Abstract
Harmattan is a season characterized by dust, cold, and sub-humid trade winds in Sub-Saharan countries. It’s similar to meteorological phenomena like Asian dust storms, Santa Ana winds, Australian bushfires, and Saharan dust in the Caribbean. It causes profound changes in the cardiorespiratory system in apparently healthy individuals and increases the risk of hospitalization in susceptible individuals. Exposure to these extreme conditions has been associated with alterations in autonomic function and baroreceptor sensitivity thus resulting in dysregulation of blood pressure control mechanisms. Baroreceptors are critical regulators of hemodynamics and cardiovascular function. They play a vital role in the short-term responses to blood pressure perturbation and are essential for acute restoration of blood pressure following cold exposure. Harmattan wind contains a barrage of chemicals, dust, and particulate matters depending on industrialization, natural and human activities. Particulate matter from Harmattan dust can trigger systemic inflammation and oxidative stress, exacerbating endothelial dysfunction and impairing vascular reactivity thus contributing to the pathogenesis of alterations in baroreceptor insensitivity, and cardiovascular diseases, including hypertension and atherosclerosis. Furthermore, fine particulate matter from dust may penetrate deep into the respiratory tract, activating pulmonary sensory receptors and eliciting reflex responses that influence autonomic tone. The presence of rich acrolein smokes and non-essential heavy metals such as cadmium, lead, and mercury in Harmattan wind also reduces baroreflex sensitivity, culminating in a sustained increase in diastolic and systolic blood pressure. This integrated review aims to provide valuable insights into how changes in each of these environmental constituents alter vital pathophysiologic and immunologic mechanisms of the body leading to baroreceptor instability and ultimately hemodynamic imbalance using available primary studies. Understanding this intricate interplay is crucial for implementing targeted interventions and informed public health strategies to mitigate the adverse effects of extreme environmental exposure and ultimately reduce poor health outcomes in the affected regions.
Keywords
Harmattan, baroreceptors, baroreflex, heavy metal, smokes, environmental health, hemodynamic instabilityIntroduction
Harmattan is a seasonal weather pattern with differential characteristics from winter [1]. It is characterized by dusty cold winds and haze, primarily experienced in West Africa from December to March [2]. Harmattan is believed to be caused by trade wind that blows from Sahara to Gulf of Guinea affecting many countries. As the wind blows, it causes environmental changes such as cold, dry and dust. Sahara dust is one of the most conspicuous features of Harmattan season. It is a significant atmospheric phenomenon with global implications. The dust is primarily transported westward across the Atlantic Ocean towards the Caribbean and the United States. In addition to the westward trajectory, dust is also transported northward across the Mediterranean Sea into Southern and Central Europe and, in some extreme cases, towards the shores of the Baltic Sea. Meteorological phenomena in other parts of the world with similar characteristics and detrimental medical implications like Harmattan include the Santa Ana winds in Southern California, which increase wildfire risks and exacerbate respiratory conditions such as asthma and allergies. The Asian dust storms, originating from deserts in Mongolia and Northern China, carry particulate matter (PM) that can trigger asthma attacks and cardiovascular issues. The Australian bushfire season, marked by intense heat and smoke, leads to poor air quality, affecting respiratory health and increasing hospital admissions for respiratory and cardiovascular problems. Similarly, Saharan dust events in the Caribbean and Southern Europe bring fine PM, causing respiratory irritation and increasing the prevalence of respiratory and cardiovascular diseases.
Harmattan dust can float for days or weeks, depending on the dryness, speed, and turbulence of the air masses. The impact of this dust is multifaceted. On one hand, it can have detrimental effects on air quality, potentially triggering asthma attacks and aggravating other respiratory conditions such as runny nose, coughing, and sneezing [2]. On the other hand, the dust plays a crucial role in fertilizing soils with phosphorus and other nutrients. Harmattan increases the risk of dehydration and dryness while in people with underlying ailments, it may exacerbate the risk of dehydration, asthma, hypertension, and skin-related problems [3, 4]. The cold weather accompanying the Harmattan season is predominantly associated with changes in the hemodynamic parameters and increased risk of hospitalizations for hypertension, congestive cardiac failure, and cerebrovascular accident (CVA) among susceptible individuals [5–7]. In addition to respiratory, cardiovascular, skin, and ocular dysregulation, Harmattan weather can also have other indirect systemic effects. This includes systemic inflammation and activation of the coagulation cascade, increasing the risk of thrombotic events. Increased oxidative stress leads to potential alterations in white blood cell counts, exacerbation of sickle cell crisis, and gastrointestinal irritation [4, 7]. Despite the various health issues attributed to Harmattan, the extent to which it exacerbates the risk of cardiovascular-related complications in humans remains inconclusive [8].
