Abstract
The emergence and subsequent advancement of nanotechnology in recent years have greatly benefited the healthcare sector, particularly in the treatment of cancer. As per study, major fatalities are related to the lung cancer. For many years, oral tyrosine kinase inhibitors (TKIs) against the epidermal growth factor receptor (EGFR) family of receptors have been used in the clinic to treat human malignancies, although they observed some very serious adverse effects in the treatment of lung cancer, especially in non-small cell lung cancer (NSCLC). Despite EGFR-TKIs’ exceptional qualities as small-molecule targeted medications, their applicability is nevertheless limited by their poor solubility, inconsistent oral bioavailability, high daily dose needs, high plasma albumin binding propensity, and initial/acquired drug resistance. Article’s purpose is to investigate EGFR-TKI’s effects on lung cancer and get around some of its drawbacks, nanotechnology will be an innovative strategy. An effective tool to increase the effectiveness of these pharmaceuticals is nanotechnology by methods other than oral. This article signifies that a range of nanomedicine delivery systems have been developed to effectively distribute EGFR-TKIs with improved drug release kinetics and tissue-targeting capacity. This review article intends to present information regarding lung cancer and EGFR relation, mechanism of recently approved EGFR-TKI’s targeted therapy, an updated landscape of EGFR-TKIs and their clinical status over lung cancer, advantages and disadvantages of nanotechnology, and new breakthroughs in nano-delivery which mentioned as a significantly better over traditional drug chemotherapy and delivery.
Keywords
Lung cancer, EGFR, tyrosine kinase inhibitor, nanotechnologyIntroduction
A great deal of progress has been made in cancer-related treatments during the last few years, as cancer is often regarded as the greatest threat to people’s health and lives. Lung carcinoma is the most prevalent cancer and has a high incidence of cancer-related fatalities including both men and women globally [1, 2]. That’s to a 2018 analysis, lung carcinoma is the main cause of mortality related to cancer, accounting for 11.6 proportions of cancer diagnoses and 18.4 proportions of fatalities [1, 3]. Patients who are diagnosed in the proximal (60%), regionally (33%), or distance (6%) stages have significantly different 5-year relative survival rates [4]. Small cell lung cancer (SCLC) and non-small cell lung cancer (NSCLC) are indeed the two types of lung carcinoma, with NSCLC account for roughly 80% of cases and SCLC for 20% [5].
The epidermal growth factor receptor is a glycoprotein with a transmembrane structure obtaining an external epidermal growth factor binding and an intracellular tyrosine kinase domain which is mainly expressed in some epithelial, mesenchymal and neurogenic tissue. It regulates signaling processes to govern the growth of cells by triggering many signal transduction cascade. This continuous process leads to the activation of tumor phenotype. Not only this sustained process but mutation in EGFR leads to malignancy in lungs like adenocarcinoma and sometime patients does not have survival benefit [6]. Traditional lung cancer chemotherapy treatments lack targeting tumor cells specifically, causing severe harm to general cells such as bone marrow arrest, gastrointestinal (GI) responses, and phlebophlogosis [7]. NSCLC generally responds to chemotherapy and radiotherapy less favorably than SCLC. In patients with advanced metastatic illness, chemotherapy-targeted medications, and other supportive therapies may boost survival and relieve symptoms [8].
The discovery of lung cancer mutations has resulted in the development of biochemically tailored therapies to enable patients with metastatic conditions to live longer lives [9]. In most cases, the first-line therapy for NSCLC involves platinum-based chemotherapy, such as cisplatin or carboplatin doublets. However, it has very major negative effects [10]. NSCLC is characterized by multiple gene point mutations, with somatic mutations in the exons of the EGFR gene occurring in roughly 70% of NSCLC patients [11].
Nanoparticles (NPs) are small artificial particles with a diameter of fewer than 100 nanometers made of polymers, lipids, or metals like gold. NPs are particularly useful in a variety of medicinal applications, ranging from diagnosis to cancer treatment [12]. These NPs are strikingly close in size to most biological structures and compounds. As a result, they provide functional features for cancer research both in vivo and in vitro [13].
Role of EGFR-TKI in targeted therapy
Patients with unique activating genetic variants in the EGFR tyrosine kinases region profit more from FDA-approved drugs (exons 18–21). The most frequent EGFR-activating alterations are exon 19 deletions (del19) (45 percent) and the L858R exon 21 replacement (40–45 percent). Exon 18 alteration make up just approximately 5 percent of all EGFR mutations, which is a significantly lower frequency [14, 15]. Figure 1 mentioned recently approved EGFR-TKI drugs as its targeted therapy for lung cancer.
