Abstract
Aim:
The present study explored the bone regeneration potential of chitosan-thiocolchicoside-lauric (CTL) nanogel using MG-63 cell lines. The cytocompatibility of CTL nanogel was also studied using osteoblast-like cells (MG-63 cell lines) and zebrafish embryos.
Methods:
The effect of CTL nanogel on the metabolic and wound-healing activity of MG-63 cells was investigated in the present study. The alkaline phosphatase (ALP) and bone sialoprotein (BSP) activity of CTL nanogel-treated MG-63 cells were assessed using ELISA. RUNX2, ALP, BSP, and COL1A1 gene expression in MG-63 cells were also investigated after treatment with CTL nanogel. Hatching rates and viability of zebrafish embryos treated with different CTL nanogel concentrations were studied. Any developmental toxicity of embryos after treatment with CTL nanogel was also investigated.
Results:
There was no significant reduction in the proliferation of MG-63 cells when treated with 5–20 μL/mL of CTL nanogel in the MTT assay (p < 0.05). No prominent morphological changes or nuclear abnormalities were found in the MG-63 cells when treated with various concentrations of CTL nanogel compared to the control group. Invitro scratch wound healing assay showed excellent migration of cells and, hence, showed the excellent wound healing ability of CTL nanogel. ELISA showed significant ALP and BSP activity of CTL nanogel-treated MG-63 cells. RUNX2, ALP, BSP, and COL1A1 gene expression in MG-63 cells after treatment with CTL nanogel were significantly increased compared to the control group (p < 0.05). The hatching and viability rates of the embryos increased as the nanogel concentrations decreased, with the highest hatching rate observed at a 5 µL concentration. Developmental toxicity, such as spinal cord bent, tail bent, or yolk sac oedema, was not observed after treatment with CTL nanogel in zebrafish embryos.
Conclusions:
CTL nanogel can be used in treating bone defects and helping wound healing. The study also suggests that CTL nanogel had a concentration-dependent effect on zebrafish embryos’ viability and hatching rates only in very high concentrations.
Keywords
MG-63 cells, bone regeneration, nanogel, chitosan, lauric acid, thiocolchicoside, zebrafish embryosIntroduction
There is always a quest for developing newer materials to aid in bone tissue engineering to regenerate bone tissues lost due to trauma, infection, or malignancy. Recent research always focused on developing biomaterials with bone regeneration potential to be helpful in the field of medicine and dentistry. In dentistry, bone regeneration is always a focus of attraction in the treatment of alveolar bony defects, maxillary and mandibular ridge augmentation, healing of extraction sockets, implantology, in maxillofacial trauma cases, and orofacial surgery following cysts and tumors, osteomyelitis [1]. Regeneration of bone is a complex, intricate, but well-organized physiologic process involving numerous cellular mechanisms and pathways from normal fracture healing to continuous bone remodeling [2]. The bone regeneration process includes several osteoprogenitor stem cells of mesenchymal origin; the ones derived from endosteum and periosteum form osteoblasts and produce osteoid and alkaline phosphatase (ALP), which later undergo mineralization [1].
Nanotechnology is the science, engineering, and technology of manipulating matter at a scale, matter at a scale, ranging from 1 to 100 nm. Nanotechnology involves imaging, measuring, and modeling nanomaterials, leading to revolutionary technologies, sustainable products, and targeted drug delivery systems. Nanotechnology plays an increasingly important role in many areas of science and technology and has the potential to impact our lives significantly in the future [3, 4]. Nanogels are promising drug delivery systems due to their unique properties, such as high drug-loading capacity and stimuli-responsiveness [5]. Hydrogel-like materials can be modified to provide multipurpose drug delivery [6]. Nanogels have properties of both nanoparticles and hydrogels, making them an attractive approach for antimicrobial delivery systems [7]. Researchers have found that nanogels can improve drug delivery to inflamed tissues, such as those affected by arthritis [5]. Nanogels can be designed to be pH and temperature-responsive, which enables targeted drug delivery to specific sites in the body [8]. Dual stimuli-responsive nanogels have been found to enhance drug bioavailability and improve the effectiveness of existing therapies [8]. Overall, nanogels hold great promise as a novel drug delivery system for treating inflammatory conditions and other diseases [9].
