. Treatment progress, including targeted and combination treatments, is anticipated to enhance treatment outcomes, particularly in affluent nations with well-established vaccination and screening programs. However, the global health impact of these advances hinges on addressing access disparities to advanced therapies and preventive measures, especially in resource-constrained settings [79]. Future research endeavors will likely explore innovative approaches that utilize emerging technologies to bolster cervical cancer awareness, prevention, and treatment. Digital health interventions, AI, and personalized screening strategies are areas of focus for potential advancements in cervical cancer care [80]. Moreover, there is a pressing need for increased research in developing countries and marginalized communities to ensure equitable access to the benefits of technological progress [81].
The development and implementation of these advancements pose challenges and opportunities. Although novel technologies hold promises for enhancing the accuracy of cervical cancer prevention, their effective deployment requires substantial time and resources. The shift from conventional screening methods to personalized approaches presents challenges and opportunities for risk assessment and integrating new biomarkers to enhance screening effectiveness. In conclusion, cervical cancer research and technology offer significant potential for progress in treatment, prevention, and screening strategies. Addressing access disparities, embracing emerging technologies, and conducting inclusive research are imperative to maximize the impact of these advancements on a global scale.
Conclusions
The future of cervical cancer prevention is marked by a promising landscape of advances in research and technology, which offers opportunities to impact the burden of this global health challenge significantly. The current state of cervical cancer, with its high incidence and mortality rates, underscores the urgent need for comprehensive preventive measures. The integration of primary and secondary prevention strategies, including vaccination, screening, and early detection, has been successful in reducing cervical cancer rates, particularly in developed countries. The emergence of innovative technologies has transformed the cervical cancer prevention landscape. LBC, automation, and molecular testing have enhanced the accuracy and efficiency of screening methods. AI is poised to revolutionize screening interpretation, offer non-invasive differentiation, and improve diagnostic capabilities. These technologies streamline the processes and provide more objective and precise screening outcomes.
Precision medicine introduces a personalized approach to cervical cancer prevention that considers individual genetic, environmental, and lifestyle factors. Personalized risk assessment tools, digital health interventions, and targeted therapies based on genetic profiling show promise in tailoring prevention and treatment strategies according to the unique characteristics of each patient. Immunotherapy specifically targeting HPV oncoproteins has emerged as a novel and promising avenue for cervical cancer treatment. ICIs and TILs have shown efficacy in clinical trials, providing new hope for advanced and recurrent cases. The exploration of genetic editing techniques, particularly CRISPR/Cas9-based methods, holds significant promise for treating cervical cancer by targeting HPV and its associated oncogenes. Although these advancements present promising opportunities, further clinical trials and research are necessary to validate their safety and efficacy in real-world clinical settings. The prospects of cervical cancer research and technology highlight the need for global collaboration and inclusive research. Ensuring equitable access to advanced therapies and preventive measures remains challenging, especially in resource-constrained settings. Nevertheless, the potential impact of these advancements on a global scale is immense, providing optimism for diminishing the occurrence and fatality rates of cervical cancer worldwide.
Abbreviations
AI: | artificial intelligence |
CIN1: | cervical intraepithelial neoplasia grade 1 |
CTLA-4: | cytotoxic T-lymphocyte-associated protein 4 |
HPV: | human papillomavirus |
HRME: | high-resolution microendoscopy |
ICIs: | immune checkpoint inhibitors |
LBC: | liquid-based cytology |
miRNAs: | microRNAs |
PD-1: | programmed death 1 |
PD-L1: | programmed death ligand 1 |
POC: | point-of-care |
RNAi: | RNA interference |
shRNA: | short hairpin RNA |
TILs: | tumor-infiltrating lymphocytes |
Declarations
Acknowledgments
We are grateful for the unwavering support and encouragement of the Chettinad Academy of Research and Education.
Author contributions
PKCS and SMT: Writing—original draft, Writing—review & editing. RV: Conceptualization, Writing—review & editing, Supervision.
Conflicts of interest
The authors declare no conflicts of interest.
Ethical approval
Not applicable.
Consent to participate
Not applicable.
Consent to publication
Not applicable.
Availability of data and materials
Not applicable.
Funding
Not applicable.
Copyright
© The Author(s) 2024.