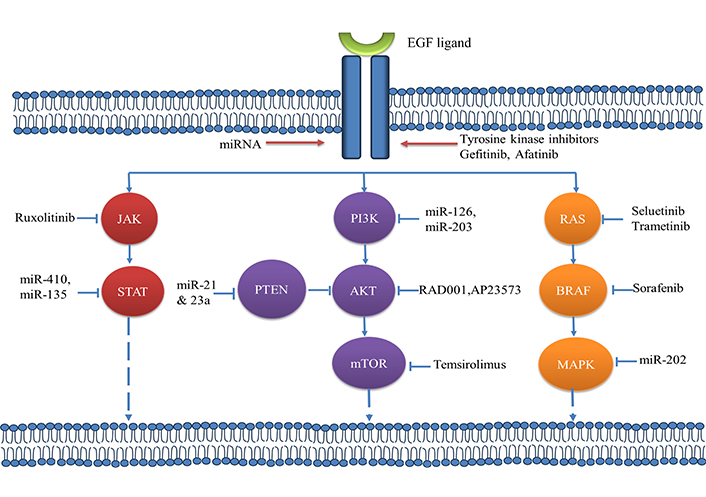
Functioning of tyrosine kinase receptor with recently approved inhibitory drugs [16]
Note. Adapted from “The emerging treatment landscape of targeted therapy in non-small-cell lung cancer” by Yuan M, Huang LL, Chen JH, Wu J, Xu Q. Signal Transduct Target Ther. 2019;4:61 (https://www.nature.com/articles/s41392-019-0099-9). CC BY.
Role of adjuvant EGFR-TKI
Exon 19 deletion mutations, advanced disease stages, prolonged treatment, and the usage of third-generation EGFR-TKIs were all linked to greater disease-free survival (DFS) benefits. Combining therapy EGFR-TKIs should enhance DFS but not Overall Survival (OS) in EGFR mutation-positive patients with stage IB-IIIA NSCLC therapy EGFR-TKIs should enhance DFS but not OS in EGFR mutation-positive patients with stage IB-IIIA NSCLC when compared to subsequent chemotherapy or placebo. The illness stage, length of the treatment, types of mutation, and therapeutic drugs may all have an impact on the degree of effect. Adjuvant EGFR-TKIs demonstrated better tolerance than chemotherapy, especially when first-generation medicines were used [17]. Various clinical trials are undergoing like Wu 2020 (ADURA) [18], Zhong 2018 (ADJUVANT) [19], Yue 2018 (EVAN) [20], Li 2014 [21], Kelly 2015 (RADIANT) [22]. Numerous recent trials have looked into the efficacy of EGFR-TKI therapy as a preoperative treatment for NSCLC. Preoperative EGFR-TKI therapy has the capability to decrease tumor size, enhance radiological outcomes, and boost the likelihood of curative surgery. The effectiveness and safety of neoadjuvant EGFR-TKI treatment were assessed using a pooled analysis of prospectively trial information. Patients having treatable EGFR-variant NSCLC can get adjuvant chemotherapy, EGFR-TKI, or a combination of the two, albeit which is the most beneficial remains unknown. It should be looked into given the superior objective response rate, surgical resection rate, and safety of neoadjuvant EGFR-TKI over chemotherapy for potentially resectable NSCLC. Patients with operable EGFR-mutant NSCLC have access to subsequent chemotherapy, EGFR-TKI, or a combination of the two, while it is debatable which is the most effective. Given the improved objective response rate, surgical resection rate, and safety of preoperative EGFR-TKI over chemotherapy for potentially resectable NSCLC, it should be investigated [23]. Table 1 mentioned recently approved EGFR- TKI and their clinical status.
