Abstract
Aim:
Loperamide hydrochloride (HCl), a peripheral opioid receptor agonist primarily used as an antidiarrheal drug, has been reported to induce apoptosis in various cancer cell lines. However, its effects on oral cancer cells are yet to be ascertained. This study investigates the cytotoxic effects of loperamide HCl on oral squamous cell carcinoma (OSCC) cells, exploring its potential as a repurposed therapeutic agent.
Methods:
The OECM-1 OSCC cells were treated with various concentrations of loperamide HCl. The metabolic alterations of OECM-1 cells were spectrophotometrically assessed at 4, 24, 48, and 72 hours using the alamarBlue cell proliferation assay. The half-inhibitory concentration (IC50) of loperamide HCl on OECM-1 cells was calculated through non-linear regression analysis. Apoptosis was evaluated using the Caspase-3/7 assay.
Results:
Loperamide HCl suppressed OECM-1 cell proliferation after 24 hours. The IC50 values of loperamide HCl on OECM-1 cells were non-evaluable at 4 hours but progressively decreased to 80.82 µM at 24 hours, 37.69 µM at 48 hours, and 34.29 µM at 72 hours. Significant apoptosis was observed at loperamide HCl concentrations of 40 µM and 50 µM after 72 hours of treatment.
Conclusions:
Loperamide HCl demonstrates a time- and dose-dependent cytotoxic effect on OECM-1 cells, significantly inhibiting proliferation and inducing apoptosis at higher concentrations. The decreasing IC50 values over time indicate enhanced cytotoxicity with prolonged exposure. These findings suggest loperamide HCl’s potential as a therapeutic agent for OSCC.
Keywords
Oral cancer, apoptosis, opiate agonist, loperamide hydrochlorideIntroduction
Despite significant advancements in the prevention, early detection, and treatment of oral cancer, the disease remains a leading cause of mortality worldwide [1]. While research has led to notable improvements in therapeutic strategies [2, 3], the complexity of cancer biology and the substantial financial burden associated with new drug development continue to hinder the pace of progress. Consequently, there remains an urgent need for more effective and accessible oral cancer therapies.
Loperamide hydrochloride (HCl) (N-dimethyl-α,α-diphenyl-4-(right-chlorophenyl)-4-hydroxyl-1-piperidines butanamide hydrochloride), a peripheral opiate agonist commonly used as an antidiarrheal drug, has demonstrated cytotoxic effects across a wide range of cancer cell lines, including human breast, renal, bladder, gastric, liver, lung, melanoma, leukemia, osteosarcoma, and even canine cancer cells. Studies indicate that it induces apoptosis, cytotoxicity, and DNA damage in a time- and dose-dependent manner [4–8]. Moreover, loperamide HCl acts as a substrate for P-glycoprotein, an ATP-dependent efflux membrane transporter involved in multidrug resistance. This feature allows it to reverse doxorubicin resistance in breast cancer cells by competing with P-glycoprotein, thereby increasing the intracellular concentration of chemotherapy drugs [9]. Its potential as an anti-cancer agent is highlighted by its accessibility, minimal side effects, low risk of allergic reactions, and most importantly, its affordability. Investigating loperamide HCl as a therapeutic option offers a promising direction in oral cancer treatment, especially when repurposing an existing drug with well-established pharmacology, pharmacokinetics, and toxicity profiles. This approach is both cost-effective and time-efficient compared to traditional drug discovery efforts. Other opiate agonists, such as morphine, D,L-methadone, and noscapine, have shown anticancer effects, including apoptosis induction and overcoming treatment resistance [10–12]. This strengthens the rationale for exploring loperamide HCl and related compounds in oral cancer therapies.
Oral squamous cell carcinoma (OSCC) accounts for approximately 90% of oral cancers, which constitute the majority of head and neck cancers. The oral cavity has a unique microenvironment, characterized by its complex tissue composition, unique microbial flora, and frequent exposure to chronic friction/irritation, as well as its strong association with carcinogens, particularly tobacco. This distinctiveness of the oral cavity and its cells is further highlighted by the specific interactions between the oral microbiome and diseases such as oral cancer. The oral microbiome is one of the most diverse in the human body, with over 700 species identified, including bacteria, viruses, fungi, and other microorganisms [13–15]. Additionally, comparative studies show that the oral microbiome clusters distinctly from other body site microbiomes, suggesting a unique microbial community structure [16]. Anatomical features create a complex environment that supports a unique microbiome, which plays a role in oral health and disease [13]. The oral microbiome is implicated in oral diseases, including oral cancer, where specific bacterial communities are associated with tumor tissues [17, 18]. Studies have shown that microbial dysbiosis in the oral cavity can contribute to the progression of oral cancers, highlighting the functional significance of the oral microbiome [18]. While the oral cavity’s microbiome is unique, it is important to consider that other body sites also have specialized microbiomes adapted to their specific environments. Understanding these unique features can provide insights into their contributions to overall health and disease. These observations raise the question of whether loperamide HCl has similar or differential cytotoxic effects on oral cancer cells compared to other established cell lines. Building on previous findings of loperamide HCl’s cytotoxic and apoptotic effects across a broad range of cancer cell lines, this study hypothesizes that loperamide HCl will exhibit similar effects on OSCC cells. Specifically, we expect loperamide HCl to reduce OSCC cell proliferation by altering cellular metabolism, inducing morphological changes, and triggering apoptosis. Given its well-documented pharmacology and minimal side effect profile, loperamide HCl offers a promising, cost-effective therapeutic option for OSCC treatment through drug repurposing.