Harmattan is also accompanied with dusts of different sizes such as large, small, fine, and ultrafine (nano-size) dust particles [9]. Despite the human body being fortified with physiological mechanisms including cough, sneezing, and mucociliary clearance to get rid of dust particles of certain dimensions, nano-sized dust particle often reaches the alveoli and alveolar capillary beds where they are engulfed by pulmonary alveolar macrophage (PAM) [10]. In some cases, prolonged exposure to dusty Harmattan can propagate PAM inflammatory actions in the respiratory tract causing the release of several cytokines such as interleukin (IL)-1, tumor necrosis factor-alpha (TNF-α), interferon-gamma (IFN-γ) [11, 12]. These pathophysiological changes may further aggravate the risk of cardiovascular-related complications among susceptible individuals [13–15].
Furthermore, a fraction of the nano-sized dusts during Harmattan exposure may be absorbed into the bloodstream and settle on the tunica intima of blood vessels and interior lining of viscera where they may interfere with constitutional functions of the viscera impairing normal hemodynamics [13]. It is crucial to maintain systemic hemodynamics, which calls for several regulatory units, including the unique mechanoreceptors, also referred to as baroreceptors. Baroreceptors are free nerve endings situated in the carotid sinus and aortic arc, heart chambers, large systemic veins, and pulmonary vasculature and they are essential for short- and long-term regulation of blood pressure [16, 17]. High-pressure baroreceptors are present in an aortic arc and carotid sinus, and they are activated by distension within where they are located causing a decline in heart rate and diastolic blood pressure to keep the mean arterial blood pressure within a homeostatic range [18]. When low-pressure baroreceptors are stimulated, ventricular contractility is enhanced with an increased heart rate [19].
Changes in the dimension of baroreceptor-containing structures elicit reflex activation of baroreceptors and conduction of electrical impulses through branches of glossopharyngeal and vagus nerves [20]. Like other phasic receptors, baroreceptors are capable of adapting (resetting) to the luminal diameters of the structures where they reside [18]. Harmattan being a multifactorial environmental change can influence the functions of baroreceptors through various mechanisms. However, the extent and magnitude by which Harmattan alters the functions of baroreceptors is unclear. The aim of this narrative review is to provide insight and highlight the effect of Harmattan exposure on baroreceptors.
Functional anatomy of baroreceptors and baroreflex sensitivity
Baroreceptors are special stretch receptors and free nerve endings that keep the medulla apprised of cardiac cycle-related distension. Like other phasic receptors, baroreceptors can adapt to mechanical stimuli rapidly and the narrowing of the carotid sinus and aortic arc makes baroreceptors discharge less. In the arterial vasculature most especially in the carotid sinus, aortic arc, and cardiopulmonary system, they function to maintain the blood volume and mean arterial blood pressure [16]. Within the relatively confined regions of the carotid sinus and aortic arc are the high-pressure baroreceptors that monitor changes in blood pressure in the internal carotid artery and aorta [21–23]. Low-pressure baroreceptors reside within the atria, ventricles, large systemic veins, and pulmonary blood vessels where they regulate the blood volume [19, 24]. Activation of both high- and low-pressure baroreceptors results in alterations in total peripheral resistance, mean arterial pressure, heart rate, and ventricular contractility in a negative feedback pattern [19, 20, 25].