Clinical Status of recently approved drugs [24, 25]. The fundamental functional principle and clinical aspect of the four FDA-approved EGFR-TKIs that are most frequently used are summarised in this gefitinib (Iressa), erlotinib (Tarceva), osimertinib (Tagrisso), afatinib (Gilotrif), and dacomitinib (Vizimpro) [26]
TKI | Erlotinib | Gefitinib | Afatinib | Dacomitinib | Osimertinib |
---|---|---|---|---|---|
Brand name | Tarceva | Iressa | Gilotrif | Vizimpro | Tagrisso |
Dose | 150 mg 1 h before or 2 h after food | 250 mg +/− food | 40 mg 1 h before or 2 h after food | 45 mg orally once daily | 80 mg +/− food |
Indication | First generation for advanced NSCLC | First generation for advanced NSCLC | Second generation for advanced NSCLC | Second generation for advanced NSCLC | Third generation for advanced NSCLC |
Side effects | Rash, diarrhea, edema, cough, conjunctivitis | skin reaction, rash, anorexia, stomatitis diarrhea, paronychia | Eruption rash dry skin, diarrhea, loss of appetite, stomatitis, diarrhea | Diarrhea, interstitial lung disease, stomatitis, rash | QTc prolongation, ocular disorder cardiomyopathy |
MOA | Competitive; reversibleEGFR L858R, Del19 | Competitive; reversibleEGFR L858R, Del19 | Covalent; irreversibleEGFR L858R, L858R/T790M | Covalent; irreversibleEGFR L858R, L858R/T790M | Covalent; irreversible |
Drug approval year | 2004 | 2003 | 2013 | 2012 | 2015 |
Status | Phase III approved (2012) | Phase III approved (2019) | Phase III approved (2021) | Phase III approved (2018) | Phase III approved (2021) |
NSCLC: non-small cell lung cancer; MOA: mechanism of action
Some recent combinations of EGFR-TKI
In 2022, a novel family of EGFR-TKIs will be developed through structural changes of 2,4-dihydroindeno[1,2-c]pyrazoles. These chemicals were compared to erlotinib’s potency [27] (The sponsor decided to discontinue the trial after deciding to examine the pairing of quaratusugene ozeplasmid and osimertinib rather than quaratusugene ozeplasmid and erlotinib [28]). The US FDA granted fast-track designation to a pair of quaratusugene ozeplasmid immunogene therapy and pembrolizumab for the treatment of patients with relapsed stage III or IV NSCLC who had already worsened on pembrolizumab [29]. Currently, phase II and III trials are being conducted to compare osimertinib against osimertinib plus chemo treatment, such as cisplatin/carboplatin and pemetrexed. The OPAL Study (NEJ032C/LOGIK1801) is a phase II study of osimertinib in association with platinum with pemetrexed in patients suffering from advanced NSCLC who have EGFR mutations [30]. Combination chemotherapy can also boost the number of people in remission. The patient with EGFR-mutated advanced NSCLC underwent well-tolerated combination therapy and benefited from osimertinib in conjunction with chemotherapy and bevacizumab [31]. For first-line chemotherapy, a combination of osimertinib and gefitinib is acceptable [32] as well as second-line treatment (OSCILLATE) [33] of EGFR-modified NSCLC resulted in fast plasma clearance of the EGFR mutation. Dual EGFR inhibition with gefitinib + osimertinib may postpone the establishment of acquired resistance, according to preclinical research [32].
Just for knowledge, osimertinib was compared to afatinib for the treating of 790M-positive NSCLC and numerous central nervous system (CNS) lesions after lack of first EGFR-TKI medication in an Asian population, and osimertinib was found to be superior to afatinib [34].
Nanotechnology in cancer
Regardless of the fact that chemotreatment and radiotherapy are treatment options for lung malignancy, the majority of patients experience a recurrence that is more resistant to subsequent treatment. A promising pharmacological approach is required to improve the prognosis of this type of cancer. Significant improvements in the research and the utilization of nanotechnology in the diagnostic and treatment of this form of cancer have been made in recent years, including the usage of LBNPs [35]. Nanomedicine is a comparatively recent treatment that aims to replace drug delivery and boost therapeutic advantages while limiting negative impacts on healthy tissues [36]. Blood circulation and clearance of the drug provide balance between its activity and toxicity. Due to the physicochemical properties of the nanomaterials, it mainly distributed in lung, liver, and kidney hence their clearance is expected to occur from these organs. The safety and effectiveness of nanocarriers are not assured and cannot be precisely managed due to the unknown processes of absorption, distribution, metabolism, and excretion, also the drug release mechanism of nanocarriers in the body. Therefore, they are rarely approved for clinical usage [37, 38]. On consideration of toxicity human body there is 2 types of toxicity e.g., primary [inflammation, oxidative stress, deoxyribonucleic acid (DNA) damage by direct exposure to organs like lung, skin, etc.] and secondary (inflammation, ROS generation, altered cellular function, etc.) [39]. Therefore, very least are actually there in clinical trials recently for lung cancer as mentioned in Table 2.
Clinical trials of EGFR-TKI with nanoparticle in lung cancer. In August 2020, a clinical trials.gov advanced search for “nanoparticles and lung cancer” was conducted. These were evaluated and chosen based on the study’s current status.