Materials and methods
Cell lines and culture conditions
Complete culture media was prepared using 1% Gibco Antibiotic-Antimycotic (Anti-Anti), 20% mesenchymal stem cell fetal bovine serum (MSC FBS), and 88% RPMI-1640 medium with L-glutamine and sodium bicarbonate (ThermoFisher, USA). OECM-1 human OSCC cells were commercially obtained from Sigma Aldrich (MERCK, Germany) and cryopreserved in liquid nitrogen at –160°C. As per the supplier, OECM-1 human OSCC cell line was derived from surgical resection of a primary tumor of a Taiwanese male patient. The cells are negative for mycoplasma contamination and genotyped by STR analysis to verify the unique identity of the cell line. Prior to experiments, cells were thawed in a water bath at 37°C for 60 seconds, centrifuged at 1,000 rpm for 8 minutes, and resuspended in complete culture media. Cells were cultured (Figure 1) at 37°C with 5% CO₂ and sub-cultured every 3 days using 0.1% TrypLE Express and 0.02% EDTA in Dulbecco’s phosphate buffered saline (DPBS). Cell counting was performed using a haemocytometer under brightfield microscopy.
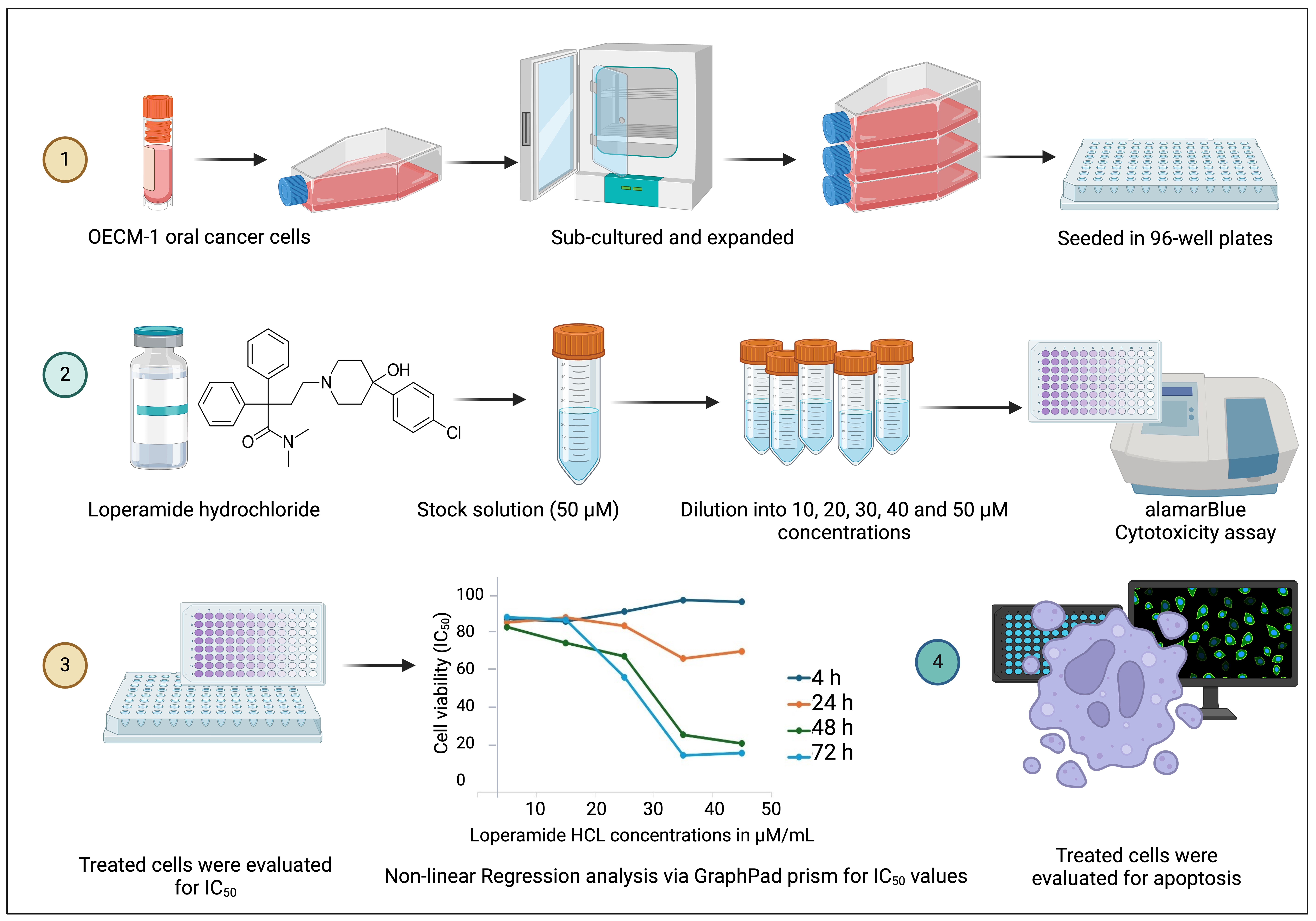
Graphical illustration of methods followed during the experiments. Commercially obtained OECM-1 cells were thawed and sub-cultured to attain sufficient cell numbers. Further, cells were treated with different concentrations of Loperamide HCl and were evaluated for its cytotoxicity, IC50, and ability to induce apoptosis in OECM-1 cells. Created in BioRender. Banavar, S. (2025) https://BioRender.com/m93c993
Cell seeding
OECM-1 cells were seeded at a density of 0.5 × 105 cells per well in 100 µL of complete culture media using a micropipette into 18 wells of a 96-well plate, corresponding to five different concentrations (10–50 µM/mL) of loperamide HCl used in the experiment, with untreated cells serving as controls (Figure 1). Four 96-well plates were prepared for evaluation at 4, 24, 48, and 72 hour intervals. Cells were allowed to adhere for 24 hours. Before treating the cells with loperamide HCl, cell culture supernatants were discarded. All experiments were performed in triplicate.