Baroreflex sensitivity (BRS) is a measure of the autonomic effector response to a given change in arterial pressure [26]. Baroreflex resetting is a phenomenon in which baroreceptors adjust their pressure threshold to maintain a new mean arterial blood pressure at a level [27]. In hypertension, baroreceptors reset their operational range to regulate high blood pressure making them adaptive to blood pressure changes [28]. Interestingly, baroreflex resetting is not only associated with hypertension and other pathological conditions. It occurs in many physiological situations where reversible maintenance of high or low blood pressure is needed, examples include exercise and pregnancy [29, 30]. Notably, the cardiovascular control pathways operate in such a way that a greater fall in heart rate to a given pressure elevation is indicative of a higher BRS and vice versa [26]. This is because activation of high-pressure baroreceptors by distension of the carotid sinus and aortic arc initiates sensory signals which are conducted respectively by the Hering branch of the glossopharyngeal nerve and depressor branch of the vagus nerve to the nucleus of tractus solitarius (NTS). NTS is an important relay center for many visceral sensations in the medulla. Through the nucleus, sensory signals reach and stimulate the nucleus ambiguous and dorsal motor nucleus [31]. This then leads to a decrease in heart rate. A reduction in BRS may occur in cardiac failure and other cardiovascular complications [32]. Thus, understanding the interplay between blood pressure, heart rate, and BRS may serve as a useful guide in assessing health status, diagnosis, and prognosis of cardiovascular-related complications [33–35].
Carotid baroreceptors
High-pressure baroreceptors are activated by stretching of the carotid sinus. Carotid sinus is a bunch of neurovascular structures that present as a dilated region in the tunica externa of the internal carotid artery near the bifurcation of the common carotid artery [23]. Their cell bodies are in petrosal ganglion [18]. Carotid baroreceptors are stimulated at a pressure threshold ranging from mean arterial blood pressure of 60 mmHg to 180 mmHg [20]. Since the internal carotid artery supplies the brain, carotid baroreceptors are involved in the control of blood pressure and blood flow to the brain [36]. Stimulation of carotid baroreceptors creates receptor potential which then develops into action potential through summation [18, 37]. Hering branch of the glossopharyngeal nerve conducts impulses from carotid baroreceptors to the NTS of the medulla, a relay center for high-pressure baroreceptors [31]. Myelinated A-delta and unmyelinated C-fibers are respectively involved in the dynamic and tonic regulation of diastolic blood pressure and heart rate [36, 38]. From the NTS, electrical signals are transmitted to both the cardiac center (nucleus ambiguuus and dorsal motor nucleus) and the caudal ventrolateral medulla [36]. The nucleus ambiguus and dorsal motor nucleus are excited causing inhibitory discharge to the heart. Stimulation of the caudal ventrolateral medulla results in inhibition of the rostral ventrolateral medulla (the vasomotor center) and inhibition of the tonic stimulation of the sympathetic chain [39]. The outcomes of stimulation of carotid baroreceptors are vasodilation, a decrease in diastolic blood pressure, and a decrease in heart rate [16] as shown in Figure 1.
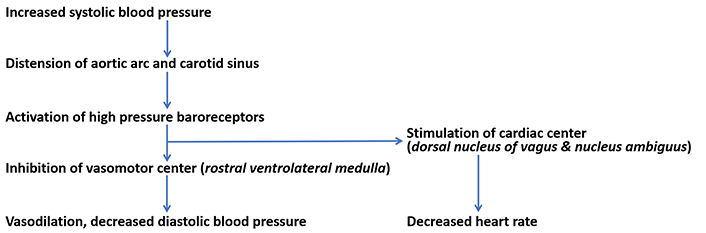
Reflex regulation of blood pressure. Increased systolic blood pressure stimulates high-pressure baroreceptors to cause a reflex reduction in diastolic blood pressure and heart rate
Aortic baroreceptors
Aortic baroreceptors are found in the tunica externa of an aortic arc with their cell bodies situated in the nodose ganglion [20, 21]. There is controversy around the higher sensitivity of aortic baroreceptors over carotid baroreceptors in regulating certain cardiovascular functions, however, emerging evidence from studies showed aortic baroreceptors exert a dominant role in the control of systemic blood pressure and heart rate control over carotid baroreceptors [18, 40]. Impulses generated by aortic baroreceptors are conducted via the aortic depressor nerve (AND), a branch of the vagus nerve that is transmitted through the NTS to both the cardiac centers where efferent parasympathetic and sympathetic activities are adjusted to compensate for the change in systemic blood pressure [20, 40, 41].
Low-pressure or cardiopulmonary baroreceptors
The confinement of cardiopulmonary baroreceptors within the atria, ventricles, pulmonary blood vessels, and large systemic veins is also crucial in maintaining resting blood pressure and total peripheral resistance [42]. For example, an increase in right atrial filling during inspiration causes the low-pressure baroreceptors to be stimulated, which raises the heart rate. This reaction is called the Bainbridge reflex [20]. Moreso, their stimulation generates electric signals which are conducted via afferent division of the vagus nerve to the NTS where integration takes place. In fact, recent evidence from heart transplantation suggests that the denervation of afferent connections from low-pressure cardiopulmonary baroreceptors might contribute to elevated resting blood pressures and total peripheral resistance, as well as blunted orthostatic responses [43]. Thus, understanding how environmental changes like Harmattan may alter the functional role of cardiopulmonary baroreceptors may provide insight and guidance in the prevention and management of cardiovascular-related events among susceptible cohorts.