Sr. No. | Clinical trial | Study type | Description | Status |
---|---|---|---|---|
1 | NCT00553462 | Phase II | This phase II trial is studying how well giving carboplatin and paclitaxel albumin-stabilized nanoparticle formulation together with radiation therapy and erlotinib works in treating patients with stage III NSCLC that cannot be removed by surgery. | Completed |
2 | NCT04486833 | Phase I–II | Safety and efficacy of GPX-001 + osimertinib in NSCLC patients with activating EGFR mutations who have progressed while on treatment with osimertinib. | Recruiting |
Despite the success of different drug delivery systems in recent years, there are yet certain challenges that be resolved and advanced technologies to be developed in order to successfully carry pharmaceuticals to their local sites. As a result, nano-based drug delivery devices are still researched to aid in improved drug delivery [40]. Two types of NPs used in the treatment of lung cancer, organic NPs (liposomes, solid lipid nanoparticle, nanostructued liquid carriers, polymeric NP, colloidal polymeric micelles, dendrimer, etc.) and inorganic NPs (quantum dots, magnetic NPs, carbon nanotubes, etc.) [41]. Figure 2 mentioned the various nanomaterials depending on their physicochemical properties.
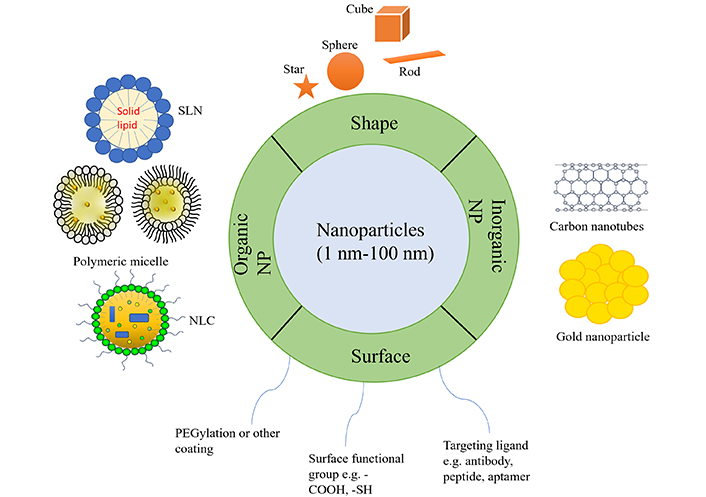
Describing physicochemical characteristics of nanoparticle. SLN: solid lipid nanoparticle; NLC: nanostructured lipid carriers; NP: nanoparticle
There are numerous advantages of using NPs over other materials. Because of the enhanced permeability and retention (EPR) action, NPS can stay in the tumour location. Another virtue of NPS is that its surface may be easily modified to reduce inappropriate ingestion by the reticuloendothelial system [42]. The large surface-area-to-volume ratio is another feature that permits therapeutic compounds to be held successfully and not degraded by the microenvironment. Nanomaterials are also being used to produce more effective and regulated drug delivery techniques [40]. Nanotechniques have proven to bridge the gap among biological and morphological sciences through the use of nanostructures and nanophases in a wide range of scientific domains [43]. Such particles are very important in nanomaterials and medication delivery systems based on nanotechnology [44, 45].
Advantages of NPs
Nanostructures stay in the bloodstream for a long period of time, allowing coupled drugs to be delivered at the appropriate dose. As a result, they cause less plasma fluctuations and have fewer negative impacts. These nanostructures penetrate the tissue matrix, allowing for easy drug uptake by cells, efficient drug distribution, and targeted action. Nanostructures are substantially easier for cells to consume than large particles with sizes ranging from 1 to 10 µm [46, 47]. NPs provide the best way to deliver natural compounds with high safety and efficacy. These nanomaterials are labeled with drugs that have minimal penetration and ingestion [47, 48]. Thus, nanotechnology provides relevant qualifications in the treatment of lung cancer via location specific and goal-oriented medication delivery [40]. NP based medicines developed not just for treatment but also for diagnosis, imaging, detection, etc. of the lung cancer [49].