Preparation of loperamide HCl solution
Loperamide HCl was obtained from Sigma (MERCK, Germany). A total of 1.5 mg of loperamide HCl powder was weighed using an electronic scale and dissolved in 0.5 mL of dimethyl sulfoxide (DMSO, MERCK, Germany) to prepare a 50 µM/mL stock solution. The V1C1 = V2C2 formula was used to calculate the required dilution with culture media, resulting in a final stock concentration of 50 µM/mL. Further dilutions were prepared as follows: 40 µM/mL (8 mL loperamide HCl + 2 mL media), 30 µM/mL (6 mL loperamide HCl + 4 mL media), 20 µM/mL (4 mL loperamide HCl + 6 mL media), and 10 µM/mL (2 mL loperamide HCl + 8 mL media) (Figure 1).
Subsequently, 100 µL of loperamide HCl solution of each concentration was pipetted into wells containing seeded OECM-1 cells. Control wells received culture media without loperamide HCl. Plates were incubated at 37°C with 5% carbon dioxide.
Cell proliferation assay to assess the metabolic alterations of OSCC cells
The cytotoxicity of loperamide HCl or its effect on OECM-1 cell metabolism and proliferation was assessed using the alamarBlue assay (ThermoFisher, New York, USA), a bio-reductive fluorometric method for evaluating cell viability. The alamarBlue reagent undergoes a color change from blue (oxidized, non-fluorescent) to pink (reduced, fluorescent) through an oxidation-reduction mechanism. This shift reflects cellular metabolism, with viable cells reducing the dye to pink, while impaired metabolism in apoptotic cells retains the blue state. The spectrophotometric measurements were taken at 4, 24, 48, and 72 hour intervals post-treatment. The reagent alamarBlue was added to the wells containing treated and control cells and incubated for 6 hours. Fluorescence was then measured using a microplate reader equipped with Infinite 200 PRO software, with excitation and emission wavelengths set at 530 nm and 600 nm, respectively (Figure 1). Following data acquisition, cells were fixed with 4% formaldehyde solution for further analysis. All assays were conducted in triplicates.
Apoptosis assay to assess the apoptotic effects of loperamide HCl solution
Apoptosis was evaluated using the CellEvent Caspase-3/7 detection reagent (ThermoFisher, USA). Cells were treated with various concentrations of loperamide HCl and incubated for 72 hours, a timepoint selected based on optimal anti-proliferative effects observed in our cell proliferation assay (explained later in the Results section). The detection reagent was prepared at 8 µM concentration in a mixture of 5% FBS and 95% DPBS. Following the treatment period, 100 µL of the diluted reagent was added to both treated and control cells and incubated for 30 minutes. Fluorescence intensity was measured using an Infinite 200 PRO spectrophotometer at excitation and emission wavelengths of 502 nm and 530 nm, respectively. Additionally, cellular morphological changes were observed and documented using a Nikon Eclipse Ti-U inverted microscope. All experiments were performed in duplicates (Figure 1).
Determination of IC50 value of loperamide HCl
The half-inhibitory concentration (IC50) of loperamide HCl, defined as the concentration required to induce cytotoxicity in 50% of the OECM-1 cells, was determined at different time points. Further, cytotoxicity was evaluated by testing a range of loperamide HCl concentrations across the specified time intervals. The resulting data were plotted, and a non-linear regression analysis was performed using the GraphPad Prism software.
Statistical analysis
The data were tabulated using Microsoft Excel, and statistical analysis was performed using SPSS version 25. A one-way analysis of variance (ANOVA) was conducted, followed by a post-hoc Tukey test to compare group differences. Statistical significance was set at p < 0.05. All experiments were performed in triplicates.
Results
Cell line and culture conditions
After thawing the cryopreserved vial, OECM-1 cells demonstrated healthy growth with prominent squamoid morphology. The cells were monitored under a brightfield microscope (Figure 2), where colony formation was observed as early as day 5. Confluency was achieved between days 5 and 7, indicating robust cell proliferation under the established culture conditions.