Overview effect of Sub-Saharan Harmattan on general health
In Sub-Sahara Africa, Harmattan experience is an inevitable seasonal phenomenon and the severity of Harmattan varies from one region to another [44]. Despite the inevitability of the Harmattan wind, there are insufficient studies from West African origin that have investigated the impacts of Harmattan season on human health most especially the baroreflex.
Notably, in the Northern region of Nigeria, the rate of deposition of the Harmattan wind was estimated as 99 g/m2 per season [45]. In the mineralogical analysis of Harmattan dust in Kano, Northern region of Nigeria, Wilke et al. [45] showed quartz (80 wt%) was highly predominant in the sample. Quartz has long been known to be made up of silicon [46]. The dust was also shown to contain higher levels of phosphorus, nitrogen, organic carbon, and trace elements than soils. Chemicals such as aluminum, selenium, chromium, tin, manganese, gold, zinc, chloride, barium, and many others may be found in Harmattan wind depending on industrial activities, natural and human activities.
Sahara dusts are vehicles for a barrage of microorganisms and infectious agents [47]. According to the study by Vergadi et al. [47], several hosts of infectious disease outbreaks such as pneumonia, respiratory tract infections, COVID-19, pulmonary tuberculosis, and coccidioidomycosis were significantly associated with a high incidence of dust events in certain geographical areas. Furthermore, a study by Okeahialam et al. [48] also showed that the Northeast dust-clouded wind in Nigeria causes air pollution and results in a plethora of adverse health outcomes such as cardiovascular diseases and cardiopulmonary disorders among others.
It is noteworthy that there is conflicting evidence on how the size of dust PMs exposure influences certain health risks. For instance, a previous study by McElroy et al. [49] highlights a relationship between the size of dust PMs and respiratory anomalies in children. In fact, the outcome of the study showed children who dwell in rural communities and people with lower income demonstrated a higher risk of respiratory problems when exposed to dust in Benin [49]. More so, previous studies also show that dust particles with an average size of 10 μm were associated with an increase in respiratory, cardiovascular, and diabetes mortality. In fact, when the level of dust particles with a size of 10 μm was greater than 40 μg/m3, there was an increase in respiratory and cardiovascular mortality by 12.6% and 9.7% respectively [50, 51]. In contrast, other studies from Europe reported no specific association between PM2.5 and cause-specific daily mortality during Sahara dust exposure [52, 53]. However, the mechanisms through which the small particulate dusts caused mortality are unclear.
Impacts of ultrafine Harmattan dust particles on baroreceptors
Over the years, the outcome of a handful of studies that have delved into how ultrafine particles impact BRS are conflicting. Briefly, when a portion of ultrafine dust particles are easily absorbed by pulmonary capillaries they are filtered into the circulation where they are transported to body tissues including the interior lining of the viscera and tunica intima of the blood vessels [53]. The presence of ultrafine dust particles in the endocardium and the tunica intima of blood vessels impairs blood flow patterns, causing turbulence and increasing the likelihood of tissue damage, paralysis, and stroke [53]. The potential mechanisms by which ultrafine particles from Harmattan dust disrupt alter BRS are associated with an increased release of free radicals as well as changes in neural activity direct interaction with blood components, particularly platelets [54, 55]. Furthermore, evidence from canine studies suggests that exposure to ambient PM increases BRS to compensate for the particle-induced alterations in blood hemodynamics where there was increased systolic blood pressure by about 2.7 mmHg, diastolic blood pressure by 4.1 mmHg and heart rate by 1.6 bpm [56]. In contrast, experimental studies in rats showed exposure to PM (0.4–0.5 μm) altered BRS leading to an increased change in systolic and diastolic blood pressure in healthy Wistar Kyoto rats [57]. On the other hand, Carll et al. [58] showed that inhalation of PM2.5 by normal diet-fed rats, exerted no major effect on BRS when compared with high-fat fed rats that showed a reduction in BRS, heart rate variability (HRV), and cardiovascular-vagal influences. A recent meta-analysis also showed decreased HRV levels during short-term exposure to PM2.5 [59]. Briefly, HRV is the variation in the intervals between successive heartbeats that indicate vascular tone and autonomic regulation [60, 61]. While it is unclear how PMs cause HRV abnormalities, evidence suggests that transient receptor potential vanilloid receptor 1 (TRPV1) plays a role in PM-induced cardiac electrophysiological changes [62]. In fact, reduced HRV is regarded as a strong predictor for the prognosis and incidence of ventricular arrhythmia [63].