Application of NPs
Getting the drug to the proper place in the body while limiting any unwanted effects on healthy organs is one of the most complex aspects of drug transmission. This is especially challenging in cure for cancer because the tumor may appear as an organ lesion. These strategies are aided by certain NPs. These benefits include their tiny size, which enables them to pass through cell membranes, link and stabilize peptides, and exit from lysosomes following endocytic [50]. The goal of drug capture in nanotechnology is to either boost release of the drug to or ingestion by specific receptors, or to reduce the toxic effects of the free drug to non-target systems. Lengthy and target-specific nanomaterials are required to achieve these goals. NP capture in the liver and spleen’s cellular eosinophils system is one of the issues [51]. Irrespective of how the treatment is administered, the bloodstream’s bioavailability permits active substance to travel to and contact practically all tissues throughout the body, and once there is drug content in the circulation, it can pass through endothelium obstacles. To build exact localization, a transporter that can disperse the medicine directly towards the target area can be used [52]. After NPs reach the intended region via systemic circulation, the interaction of ligands and receptors is referred to as active targeting. Only close proximity (0.5 nm) between the two components allows for ligand-receptor interaction [53]. Polyethylene glycol (PEG) surface renovation culminated in longer bloodstream circulation by blocking identification and consumption by the single-nuclear engulfing system [54]. Surface charge and other chemical characteristics may influence NP fate in cells. Gold NPs with PEG surfaces were effectively up taken in endosomes and cytoplasm, with nuclear localization [55]. Cells ingested poly(DL-lactide-co-glycolide) NPs by endocytosis [56, 57]. carbohydrate-binding agents to increase cellular contact on the interface of both biocompatible and biodegradable polylactic-co-glycolic acid (PLGA) nanospheres [58]. When the drug is administered as NPs or incorporated into them, higher adherence may contribute to improved drug activity. The attachment of certain peptides, like antibodies, to the nanomaterial surface may allow for more precise immunologically directed particle localization [59, 60]. Antibodies (immunoglobulin) are a crucial component in the formation of a system that actively targets NPs. Single-chain segment factors and antibody antigen-binding segments have two lighter and heavier chains that can bind sensors or antigen, respectively [61]. Folate, often known as folic acid belongs to the B vitamin family. It is a glycosylphosphatidylinositol-anchored cell-surface receptor, and this system is a ligand that is ideal for targeting tumor cells [62]. Aptamers are non-toxic targeting ligands that have more flexibility, stability, low molecular weight, and repeatability, than natural antibodies [52]. Table 3 mentioned the recent NP formulations of EGFR TK anticancer drugs.
Summary of the recently published studies about the EGFR TK anticancer drugs-loaded Nanoparticulate formulations for the treatment of LC
Sr. No. | Drug | Nanoparticle formulation | Aim/Description | Year | Ref |
---|---|---|---|---|---|
1 | Afatinib | Liposome | LPN modified with FD7 or CCD as a delivery platform was explored to enhance afatinib delivery across the BBB model of mouse brain-derived endothelial bEnd.3 cells. | 2021 | [63] |
2 | Osimertinib | Liposome vesicle | Liposome-based delivery of OSI can provide a new formulation of the drug that can be administered via alternative delivery routes (intravenous, inhalation). | 2020 | [64] |
3 | Gefitinib | Liposome | Gefitinib encapsulation based on nano liposome for enhancing curative effect of lung cancer | 2020 | [65] |
4 | Gefitinib | SLN | Preparation and evaluation of inhalable dry powder containing glucosamine-conjugated gefitinib SLNs for lung cancer therapy | 2020 | [66] |
5 | Gefitinib | Liposome | Liposomal gefitinib dry powder inhalers (LGDs) were prepared using the injection-lyophilization method | 2020 | [67] |
6 | Afatinib | Luminescence nanoparticles | Next-generation cancer-specific hybrid theranostic nanomaterials: MAGE-A3 NIR persistent luminescence nanoparticles conjugated to afatinib for in situ suppression of lung adenocarcinoma growth and metastasis | 2020 | [68] |
8 | Erlotinib | SLN | Co-encapsulated nanoparticles of Erlotinib and Quercetin for targeting lung cancer through nuclear EGFR and PI3K/AKT inhibition | 2022 | [69] |
9 | Erlotinib | Mesoporous silica nanoparticle | Injectable thermosensitive hydrogel containing erlotinib-loaded hollow mesoporous silica nanoparticles as a localized drug delivery system for NSCLC therapy | 2020 | [70] |
LPN: lipid polymeric nanoparticle; BBB: blood brain barrier; SLN: solid lipid nanoparticle; EGFR: epidermal growth factor receptor; NSCLC: non-small cell lung cancer
Table 4 mentioned newer combinations of anticancer NP formulations respectively.