Chitosan is a biopolymer that offers many advantages in biomedical applications. Its biocompatibility, biodegradability, and non-toxicity make it an excellent choice for medical applications such as wound healing and antimicrobial agents. Chitosan-based materials can be formed into various structures, making them ideal bioactive materials. Furthermore, chitosan has been used in nanotechnology-based formulations, including nanoparticles for drug, protein, and gene delivery through various administration routes. Chitosan’s antimicrobial properties also help develop antimicrobial agents. Additionally, it has been employed in tissue engineering and drug delivery. Chitosan is considered safe as a pharmaceutical excipient, making it a promising material for biomedical applications [10–15].
Lauric acid is a saturated fatty acid naturally found in plant oils, such as coconut and palm kernel [16]. Additionally, lauric acid has been studied for its antimicrobial, antiviral, antifungal, and anticancer properties [17]. Studies have also found that lauric acid can increase total serum lipoproteins, particularly high-density lipoprotein (HDL), and has a more favorable effect on total HDL than many other fatty acids [18]. Lauric acid is a significant component of coconut milk, oil, laurel, and palm kernel oil [19].
Thiocolchicoside is a drug that is commonly prescribed to treat muscle spasms and associated pain. It is a derivative of colchicoside, a natural compound found in autumn crocus plants and Gloriosa superba [20]. Thiocolchicoside is known for its efficacy and safety profile and is widely used to manage a range of conditions that cause muscle spasms. This includes lower back pain, cervical and lumbar spondylosis, fibromyalgia, postoperative pain, sports injuries, and chronic pain syndromes. The pharmacokinetics of thiocolchicoside make it an ideal medication for treating muscle spasms. It is rapidly absorbed after oral administration and has a high bioavailability, with peak plasma concentrations achieved within 1–2 h of administration. Thiocolchicoside is metabolized quickly in the liver and excreted primarily in the urine, with a half-life of approximately 4–6 h [19]. In clinical trials, thiocolchicoside has demonstrated significant efficacy in treating acute and chronic muscle spasms, with a rapid onset of action and sustained pain relief [21]. Thiocolchicoside’s safety profile, efficacy, and rapid onset action make it a highly effective muscle relaxant. Further research is needed to explore its full potential, mechanisms of action, and its use in combination with other medications.
In the present study, we tried to combine the anti-inflammatory properties of thiocolchicoside, the antimicrobial properties of lauric acid and the wound healing and regeneration potential of chitosan to obtain a novel gel which can be a breakthrough for the regeneration of intraoral bone defects specifically periodontal bone defects.
Over the last decade, zebrafish (Danio rerio) have become a valuable vertebrate model for assessing the developmental toxicity of drugs or chemicals [22, 23]. They serve as excellent models for investigating specific genes and signaling pathways. Hence, pharmaceutical companies and researchers use them for drug prescreening [24].
The main goal of this work is to propose a new chitosan-thiocolchicoside-lauric (CTL) nanogel to improve wound healing and bone tissue regeneration and evaluate its properties invitro, including reduction of metabolic activity.
Materials and methods
Preparation of CTL nanogel
Thiocolchicoside, lauric acid, and chitosan were obtained from Sisco Research Laboratories, India. CTL nanogel was prepared by adding 5 mL of prepared lauric acid solution to 5 mL of prepared thiocolchicoside solution. The reaction mixture was kept on a magnetic stirrer at 700 rpm for 2 h. After that, 10 mL of medium molecular-weight chitosan was added. The chitosan-mediated nanogel was stirred for up to 24 h to attain uniform dispersion [25].
Mechanical characterization of gel
pH
The pH of CTL formulation was determined by using a calibrated digital pH meter in triplicates. The pH meter was calibrated before each use with standard pH 4 and 7 phosphate buffer solutions and a molarity of 100 mM. The temperature of formulations was maintained at 25°C.
Viscosity
The viscosity of CTL nanogel was determined using Brookfield Digital Viscometer LVDV-II + PRO Brookfield at 25°C. The measurements were carried out using spindle no. 62 at the speed of 100 rpm in the sample and the viscosity was measured at 10 minutes after the rotation of the spindle.
Spread ability
This method uses a circular mold plate of glass (diameter = 20 cm, width = 0.2 cm) with a central orifice of 1.2 cm diameter, which is placed on a glass support plate (20 cm × 20 cm) positioned over millimetric graph paper. Each sample was introduced into the orifice of the die plate and the surface was levelled with a spatula. The plaque mold was carefully removed, and a glass plate of known weight was placed over the sample. After one minute, the diameter in opposing positions (as covered by the CTL nanogel) was read with the aid of the graph paper scale. Subsequently, we calculated the average diameter.