Emerging evidence has also highlighted the role of pro-inflammatory and prooxidative mediators on cardiac autonomic dysfunction upon PM exposure [55, 64]. They exert some pathological changes that contribute to cardiorespiratory mortality and morbidity [52, 65, 66]. For instance, an experimental study by Chiarella et al. [55] demonstrated autonomic nervous dysfunction in mice inhaling PM2.5 which is associated with an increased-levels of circulating catecholamine, activation of the sympathetic nervous system, increased release of proinflammatory cytokines (IL-6) and the development of a hypercoagulable state.
In elderly people, Liu et al. [67] demonstrated the effect of small PM2.5 was associated with elevated changes in heart rate, blood pressure, thiobarbituric acid and endothelin-1 (ET-1) and vascular endothelial growth factor, and lower brachial artery diameter. Moreover, data from the population-based atherosclerosis risk in communities study showed a reduction in autonomic cardiovascular control among persons exposed to air pollutants (10 μm) with a larger impairment in high-risk individuals [68].
Epigenetic modification of cardiovascular functions due to PM exposure
Studies have highlighted the role of microRNAs (miRNAs) as a possible mechanism to elucidate the impact of PM exposures on the cardiovascular system [69, 70]. Briefly, miRNAs are a class of small noncoding RNAs that regulate various cellular activities including cell growth, differentiation, development, and apoptosis [71]. In fact, clinical and experimental studies showed alterations in miRNAs upon PM exposure increases the risk of endothelial dysfunction, atherosclerosis, and systemic inflammation [70, 72, 73]. For instance, ambient PM2.5 exposures were associated with increased levels of extracellular vesicle (EV) miRNAs in the serum of older individuals. Furthermore, in silico pathway analysis on PM2.5-associated EVmiRNAs identified several key cardiovascular disease-related pathways including oxidative stress, inflammation, and atherosclerosis [70].
Effect of silicon dioxide (quartz) on baroreceptors
Notably, evidence regarding the effects of silicon dioxide nanoparticles on baroreceptors is scanty. Silicon is generally known to be non-toxic when taken orally and thus has been utilized in biomedicine, foods, drug delivery, and bone repairs. However, at a nano dimension, the tendency of bioaccumulation is high thus tending towards silicosis and other adversities [74]. In fact, emerging evidence suggests that increased exposure to silicon dioxide nanoparticles can impair the functions of cardiomyocytes [75]. In the study by Guerrero-Beltrán et al. [75], silicate nanoparticle administration in a dose-related pattern impaired contractility and intracellular Ca2+ transient amplitude during β-adrenergic stimulation. Furthermore, SiO2 treatment depolarized the mitochondrial membrane potential and decreased ATP production by 55%. Notable glutathione depletion and H2O2 generation were also reported. The mechanism behind SiO2 cardiotoxicity is due to increased oxidative stress which is linked to a higher risk of heart failure, arrhythmias, and sudden death. More so, a study by Lozano et al. [76] that investigated the effect of amorphous silicate nanoparticles on cardiac muscle cells showed increased dose of silicate nanoparticles led to an increased lactate dehydrogenase and necrosis of cardiomyocytes respectively.
Effect of non-essential heavy metal from Harmattan wind on baroreceptors
Non-essential heavy metals are naturally occurring elements having higher relative densities and they include cadmium, lead, mercury, chromium, arsenic, and many more [77]. Due to their heaviness, they tend to be insoluble as well as non-biodegradable. As a result of their non-degradability, they accumulate in soft body tissues including blood vessels and the heart, and promote oxidative stress-induced tissue damage [35]. For instance, when cadmium was administered to rats at an oral dose not less than 150 mg/kg there was a reduction in BRS following three and seven days of phenylephrine loading [78]. However, on the fourteenth day, there was a restoration of BRS. Thus, this suggests that cadmium modulated baroreflex regulation of the heart rate by disrupting the sensory arm of the baroreflex in a dose and duration-dependent pattern. More studies are needed to clarify whether prolonged exposure to cadmium elicits any adaptive responses.