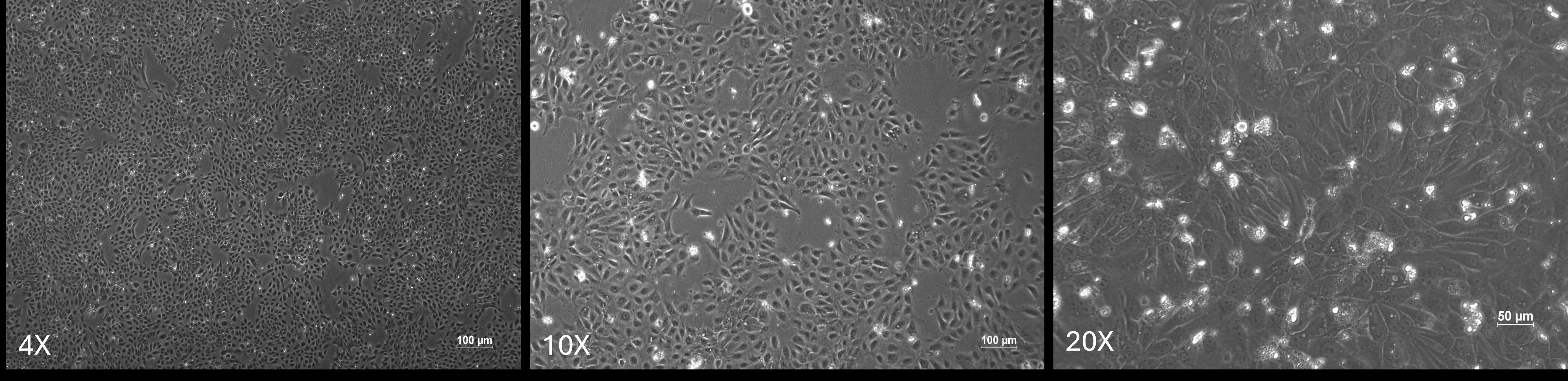
OECM-1 cells visualized at 4×, 10×, and 20× magnification. Inset scale bar is 100 µm for 4× and 10× magnification. For 20×, scale bar is 50 µm
Cytotoxic effect of loperamide HCl
The alamarBlue assay demonstrated that OECM-1 cells treated with higher concentrations of loperamide HCl exhibited a color shift toward purple, indicating reduced cell viability. This shift reflects a lower conversion of resazurin, a blue, redox indicator, to resorufin, a fluorescent red metabolite generated by metabolically active cells. In contrast, cells exposed to lower concentrations of loperamide HCl retained red coloration, suggesting higher metabolic activity and proliferation due to sustained resazurin reduction. Loperamide HCl significantly inhibited OECM-1 cell metabolism and proliferation at higher concentrations i.e., at concentrations from 40 µM/mL compared to the control group (p < 0.05).
The mean values and standard deviations for different concentrations of loperamide HCl across four time intervals were measured and presented graphically (Figure 3). At the 4 hour time point, the mean values ranged from 101.50% to 114.14%, with standard deviations between ±1.12 and ±17.99 for concentrations of 10 to 50 µM/mL. At 24 hours, the mean values ranged from 79.45% to 103.81%, with standard deviations of ±0.37 to ±4.33. At 48 hours, mean values ranged from 28.96% to 98.18%, with standard deviations between ±0.60 and ±4.02. Finally, at 72 hours, the mean values were 104.00%, 102.44%, 68.32%, 21.90%, and 23.30%, with standard deviations ranging from ±0.62 to ±2.67 for increasing concentrations of loperamide. The untreated control cells maintained good viability and proliferation throughout all time points.
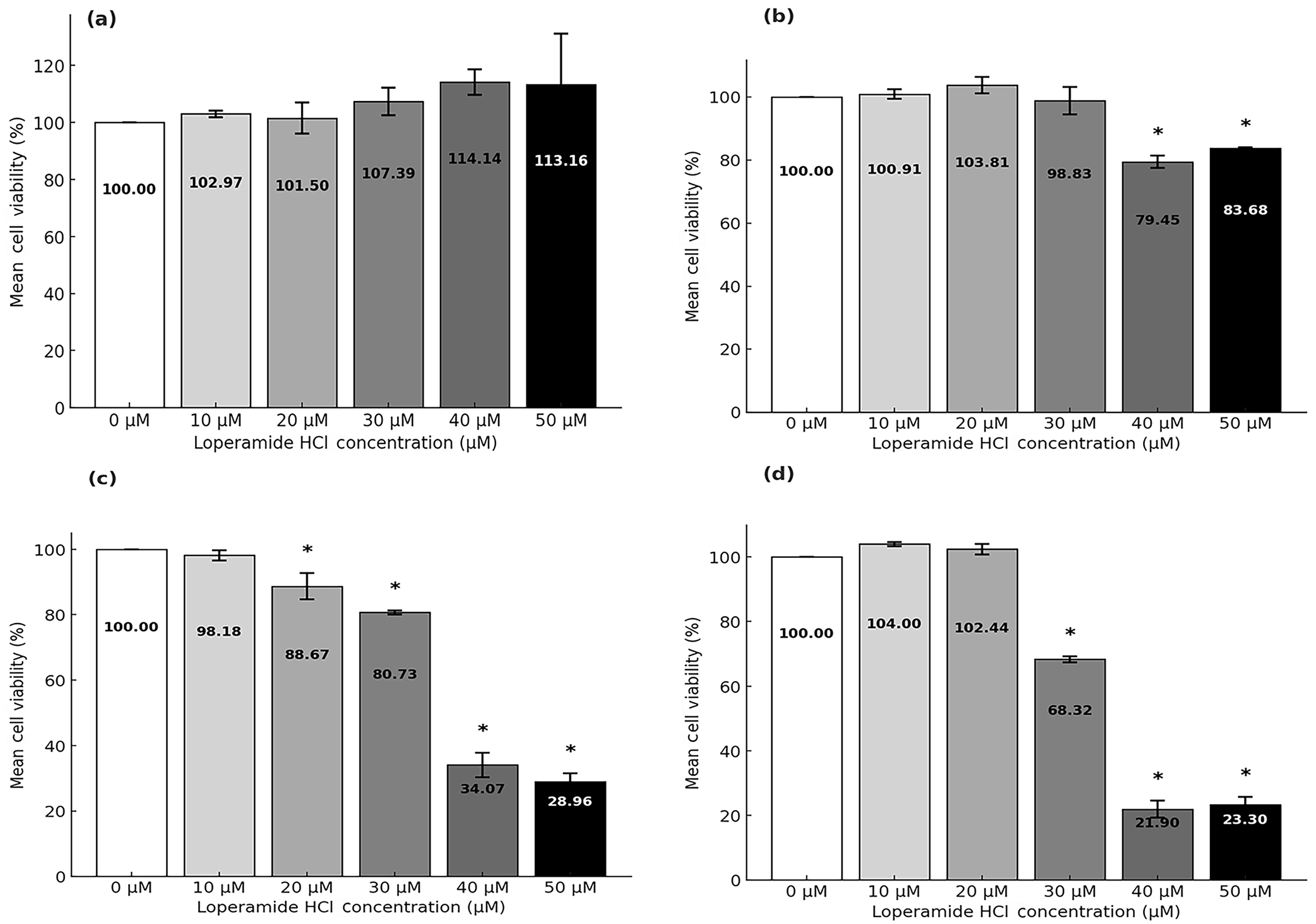
Proliferation analysis of OECM-1 cells treated with loperamide HCl at (a) 4 hours, (b) 24 hours, (c) 48 hours, and (d) 72 hours. Each subfigure represents the mean values of cell viability at the specified time points, with increasing concentrations of loperamide HCl (10 µM–50 µM). Data are presented as mean ± standard deviation. * indicates statistically significant differences, compared with control group
Figure 3a and 3b illustrate cell proliferation at 4 and 24 hours, where no distinct trend was observed. However, at 48 and 72 hours (Figure 3c and 3d), a distinct decline in cell viability was observed. At 48 hours, compared to untreated control group cells, significant reductions in cell proliferation of 66% (100% – 34.07%) and 71% (100% – 28.96%) were observed for 40 µM and 50 µM concentrations, respectively. By 72 hours, OECM-1 cell viability decreased by approximately 78% (100% – 21.9%) and 77% (100% – 23.3%) at these same concentrations.