Some newer combinations of TKI
Sr. No. | Drug | Nanoparticle formulation | Description | Year | Ref |
---|---|---|---|---|---|
1 | Icotinib & doxorubicin | EDS nanoparticle | In conclusion, the present study demonstrated that the use of synergistic NPs was effective for in vitro and in vivo targeted delivery and the inhibition of NSCLC and greatly enhanced the synergistic effect of doxorubicin and icotinib | 2020 | [71] |
2 | Erlotinib & bevacizumab | pH-sensitive lipid-polymer hybrid nanoparticles | Non-small cell lung cancer combination therapy: hyaluronic acid modified, epidermal growth factor receptor-targeted, pH-sensitive lipid-polymer hybrid nanoparticles for the delivery of erlotinib plus bevacizumab | 2020 | [72] |
3 | Erlotinib & bevacizumab | Magnetic nanoparticle | A multifunctional nanotheranostics agent potentiates erlotinib to EGFR wild-type non-small cell lung cancer | 2022 | [73] |
4 | Gefitinib & thymoquinone | PLGA nanoparticle | Delivery of gefitinib in synergism with thymoquinone via transferrin-conjugated nanoparticle sensitizes gefitinib-resistant non-small cell lung carcinoma to control metastasis and stemness | 2021 | [74] |
5 | Osimertinib & selumetinib | Micelle | Nanoparticles for co-delivery of osimertinib and selumetinib to overcome osimertinib-acquired resistance in non-small cell lung cancer | 2021 | [75] |
6 | Erlotinib & doxorubicin | Rhamnolipid-coated W/O/W double emulsion nanoparticles | RL-NP-DOX-ERL provided efficient delivery of doxorubicin and ERL to tumor tissue and successful tumor therapy with a synergetic effect | 2021 | [76] |
NPs: nanoparticles; NSCLC: non-small cell lung cancer; EGFR: epidermal growth factor receptor; PLGA: polylactic-co-glycolic acid; RL-NP-DOX-ERL: rhamnolipid-coated double emulsion nanoparticles containing doxorubicin and erlotinib
Recent advances in EGFR-TKI drug NPs in cancer
Organic NPs
Liposome
Alec Bangham was the first to find them in 1960. Liposomes are a well-known medication delivery technology used to enhance medicine distribution. They are circular packets made up of phospholipids and immunosuppressant with diameters that vary from 50 to 450 nm [77]. They have also been proven to stabilise medicinal molecules, promote biodistribution, work with both water loving and water phobic medications are available, and they are both biocompatible and biodegradable. Four categories of liposomes: (1) Water nucleus was wrapped by a lipid bilayer that could produce anionic, cationic, or neutral lipids and phospholipids in traditional liposomes. Water loving or water phobic materials can be used to infill both the cell membrane and the aquatic area in this scenario. (2) PEGylated varieties: PEG is introduced into the liposome’s interface to achieve steric saturation. (3) Ligand-targeted type: ligands such as polysaccharides, antibodies and peptides are bonded to the liposome’s interface or the ends of already binded PEG sequence and (4) Theragnostic liposome: This form of liposome is hybrid of the previous 3 categories, containing a NP as well as a targeting, imaging, and therapeutic component [78, 79]. Afatinib was encapsulated in “liposomes” (LPs) to allow for prolonged blood circulation and increased tumor permeability and retention. Cetuximab was also created to attach to drug-loaded liposomes, resulting in the formation of “immuno-liposomes” with tumor-cell selection and therapeutic action [80]. The ammonium sulfate gradient approach can be used to make afatinib liposomes with a greater encapsulation efficiency and a smaller particle size [81]. The pH-sensitive liposome with afatinib is a novel targeted medication delivery system for cancer treatment. Additionally, the inhibitory effect on H-1975 and HCC-827 was showed by demonstrating that afatinib-loaded pH-sensitive liposome had high anticancer activity [82].