Furthermore, a study by Sancini et al. [79] showed daily exposure to urban pollutants including cadmium is associated with changes in blood pressure. Through multiple linear regression analysis, non-smoking outdoor workers who were occupationally exposed to cadmium were shown to exhibit a significant reduction in the orthostatic diastolic blood pressure response. One of the possible mechanisms by which cadmium exposure elevated pressor activity by suppressing the bioavailability of nitric oxide through enhanced production of reactive oxygen species by nicotinamide adenine dinucleotide phosphate oxidase, a membrane-oriented enzyme presented in the cell membranes of body cells [80].
Emerging evidence has also highlighted the detrimental effect of lead exposure in experimental and human studies. For instance, a study by Geraldes et al. [81] showed baroreceptor reflex impairment, elevated chemosensitivity, sympathetic overexcitation, hypertension, and tachypnea during lead exposure from the fetal stage to adulthood in rats. In fact, the administration of angiotensin (1–7) antagonist (A-779) was shown to reverse these anomalies [81]. Furthermore, lead acetate treatment in Wistar rats for a period of thirty days leads to reduced BRS and sympathovagal imbalance in a dose-dependent manner [82]. Previous studies involving human participants who were occupationally exposed to lead also demonstrated lower heart rate recovery when compared to non-exposed subjects during exercise testing and transthoracic echocardiography. In fact, a positive correlation was found between 24-hour urine level and blood lead level and heart rate recovery at the first minute [83]. In another study by Minigaliyeva et al. [84], male albino rats that were exposed to lead oxide nanoparticles (49.6 ± 16.0 nm in size) sub-chronically demonstrated impaired cardiomyocyte contractile function due to increased oxidative stress damage as well as changes in blood pressure parameters. Lead oxide also caused anemia, hypocalcemia, and hypoxia in the rats. A study by Yilmaz et al. [85] showed individuals exposed to mercury demonstrated reduced heart rate recovery when compared to non-exposed healthy subjects. Moreso, Liu et al. [86] reported an association between combined high blood lead and mercury levels with reduced resting heart rate in 532 young male adults. Thus, exposure to toxic metals resulting in a low resting heart rate may increase the risk of baroreflex dysfunction (Figure 2) [86].
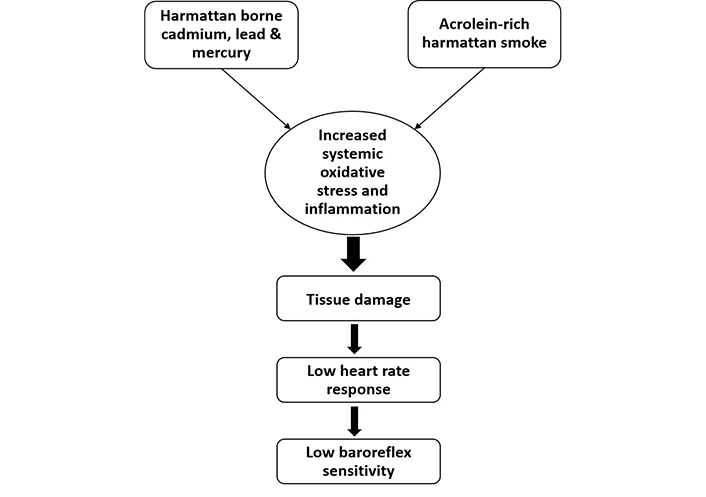
Effect of Harmattan wind on baroreflex sensitivity. Acrolein-containing Harmattan smoke as well as non-essential heavy metals such as cadmium, lead, and mercury caused a decrease in heart rate response culminating into impairment of baroreflex sensitivity
Effect of Harmattan smokes on baroreceptors
Smokes are suspensions in the air made up of a complex cocktail of gaseous chemicals such as carbon monoxide, nitrogen oxides, hydrogen cyanides, polycyclic aromatic hydrocarbons, benzenes, formaldehyde, gases, N-nitrosamines, non-essential heavy metals and acrolein which are emitted from the combustion of substances such as woods, charcoal, tobacco, and cigarette [87]. In fact, evidence suggests a deleterious effect of acrolein on cardiovascular function and BRS. A study by Lozano et al. [76] showed acrolein led to a reduction in BRS and an increase in arrhythmia incidence in Wistar Kyoto rats and spontaneous hypertensive rats.