Syringe ability
All prepared CTL formulations were transferred into an identical 1 mL syringe placed with 20-gauge needle to a constant volume of 1 mL. The solutions that were easily passed from the syringe were termed as pass and those difficult to pass were termed as fail.
Gelling time
Gelling time of CTL formulation was measured by the test tube inversion method. In this method, 2 mL of the formulation was positioned in a 10 mL glass test tube. This test tube was placed in the water bath (37 ± 2°C) and gelling time was noted. Gelation temperature: 2 mL of formulations were transferred to test tubes sealed with paraffin and immersed in a water bath at 4°C. The temperature of the bath was augmented in increments of 1°C. The CTL nanogel formulation was examined for gelation, which was deemed to have occurred when the meniscus would no longer move upon tilting at 90°C. All measurements were performed in triplicate.
Chemicals
DMEM F-12, antibiotics, trypsin-EDTA, phosphate buffer saline (PBS), fetal bovine serum (FBS) from Gibco (Invitrogen, USA). 3-(4,5-dimethylthiazol-2-yl)-2,5-diphenyl tetrazolium bromide (MTT) reagent and dimethyl sulfoxide (DMSO) from Sigma Aldrich Chemicals Pvt Ltd, USA. All the reagents and chemicals used for this study were analytical grade.
Maintenance of the cell line
The human osteoblast-like cell line (MG-63) was provided by the National Centre Cell Sciences (NCCS), in Pune. The cells were cultivated in T25 culture flasks supplemented with Dulbecco’s modified Eagle medium (DMEM), 10% FBS, and 1% antibiotics, cells kept in a wet environment with 5% CO2 at 37°C. Once the cells had attained confluence, they were trypsinized and passed on.
The cell metabolic activity (MTT assay)
The human osteoblast-like cells (MG-63) were plated separately in 96 well plates with a concentration of 5 × 103 cells/well in DMEM media with 1× antibiotic solution and 10% FBS (Gibco) in a CO2 incubator at 37°C with 5% CO2. The cells were washed with 100 μL of 1× PBS; then, the cells were treated with CTL nanogel (5–100 μL/mL) collected from the earlier process and incubated in a CO2 incubator at 37°C with 5% CO2 for 24 h. After the treatment period, the medium was aspirated from cells. 0.5 mg/mL MTT prepared in plain media was added and incubated at 37°C for 4 h using a CO2 incubator. After incubation, the MTT medium was discarded from the cells and washed using 100 μL of PBS. The crystals formed were dissolved with 100 μL of DMSO and thoroughly mixed. The developed color intensity was measured at 570 nm. The absorbance was measured at 570 nm using a microplate reader. The percentage cell viability was measured using the formula:
Morphology study
Based on the MTT assay, we selected the optimal doses for further studies, including analyzing cell morphology changes with an Inverted Phase Contrast Microscope. 2 × 105 cells were seeded in 6-well plates and treated with CTL nanogel (10, 20, and 40 μL/mL) for MG-63 cells for 24 h. After 24 h, the medium was removed, and cells were washed once with PBS at pH 7.4. The plates were then observed under an Inverted Phase Contrast Microscope.
Scratch wound healing assay
MG-63 cells (2 × 105 cells/well) were seeded onto six-well culture plates. The cell monolayer was scratched using a 200 μL tip to create a wound, washed with PBS, and photographed in an inverted microscope. CTL nanogel (10 and 20 μL/mL) treatment was done for 24 h, and control cells received serum-free culture medium. After treatment, the wound area was photographed using the same microscope. The experiments were repeated in triplicate for each treatment group.
ALP and bone sialoprotein activity assay
Osteoblast-like cells (MG-63) were seeded in 6-well plates in triplicate at a density of 2 × 105 cells/well and cultured in defined media after reaching about 80% confluency. Following 24 h in culture, the cells were treated with CTL nanogel (10 and 20 μL/mL). The GENLISA™ ELISA kit (Krishgen Biosystems, USA) was used to detect ALP and bone sialoprotein (BSP) activity of CTL nanogel-treated osteoblast-like cells, following the manufacturer’s instructions.