IC50 value of loperamide HCl against OSCC cells
The IC50 value of loperamide HCl was determined through dose-response curves using non-linear regression analysis in GraphPad Prism software. The IC50 value at 4 hours was non-evaluable or non-convergent. Over time, the IC50 value decreased, indicating that lower concentrations of loperamide HCl were required to induce 50% cytotoxicity in OECM-1 cells as the exposure time increased. The IC50 at 24 hours was 80.82 µM, while at 48 and 72 hours, the values were 37.69 µM and 34.29 µM, respectively (Figure 4).
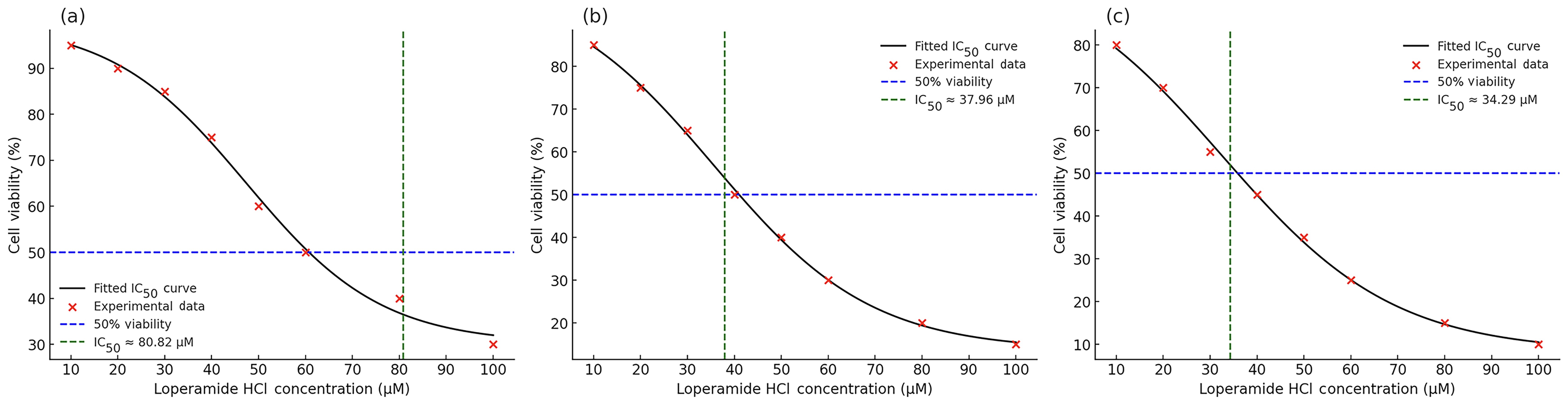
Dose-response curve showing cell viability (%) versus loperamide HCl concentrations at different time points. (a) 24 hours, (b) 48 hours, and (c) 72 hours. Half-inhibitory concentration (IC50) values are interpolated from the graph. Note: the IC50 for 4 hours is not shown in the graph, since it could not be evaluated
Apoptosis detection
Apoptosis in OECM-1 cells was further evaluated using the CellEvent Caspase-3/7 Green Detection Reagent after 72 hours of treatment with 10–50 µM loperamide HCl. Caspase-3/7 activation, a key event in apoptosis, was detected by a DEVD peptide conjugated to a dye with excitation/emission maxima of 502/530 nm. Upon caspase-3/7 activation, the peptide cleaves, allowing the dye to bind to DNA, resulting in green fluorescence, which was observed under a fluorescence microscope (Figure 5).