Notably, the production of smoke is predominant in environments where there are weak policies and implementation regarding control of air pollution [88]. Data published by the Africa Health Organization in 2019 indicated a rise in tobacco smoke exposure between the years 2000 and 2020 in some selected countries [89]. The fact that Harmattan wind is typically dry and sub-humid also increases the burning tendencies and smoke production. The concentration and composition of smoke may vary, and it depends on human activities, natural resources, industrialization, and the extent of air pollution control. Exhausts and petroleum-derived particles are another example of air pollutants that may modulate autonomic cardiovascular control. In a study by Gerhardt et al. [90], 24 smokers consisting of twelve males and twelve females who smoked at least 10 cigarettes per day and for six years demonstrated an increase in heart rate and blood pressure when compared with non-smokers after twenty-four hours. In fact, BRS was glaringly lower in acute tobacco smokers when compared with non-smokers. There was a rise in HRV following smoking as well as an elevated mean arterial blood pressure [91].
Furthermore, a study by Arosio et al. [92], showed an increase in heart rate as well as elevated systolic and diastolic blood pressure among acute smokers when compared with non-smokers and this was associated with impaired BRS. Alteration of sympathetic and vagal neural cardiovascular outflows was also reported among habitual smokers. Arterial pressure was found to increase while RR interval and muscle sympathetic nerve activity decreased. There was also a total resetting of the carotid baroreceptor reflex [93]. In contrast, Cui et al. [94] reported no significant change in BRS among smokers when compared with non-smokers. Although there was an increase in heart rate, mean arterial blood pressure, and muscle sympathetic nervous activity among the smokers in comparison to the non-smokers [94].
Effect of Harmattan cold on baroreflex
Harmattan cold may elicit responses like the cardiovascular changes widely reported with cold pressor. Cold induces local spinal reflex resulting in an elevated peripheral vascular resistance and diastolic blood pressure. Decreased blood flow occasioned by raised diastolic blood pressure elicits renin secretion formation of angiotensin II and secretion of aldosterone; all causing extracellular fluid volume, heart rate, and systolic blood pressure to increase. Although reversible in normotensive people, massive increases in diastolic and systolic blood pressure are seen in hypertensive patients owing to their high resting vascular tone. As baroreceptors respond acutely to blood pressure changes, their role in the control of blood pressure during a cold challenge is short-lived. However, they may be involved in the acute recovery of blood pressure and heart rate following the cessation of cold challenge and other sympathetic situations including anxiety, fear, and exercise. In a study by Cui et al. [95], sodium nitroprusside and phenylephrine were infused into subjects where a decrease in blood pressure was observed during the second while an increase was reported in the third minute of cold pressor challenge, however, the baroreceptor activities were not disrupted during cold challenge. Pikkarainen et al. [96] evaluated BRS after acute upper body exercise in patients with stable coronary artery disease under ambient cold. Although there was no attenuation of systolic blood pressure but there was elevated post-exercise BRS and overall vagal activity [96].
Emerging evidence from a retrospective study of 203,703 patients in three hospitals in Germany between 2010 and 2018 showed prolonged exposure to cold led to increased hospitalization of 7,362 patients for hypertensive disease. The regression analysis also showed an association between decreased level of maximal temperature by 5°C and a 3% increase in risk for admission for hypertension and vice versa [7]. Another recent meta-analysis found that participants’ blood pressure increased significantly during exposure to cold, while during the hot season, their average blood pressure decreased by about 5/3 (systolic/diastolic blood pressure) mmHg. This decline appears to be higher in treated hypertensive patients and older people [97]. The resultant increased blood pressure during severe cold exposure may induce stroke and myocardial infarction in high-risk patients [98, 99]. In fact, a study by Tian et al. [100] showed that moderate cold weather is associated with a considerable attributable risk for cardiovascular diseases.