Real-time polymerase chain reaction
The gene expression of bone biomarker genes was analyzed using real-time polymerase chain reaction (PCR). After the CTL nanogel (10 and 20 μL/mL) treatment, the total RNA was isolated by the standardized protocol with the help of Trizol reagent (Sigma). 2 μg of RNA is used for cDNA synthesis using reverse transcription using a PrimeScript, 1st strand cDNA synthesis kit (TakaRa, Japan). The specific primers (Table 1) were used to amplify the targeted genes. The polymerase chain reaction was performed with GoTaq® qPCR Master Mix (Promega), which contains SYBR green dye and all other components. Real-time PCR was carried out in a CFX96 PCR system (Biorad). The results were analyzed using the comparative CT method, and the 2−∆∆CT method was used to calculate fold change, as given by Schmittgen and Livak (2008) [26].
Real-time PCR primer sequence
Gene | Forward | Reverse | Tm (°C) |
---|---|---|---|
RUNX2 | GAGGGCACAAGTTCTATCTG | CGCTCCGGCCCACAAATCTC | 60 |
ALP | GCTGTAAGGACATCGCCTACCA | CCTGGCTTTCTCGTCACTCTCA | 60 |
BSP | GGCAGTAGTGACTCATCCGAAG | GAAAGTGTGGTATTCTCAGCCTC | 60 |
COL1A1 | GACATGTTCAGCTTTGTGGACCT | GCCAGCAGATCGGTTCCAG | 60 |
RANKL | GCCTTTCAAGGAGCTGTGCAAAA | GAGCAAAAGGCTGAGCTTCAAGC | 60 |
OPG | GGTCTCCTGCTAACTCAGAAAGG | CAGCAAACCTGAAGAATGCCTCC | 60 |
PCR: polymerase chain reaction; ALP: alkaline phosphatase; BSP: bone sialoprotein; RUNX2: Runt-related transcription factor 2; Tm: melting temperature; RANKL: receptor activator of nuclear factor kappa beta ligand; OPG: osteoprotegerin
Zebrafish embryonic toxicology evaluation
In this study, the embryonic toxicology evaluation of CTL nanogel was conducted using wild-type zebrafish (Danio rerio) received from local Indian vendors. The zebrafish were maintained in tanks with controlled environmental conditions, including a temperature of 28 ± 2°C, a light/dark cycle of 14:10 h, and a pH range of 6.8–8.5. They were fed twice daily with commercially available dry blood worms or optimal food. One female and three males were placed in a breeding tank to get zebrafish embryos. The eggs formed were collected and carefully rinsed using freshly prepared E3 medium without methylene blue. The embryos were then exposed to different nanogel concentrations ranging from 5 to 80 µL for 24 to 96 h post-fertilisation in culture plates of varying well sizes (6, 12, and 24 wells) with 20 embryos per 2 mL solution per well. The treatment and control groups were replicated three times. Embryonic mortality and hatching rates were evaluated as study endpoints every 24 h, and any malformations observed among the embryos and larvae in both groups were identified and accurately documented using a COSLAB-Model: HL-10A light microscope. Dead embryos were removed from the nanogel-treated groups every 12 h and maintained at a temperature of 28°C. All experimental plates were covered with foil to prevent light interference.
Statistical analysis
One-way analysis of variance (ANOVA) and the student’s t-test was used to analyse all of the data using SPSS Statistics, version 26 (IBM Corp., Armonk, NY). The results are shown as mean ± SD in triplicate. The threshold for statistical significance was set at p < 0.05.
Results
The CTL nanogel was found to have an average pH of 6.1, with a mean viscosity of 4,827 Cps and spread ability of 16.04 g∙cm/s, shown in Table 2. The average gelling temperature and gelling time of the nanogel were found to be 24.4°C and 143 s respectively, and all the batches also passed the syringe ability test as shown in Table 2.