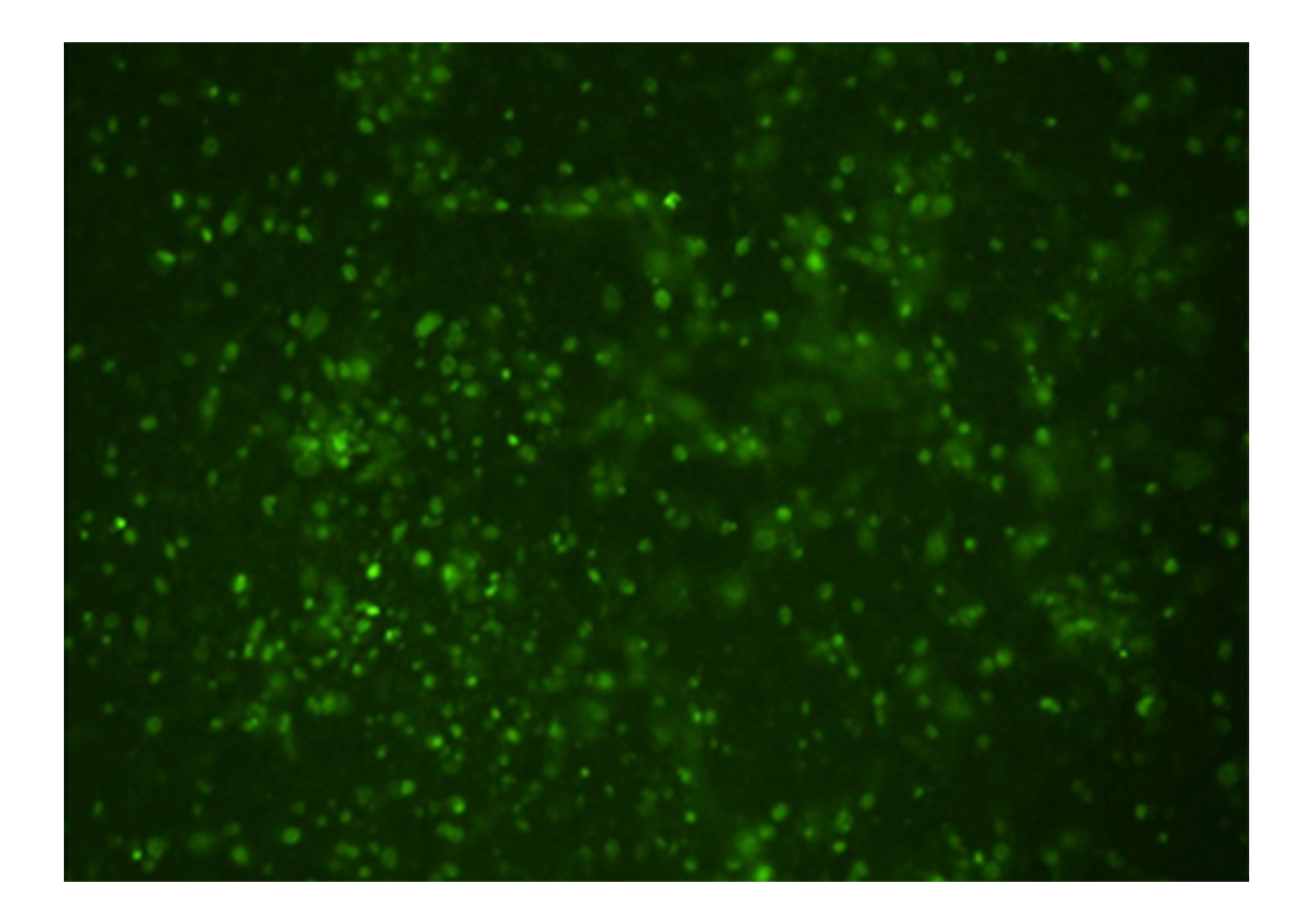
Green fluorescence observed in OECM-1 cells after 72 hours of treatment with 50 µM of loperamide HCl (10× magnification), indicating apoptosis
The fluorescence intensity, which correlates with the level of apoptosis, was quantified using a spectrophotometer. Cells treated with 40 and 50 µM loperamide HCl showed significantly higher levels of apoptosis at 72 hours, with nearly twice the number of apoptotic cells compared to control (Figure 6).
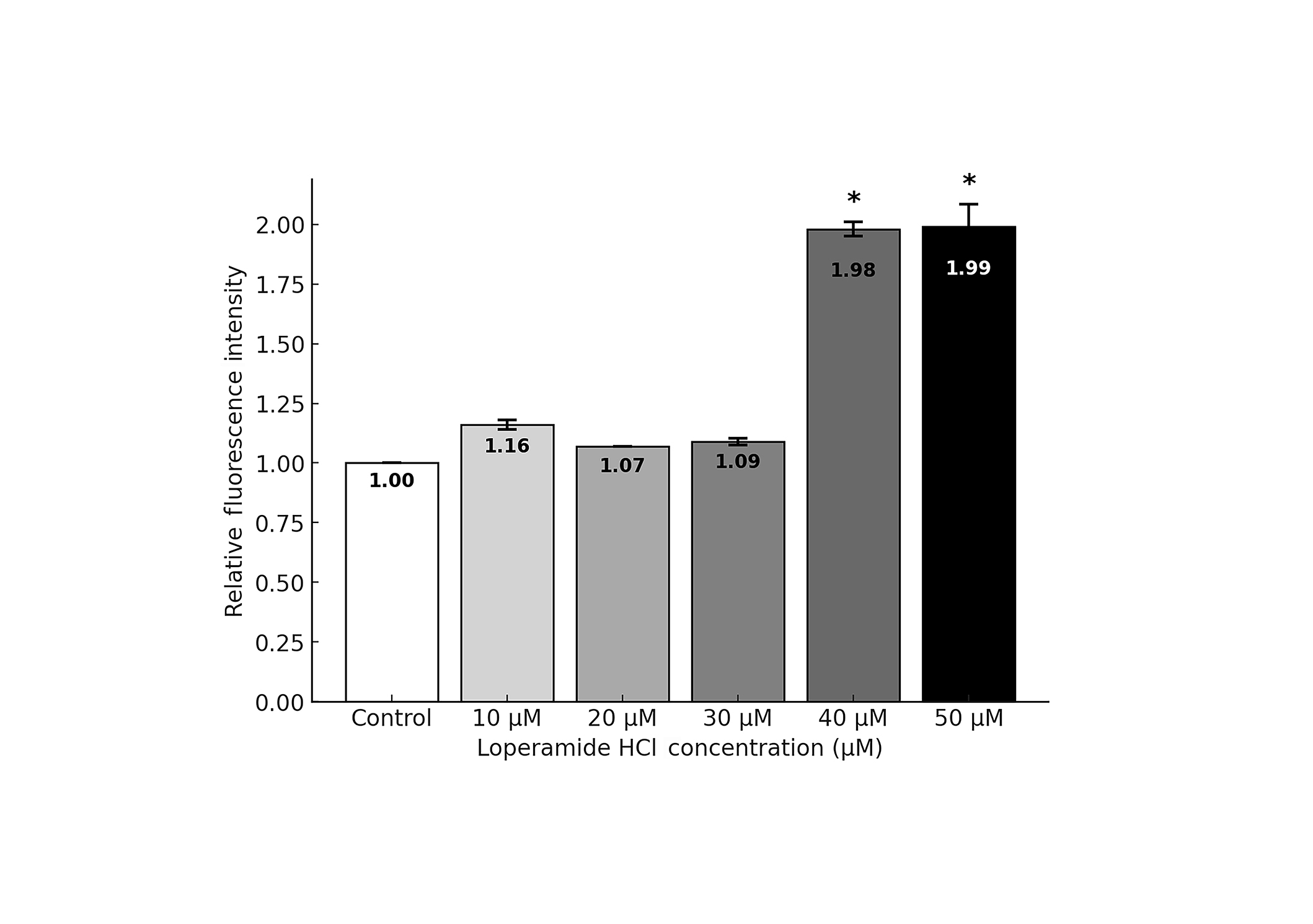
Quantified apoptosis levels in OECM-1 cells treated with loperamide HCl at 72 hours. Data are presented as mean ± standard deviation. * indicates statistically significant differences, compared with control group
OECM-1 cell proliferation
Multiple comparisons using Tukey’s HSD test revealed a time- and concentration-dependent effect of loperamide HCl on OECM-1 cell proliferation. At 4 hours post-treatment, no significant differences in cell proliferation were observed across all loperamide HCl concentrations compared to the control (p > 0.05). By 24 hours, significant reductions in cell proliferation became evident at higher concentrations (40 µM and 50 µM; p < 0.01), while lower concentrations (10–30 µM) showed no significant effect.
The anti-proliferative effect became more pronounced at 48 hours, with significant reductions in cell proliferation observed across a broader range of concentrations. Notably, moderate concentrations (20–30 µM) began showing significant inhibitory effects (p < 0.05), while higher concentrations (40–50 µM) maintained strong anti-proliferative activity (p < 0.01). By 72 hours, the inhibitory effect was further enhanced, with significant reductions in cell proliferation observed at concentrations of 30 µM and above (p < 0.01).
Interestingly, no significant differences were observed between 40 µM and 50 µM concentrations at any time point (24, 48, and 72 hours), suggesting a potential plateau in the anti-proliferative effect at these concentrations.