Solid lipid nanoparticle (SLN)
SLNs are a novel dispersed drug administration technique constituted of biological oily material which remained solid at ambient and internal temperatures [35]. SLN has the potential to transport both hydrophilic and lipophilic medicines, as well as a high level of stability, extended bloodstream persistence, and the ability to be created from biocompatible materials [83]. These particles range in size from 50 to 1,000 nm. Solid lipids used as a solid material for drug encoring possessing monosaccharides, fatty acids, disaccharides, triglycerides, and compound glyceride mixtures. This matrix is stabilized by a mixture of surfactants or polymers [35]. SLNs pair the characteristics of a fat-emulsion carrier, liposome carrier, and polymeric NP, building them ideal for localized drug administration [84]. The ability of SLN to transfer the commonly used cytostatic (5-fluorouracil) through A549 cells’ resistance [85]. Treatment of NSCLC with microparticles containing erlotinib-loaded SLN by formulating dry powder inhaler to increase cargo drug molecule in alveoli adenocarcinoma over oral dosage of erlotinib [86]. Cetyl alcohol, Compritol 888 ATO, bee wax, cholesterol butyrate, and emulsifying wax are the most often utilized solid lipids for making SLN [87]. As per Wioletta Zielińska [88] possibilities for using SLNs in combination with 5-fluorouracil to defeat NSCLC cell line A549’s drug resistance. Unfortunately, like other colloidal systems, the SLNs have low drug loading, which is restricted by the drug’s solubility in the melting lipid phase and its ejection during storage [89, 90]. Afatinib-loaded SLN and paclitaxel delivered via PLGA porous microspheres dry powders: a newer therapy for EGFR inhibitors of tyrosine kinase NSCLC resistant. Author showed that combination of the EGFR-TKI with other chemotherapeutic agent would be feasible to overcome resistant over resistance produced by sing EGFR-TKI [91].
Nanostructured lipid carriers (NLC)
NLC (nanostructured lipid carriers) are second-generation lipid-based NPs that were first published in the mid-1990s to address the drawbacks of SLNs [92]. NLC are formed up of solid and liquid lipids that have been partially crystallized and dispersed in an aqueous phase containing an emulsifier [91]. This loosely packed crystalline structure allows drug molecules to be entrapped, reducing drug leakage during storage and allowing regulated drug release [93]. The NLC system has a low drug loading capacity because of the crystalline structure of the lipids and gelation in the dispersed phase due to solid lipid polymorphism [94]. In 2019, researchers compared erlotinib-loaded NLC and liposomes vs. the A549 lung cancer cell line in vitro. Erlotinib-NLC were more effective against cancer than erlotinib-liposomes mentioned in Table 4 [67].
Polymeric NPs
Polymeric NPs have already been widely studied in the treatment for cancer [95]. Polymers with a variety of structures, both natural and manufactured, can be used. Since they are biocompatible and biodegradable, polypeptides, albumin, gelatin, and chitosan are among the most often utilized natural polymers. Among the synthetic polymers utilized are PEG, polylactic acid, PLGA, and polycaprolactone [96]. Their use of polymeric NP allows maximum packaging of hydrophobic medicines, resulting in longer circulation durations and more effective enforcement at the site of action, as well as an improvement in therapeutic and targeting agent efficacy [97]. The goal of Vaidya’s study [98] was to create a NP method to overcome erlotinib resistance in patients of NSCLC. Erlotinib-cyclodextrin complex was synthesized using cyclodextrin sulfobutyl ether, which was then loaded into the core of biodegradable PLGA NPs via repeated emulsion solvent evaporation. Cationic polymers are known for their cellular toxicity and enhanced clumping in lung capillaries because they are non-degradable and poor compliance. As a result, constant monitoring of cationic polymer-induced toxicity is essential, as they cause dyspnea when they combine with a biosurfactant [99].
Polymeric micelles
Micelles are amphiphilic block copolymer nanomaterials that self-collected in a watery solution that build a conceptual model [40]. Polymeric micelles have a restricted dispersion to avoid too fast elimination through kidney, allowing them to concentrate in carcinomal parts via the EPR effect. Their polymeric coating also minimizes unwanted interactions with bioactive molecules. Their inner space structure allows for drug assimilation, which enhances stability and bioavailability, these nanomaterials have a lot of potential for water hating material transportation [100, 101]. The biodegradable nature of this technique for drug delivery systems, which may be employed for both cancer and eye medication administration, is its main advantage [41]. There are many formulations of EGFR-TKI which were signified for HER2 overexpression, synergistic effect, and multidrug resistance [102].
Dendrimer
Regardless of how the medication is delivered, the bioavailability of the blood permits drug particles to diffuse and get into practically all tissues in the body that are affected, and once the medication is in circulation, it crosses capillary boundaries. A carrier that redistributes the medicine selectively toward the target tissue can be employed to accomplish correct targeting [103]. Drug solubility and drug targeting (active and/or passive targeting) are both affected by dendrimers. Dendrimers have been used to diagnose diseases, particularly malignancies; consequently, we will focus on their diagnostic uses and briefly explore their capacity to deliver anticancer medications here [104]. Dendrimers offer a wide range of applications owing to their functional and structural diversity. They can be used in a variety of applications, possessing gene and small interfering ribonucleic acid (siRNA) delivery, pharmacy, photodynamic therapy, biomedicine, oligonucleotide conjugation, immunology, and imaging [105].