Mechanical properties of CTL nanogel
CTL nanogel | Gelling temperature (°C) | Syringe ability | Viscosity (Cps) | pH | Spread ability (g∙cm/s) | Gelling time (s) |
---|---|---|---|---|---|---|
Batch 1 | 23.9 | Pass | 4,831 | 6.12 | 11.58 | 123 |
Batch 2 | 24.1 | Pass | 4,830 | 6.15 | 18.30 | 159 |
Batch 3 | 25.2 | Pass | 4,820 | 6.08 | 18.25 | 147 |
CTL: chitosan-thiocolchicoside-lauric
CTL nanogel was found to have no significant effect on cell metabolic activity in MG-63 cells when treated with 5–20 μL/mL, after which a dose-dependent decrease in viability in MTT assay after 48 h (p < 0.05) (Figure 1). No prominent morphological changes or nuclear abnormalities were found in the MG-63 cells when treated with various concentrations of CTL nanogel (10, 20, and 40 μL/mL) for 24 h compared to the control group (Figure 2). When treated with 10 and 20 μL/mL after 24 h, the invitro scratch wound healing assay showed enhanced migration of cells and, thereby indicated the improved wound healing ability of CTL nanogel (Figure 3A and 3B). There was a 50% reduction in the wound when treated with 20 μL/mL of CTL nanogel and 38% wound closure by 10 μL/mL in contrast to only 24% wound closure by control. ELISA showed significant ALP and BSP production by CTL nanogel-treated MG-63 cells. When treated with 20 μL/mL, CTL nanogel ALP concentration was 35 ng/mL, while 15 ng/mL was found for control. BSP concentration was found to be 28 ng/mL in the 20 μL/mL CTL nanogel treated group compared to 14 ng/mL untreated control group (Figure 4A and 4B). Runt-related transcription factor 2 (RUNX2), ALP, BSP, and COL1A1 gene expression in MG-63 cells after treatment with 20 μL/mL CTL nanogel showed a 3-fold change, a 5-fold, 4-fold, and 4-fold increase in genetic expression, respectively, when compared to the control group (p < 0.05) (Figure 5).
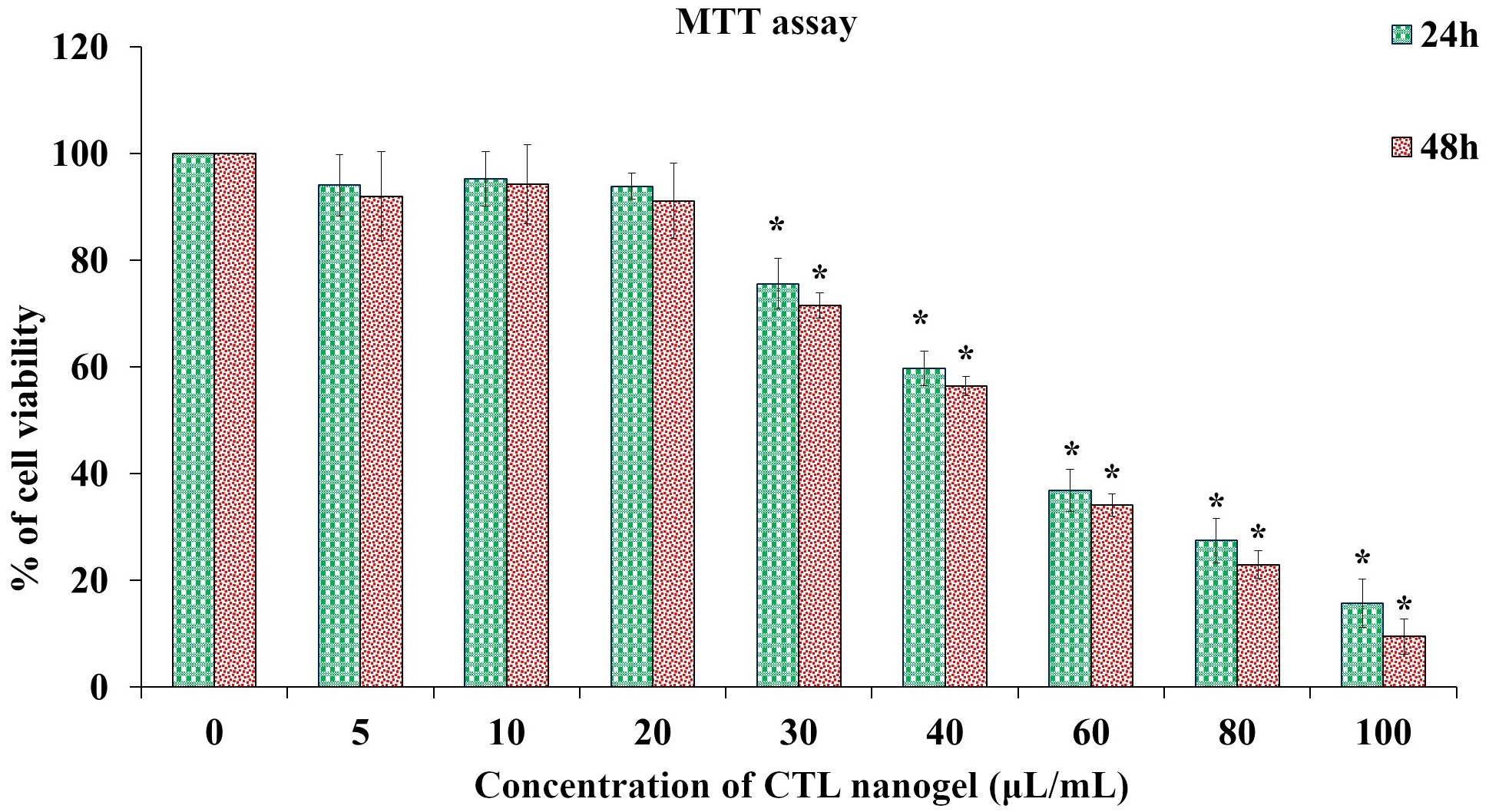