Apoptosis induction by loperamide HCl
Apoptosis analysis at 72 hours revealed concentration-dependent effects of loperamide HCl. Low to moderate concentrations (10–30 µM) showed minimal impact on apoptosis, with only 10 µM showing a marginal effect (p = 0.006) compared to control. However, higher concentrations demonstrated significant pro-apoptotic activity, with both 40 µM and 50 µM inducing marked increases in apoptosis (p < 0.01). The strongest apoptotic response was observed at 50 µM, showing significant differences compared to all lower concentrations (p < 0.01 vs. 10 µM; p = 0.007 vs. 20 µM; p = 0.003 vs. 30 µM) (Table 1). These findings align with the cell proliferation data, supporting the cytotoxic effect of loperamide HCl at higher concentrations.
Post-hoc analysis for multiple comparisons and their corresponding p-values for apoptosis assay at 72 hours
Loperamide HCl (µM/mL) | 0 | 10 | 20 | 30 | 40 | 50 |
---|---|---|---|---|---|---|
0 | - | 0.006 | 0.354 | 0.204 | 0.990 | 0.204 |
10 | 0.006 | - | 0.175 | 0.309 | 0.002 | < 0.01 |
20 | 0.354 | 0.175 | - | 0.998 | 0.153 | 0.007 |
30 | 0.204 | 0.309 | 0.998 | - | 0.081 | 0.003 |
40 | 0.990 | 0.002 | 0.153 | 0.081 | - | 0.447 |
50 | 0.204 | < 0.01 | 0.007 | 0.003 | 0.447 | - |
-: not applicable
Discussion
This study investigated the cytotoxic and apoptotic effects of loperamide HCl on OECM-1 cells. The rationale for the selected doses of loperamide and the timing was based on its demonstrated cytotoxicity from previous research on other various cancer cells, including canine cancer cells, optimal treatment duration for observing effects, and the drug’s established pharmacological profile. Likewise, OECM-1 oral cancer cells were treated with loperamide for various time points. This timing was crucial to evaluate the dose-dependent effects on cell viability and apoptosis.