Inorganic NP
Metal-based NP
Metal-based NPs such as gold, silver, carbon nanotubes, and quantum dots have all been studied as potential drug delivery methods in the treatment of NSCLC [106]. Magnetic NPs are made up of a magnetic inner side and an active layer over it. Materials like gold, iron, cobalt, and nickel build up the magnetic core, which gives it its magnetic qualities. The upper cover prevents clustering and decreases the magnetic core’s contact with other substances [107]. Metal-based NPs have shown exponential expansion in research due to their acceptable biocompatibility and simplicity of size manipulation and surface modification. Their ability to block visible light makes them ideal for intracellular tracking [108]. As per Alex Yang-Hao Yu, collagen gold NPs could be used as a platform for siRNA delivery because of the positive findings. Treatment with collagen-gold-EGFRsi resulted in noticeable tumour control effects, with tumour weight reduced by 30% for lipofetamine carriers and 70% for C-Au carriers [109].
Carbon nanotubes
Carbon nanotubes are hydrophobic tubular structures constituted of carbon atoms having diameters vary from 4 nm to 100 mm that change dependence on the graphene molecule arrangement. Carbon nanotubes are inherently not soluble in organic solvents or aqueous solutions, and the toxicity they generate in body fluids is a critical issue that must be resolved [110]. Due to its unique physicochemical properties like low cytotoxicity, bioavailability, etc. has ability to gather into cancer cells without any phagocyte recognition, and hence they achieve high intracellular drug concentration [111]. Carbon nanotubes may enter cells and distribute chemicals into the cytoplasm utilizing “needle-like penetration.” These NPs have a vast surface area, which allows for multiple attachment sites for potential targeting ligands, as well as an interior cavity that can hold therapeutic or diagnostic substances [36]. The parallels between asbestos and carbon nanotubes have sparked widespread worry about potential dangers and are still under investigation. Remember that the asbestos disaster may have been avoided [112]. Chemical treatment increases biocompatibility lowers toxicity and converts them into water-soluble nano-carriers [113].
Conclusions
We have summarized targeted therapy for NSCLC, especially through EGFR-TKI. Numerous receptors are there but EGFR is more commonly responsible for the metastasis of cancer. EGFR-TKIs directly act on the cellular membrane and directly stop tumor growth. These inhibitors are used in various ways to overcome EGFR resistance and to become more potent. This review listed recent advances in EGFR-TKI like new generation drugs and adjuvant therapy. Despite having such good qualities all these have some limitations as these produce adverse effects sometimes very serious. NPs are introduced to overcome side effects and to provide targeted release of these drugs. This study showed that introducing nanotechnology comes with better drug delivery and fewer side effects over traditionally used EGFR-TKI therapy for lung cancer. Types of NPs are introduced some are under clinical trials and some are under process like liposomes, SLNs, NLC, polymeric NP, micelle, dendrimers, etc. But the least numbers are actual in clinical trials for the lung cancer treatment and this could be the starting point to carry out novel delivery using nanotechnology. For the development of the novel delivery of these drugs we need to study the biology and microenvironment of the tumor and then interaction between prepared NP and tumor. Preparation of the NP simple, low cost, reproducible with high safety, selectivity and efficacious towards tumor. This study provides you with information about recent studies and future aspects of EGFR-tyrosine kinase receptor inhibitory drugs also its NPs and newer combinations that provide better therapy for NSCLC.
Abbreviations
DFS: | disease-free survival |
EGFR: | epidermal growth factor receptor |
NLC: | nanostructured lipid carriers |
NPs: | nanoparticles |
NSCLC: | non-small cell lung cancer |
PEG: | polyethylene glycol |
PLGA: | polylactic-co-glycolic acid |
SCLC: | small cell lung cancer |
SLN: | solid lipid nanoparticle |
TKIs: | tyrosine kinase inhibitors |
Declarations
Acknowledgments
Authors are thankful to the Management of Dr Vishwanath Karad MIT-World Peace University, Kothrud, Pune 411038.
Author contributions
PSW: Writing—original draft, Data curation. ARC: Conceptualization, Writing—review & editing. KGR: Data curation, Validation, Visualization. BGP: Writing—review & editing. SCJ: Supervision, Validation.
Conflicts of interest
The authors declare no conflicts of interest.
Ethical approval
Not applicable.
Consent to participate
Not applicable.
Consent to publication
Not applicable.
Availability of data and materials
Not applicable.
Funding
Not applicable.
Copyright
© The Author(s) 2024.