The potential mechanism involved in hemodynamic alterations during cold exposure is attributed to thermoregulatory vasoconstriction, which aims to maintain core temperature and decrease the skin’s blood flow [101, 102]. Of note, cold-induced vasoconstriction is an autonomic response that influences the cardiac afterload, total peripheral resistance, blood pressure, cardiac output (CO), and stroke volume [103]. An increased sympathetic nerve activity during cold exposure can lead to an imbalance in the release of vasoactive and vasodilatory metabolites such as ET-1 and nitric oxide from the endothelium resulting in vasoconstriction [104, 105]. The resultant vasoconstriction alters the microvascular environment and reduces shear stress [106, 107]. In fact, evidence suggests a reduction in flow-mediated diameter (FMD) and hyperaemic flow velocity during cold exposure in humans [108]. High levels of circulating ET-1 also promote sympathetic nerve activity 1 and increase blood pressure via endothelin receptor type-A study in rats [105].
Additional mechanistic evidence associated with cold-induced hemodynamic modification is linked to an increased activity of the renin-angiotensin-aldosterone system (RAAS) [109]. A previous study showed that rats exposed to prolonged cold demonstrated elevated RAAS activity along with concurrent activation of brain angiotensin II type 1 (AT1) receptors [109]. The upregulation of certain intracellular signaling such as the Rho-kinase (ROCK) pathway is also linked to cold-induced vasoconstriction in people with essential hypertension [110]. The vasoconstrictive effect of ROCK upregulation is attributed to an age-associated increase in oxidative stress and angiotensin II signaling [111]. It is unclear if mechanistic variability exists among different age groups that are exposed to prolonged Harmattan season. Cardiovascular regulatory mechanisms to cold are unique in that they interpret the narrowing of arterial vasculature as an index of hypoperfusion, hypovolemia, and hypotension and arterial dilation as an indication of hypertension and hypervolemia. They are also not efficient enough. Hence, there are need for intentional heat gain strategies to complement intrinsic heat gain mechanisms. Smoking alcoholics, dietary fat, and salt intake, as well as sedentary behavior and other phenomena that might narrow arterial vasculatures must be avoided during Harmattan.
Conclusions
Baroreceptors play critical roles in the short-term responses to blood pressure perturbation and are therefore essential for acute restoration of blood pressure following cold exposure. Chilly Harmattan wind orchestrates duration-dependent perturbation in blood pressure causing remarkable alterations in the cardiorespiratory system in apparently healthy individuals while exaggerating the risks of hospitalization in typically vulnerable individuals. This phenomenon has been associated with various health implications, including potential impacts on baroreceptor sensitivity, blood pressure regulation, and other autonomic functions. Prolonged exposure leads to alterations in baroreceptor sensitivity, influencing the body’s ability to regulate blood pressure in response to changes in blood volume and vascular tone. Furthermore, the presence of PMs, acrolein-rich smokes and non-essential heavy metals such as lead, mercury, and cadmium in Harmattan wind may also result in a reduction in BRS. Regular use of protective masks to reduce inhalation of deleterious Harmattan wind substances must be encouraged to improve health outcomes in patients and apparently healthy individuals. Policies geared towards regulating air pollution are needed to protect vulnerable patients.
Future perspective
Intensive primary studies are needed to clarify the impact of different Harmattan dust sizes on baroreceptors and BRS. Primary studies from the Sub-Sahara zone regarding the system and multisystem impact of Harmattan wind are most needed. Thus, understanding the mechanism underlining autonomic dysfunction during exposure to Harmattan season may help provide insight and guidance toward a multilevel focus on the therapeutic intervention needed to prevent and manage cardiovascular-related events in different age groups and higher-risk patients.
Abbreviations
BRS: | baroreflex sensitivity |
ET-1: | endothelin-1 |
HRV: | heart rate variability |
miRNAs: | microRNAs |
NTS: | nucleus of tractus solitarius |
PM: | particulate matter |
Declarations
Author contributions
MJA: Conceptualization, Writing—review & editing. OAF: Writing—review & editing. AA: Methodology, Writing—review & editing.
Conflicts of interest
In compliance with the ICMJE uniform disclosure form, all authors declare the following: No funds, grants, or other support was received. The authors have no relevant affiliations or financial involvement with any organization or entity with a financial interest in or financial conflict with the subject matter or materials discussed in the manuscript. This includes employment, consultancies, honoraria, stock ownership or options, expert testimony, grants or patents received or pending, or royalties. The authors declare that they have no conflicts of interest.
Ethical approval
Not applicable.
Consent to participate
Not applicable.
Consent to publication
Not applicable.
Availability of data and materials
Not applicable.
Funding
Not applicable.
Copyright
© The Author(s) 2024.