Loperamide HCl significantly inhibited the proliferation and induced apoptosis of OECM-1 cells in a dose- and time-dependent manner, with maximal effects observed at concentrations of 40–50 µM, over 48–72 hours. Compared to other cancer cell lines, where significant cytotoxic effects were noted at 25 µM within 24 hours, OSCC cells required higher concentrations and prolonged exposure [8]. This suggests distinct biological characteristics and resistance mechanisms in OSCC cells. These differences may be attributed to factors such as OSCC’s acidic extracellular microenvironment, its direct exposure to external environmental insults, and heightened activity of vacuolar ATPases, all of which collectively hinder drug efficacy [19, 20]. The high prevalence of tobacco and alcohol use among OSCC patients likely contributes to a more resilient cancer phenotype [21]. Additionally, variations in the assays employed or the inherent potency of loperamide HCl on OECM-1 cells could also be contributing factors.
The IC50 values at 4 hours were directly non-evaluable, however, the IC50 values were calculated using nonlinear regression analysis in GraphPad Prism, which fits a dose-response curve to the viability data. Although the maximum concentration tested in this study was 50 µM, the regression model extrapolates the IC50 value based on the trend observed in the experimental data. This approach is commonly used to estimate median lethal dose (LD₅₀) values when the exact point is not directly tested but falls within the curve’s projection. The elevated IC50 values observed in this study demonstrate OECM-1 cells’ robust resistance to loperamide HCl and enhanced survival mechanisms compared to other cancer cells [5]. While no significant reduction in proliferation was observed at 4 hours, IC50 values decreased substantially with prolonged exposure between 24 and 72 hours. Notably, the lack of significant difference between 40 µM and 50 µM, at later time points suggests a potential threshold effect, indicating that optimizing treatment duration may be more critical than dose escalation.
Loperamide HCl demonstrated significant cytotoxic effects and induced apoptosis in OECM-1 cells. Quantitative photo-spectrometry revealed that treated cells exhibited nearly double the fluorescence intensity compared to controls. Initial apoptotic responses were observed at 10 μM, with marked induction occurring at 40–50 μM concentrations after 72 hours of treatment. The mechanism of action appears to operate through caspase-dependent apoptotic pathways, specifically through the activation of caspase-3/7, a crucial mediator of programmed cell death. The findings are particularly significant given that cancer cells typically evade apoptosis. Previous studies have demonstrated that loperamide HCl induces apoptosis in various cancer cells through multiple mechanisms: activation of the ROS/JNK signaling pathway, DNA damage induction via γH2AX expression resulting in DNA fragmentation, and increased expression of cleaved caspase-3 [5–7].
The observed effects of loperamide HCl on OECM-1 cells suggest its potential therapeutic value in OSCC treatment, with mechanisms consistent with its reported effects in other cancer types. These results contribute to our understanding of how pharmacological interventions can restore normal apoptotic responses in cancer cells. This study is limited by the lack of direct comparisons with other oral cancer cell lines and established OSCC treatments, which could provide a broader context for loperamide HCl’s therapeutic potential. Future research should focus on exploring the mechanistic pathways underlying loperamide HCl-induced apoptosis, including mitochondrial involvement and complete caspase signaling cascades. Additionally, in vivo studies are warranted to evaluate its efficacy and safety as an adjuvant or alternative treatment for OSCC.
In conclusion, this study demonstrates the potential of loperamide HCl as a therapeutic agent for OSCC by restoring normal apoptotic responses in oral cancer cells. While its established safety profile and significantly lower cost compared to current chemotherapeutic options make it a promising candidate for drug repurposing, the findings remain preliminary. Additional research is required to fully elucidate its mechanism of action beyond what is currently known, as well as to evaluate its efficacy and safety in preclinical and clinical settings. Further validation studies, including in vivo models and clinical trials, are necessary to confirm its therapeutic potential and establish optimal dosing strategies. A cautious and rigorous approach is essential to ensure its successful repurposing as a treatment for OSCC.
We acknowledge that this study focused solely on oral cancer cells, and additional cytotoxicity testing on non-cancerous cell models, such as human red blood cells or Vero cells, would provide valuable insights into the selective cytotoxicity and safety profile of loperamide HCl. Future research should address these aspects to ensure its safe and effective use in OSCC treatment.
Abbreviations
DPBS: | Dulbecco’s phosphate buffered saline |
FBS: | fetal bovine serum |
HCL: | hydrochloride |
IC50: | half-inhibitory concentration |
OSCC: | oral squamous cell carcinoma |
Declarations
Author contributions
CCT, USLT, and ZXK: Investigation, Writing—original draft. PC: Validation, Conceptualization, Funding acquisition, Writing—review & editing, Supervision. SPK: Writing—review & editing. SRB: Conceptualization, Investigation, Writing—original draft, Writing—review & editing, Supervision. All authors read and approved the submitted version.
Conflicts of interest
The authors declare that they have no conflicts of interest.
Ethical approval
Not applicable.
Consent to participate
Not applicable.
Consent to publication
Not applicable.
Availability of data and materials
All datasets supporting the research findings can be found in the link https://drive.google.com/drive/folders/1DwgE1dBSM4y_nI9E7cj9HT_M7mfjGcEH.
Funding
This research was funded by IMU University, with a reference number of BDS I-01/2021(07), IMU University, Kuala Lumpur, Malaysia. The funder had no role in study design, data collection and analysis, decision to publish, or preparation of the manuscript.
Copyright
© The Author(s) 2025.
Publisher’s note
Open Exploration maintains a neutral stance on jurisdictional claims in published institutional affiliations and maps. All opinions expressed in this article are the personal views of the author(s) and do not represent the stance of the editorial team or the publisher.