Cytocompatibility of CTL nanogel on MG-63 cells. Cells were treated with (5–100 μL/mL) for 24 h, and 48 h, and cell viability was evaluated by MTT assay. Data are shown as means ± SD (n = 3). *Compared with the control blank group, p < 0.05. SD: standard deviation; CTL: chitosan-thiocolchicoside-lauric
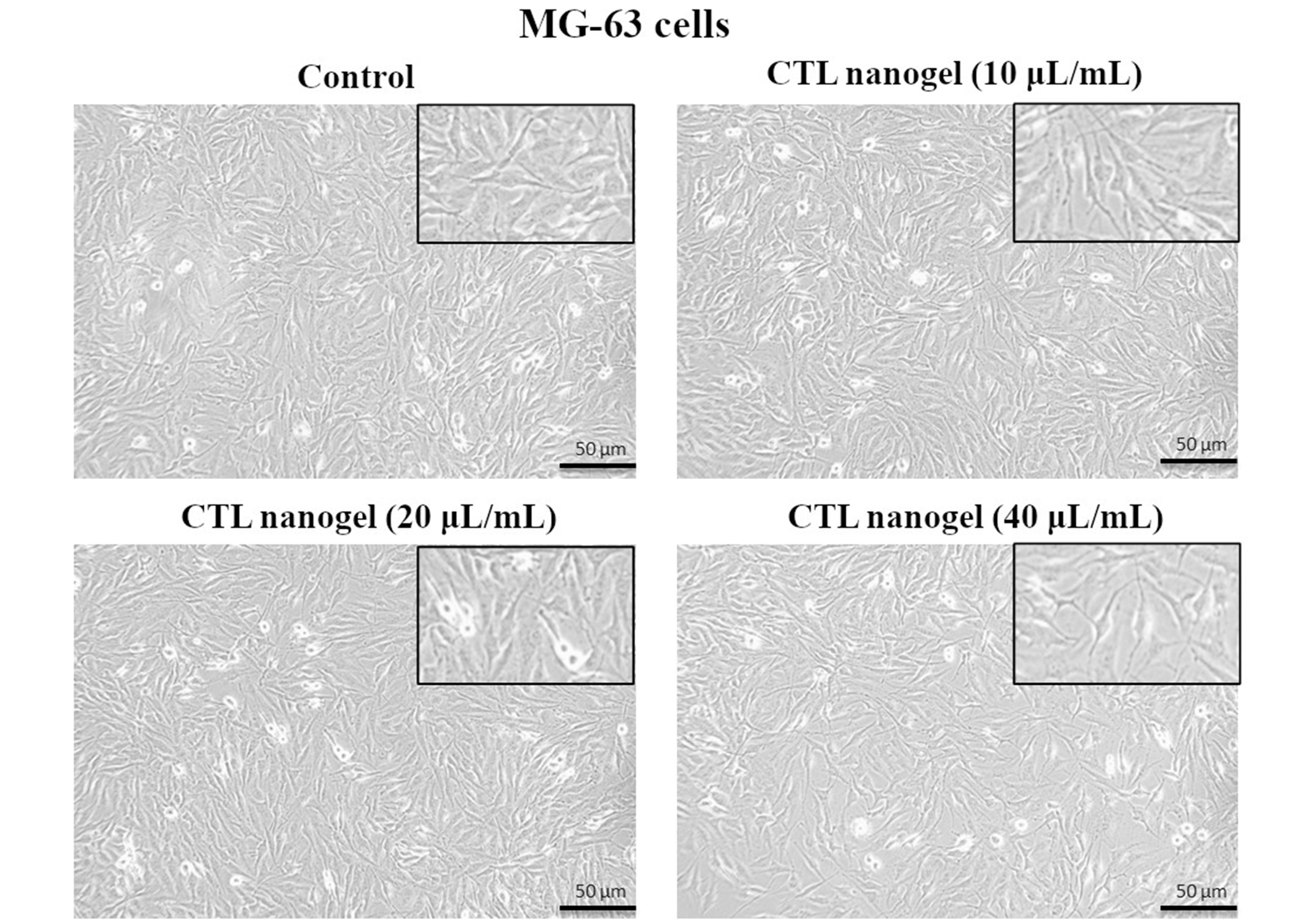
Effect of CTL nanogel on cell morphology of MG-63 cells (20× magnification). Cells were treated with CTL nanogel (10, 20, and 40 μL/mL) for 24 h along with the control group. Images were obtained using an Inverted Phase Contrast Microscope. CTL: chitosan-thiocolchicoside-lauric
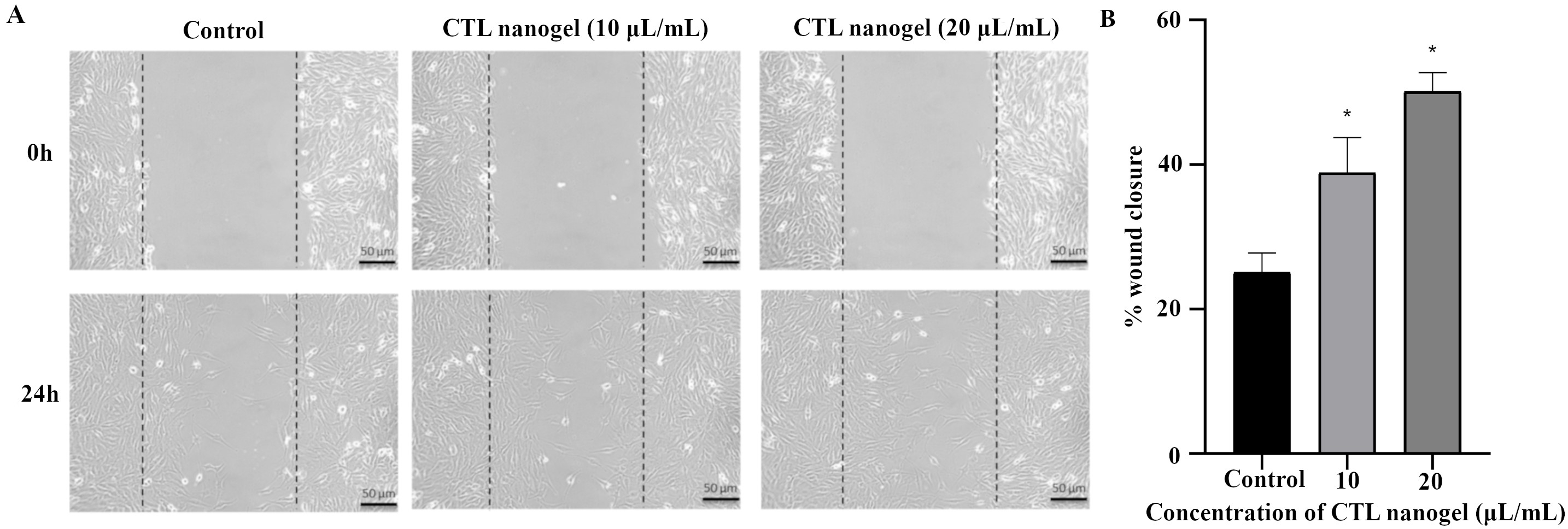
Invitro scratch wound healing assay. (A) Inverted Phase Contrast image of invitro scratch wound healing assay. Human MG-63 cells were injured and cell migration assay with and without treatment of CTL nanogel (10 and 20 μL/mL) was performed for 24 h. (B) Percentage of wound closure by MG-63 cells when treated with CTL nanogel (10 and 20 μL/mL) after 24 h. *Compared with the control blank group, p < 0.05. CTL: chitosan-thiocolchicoside-lauric
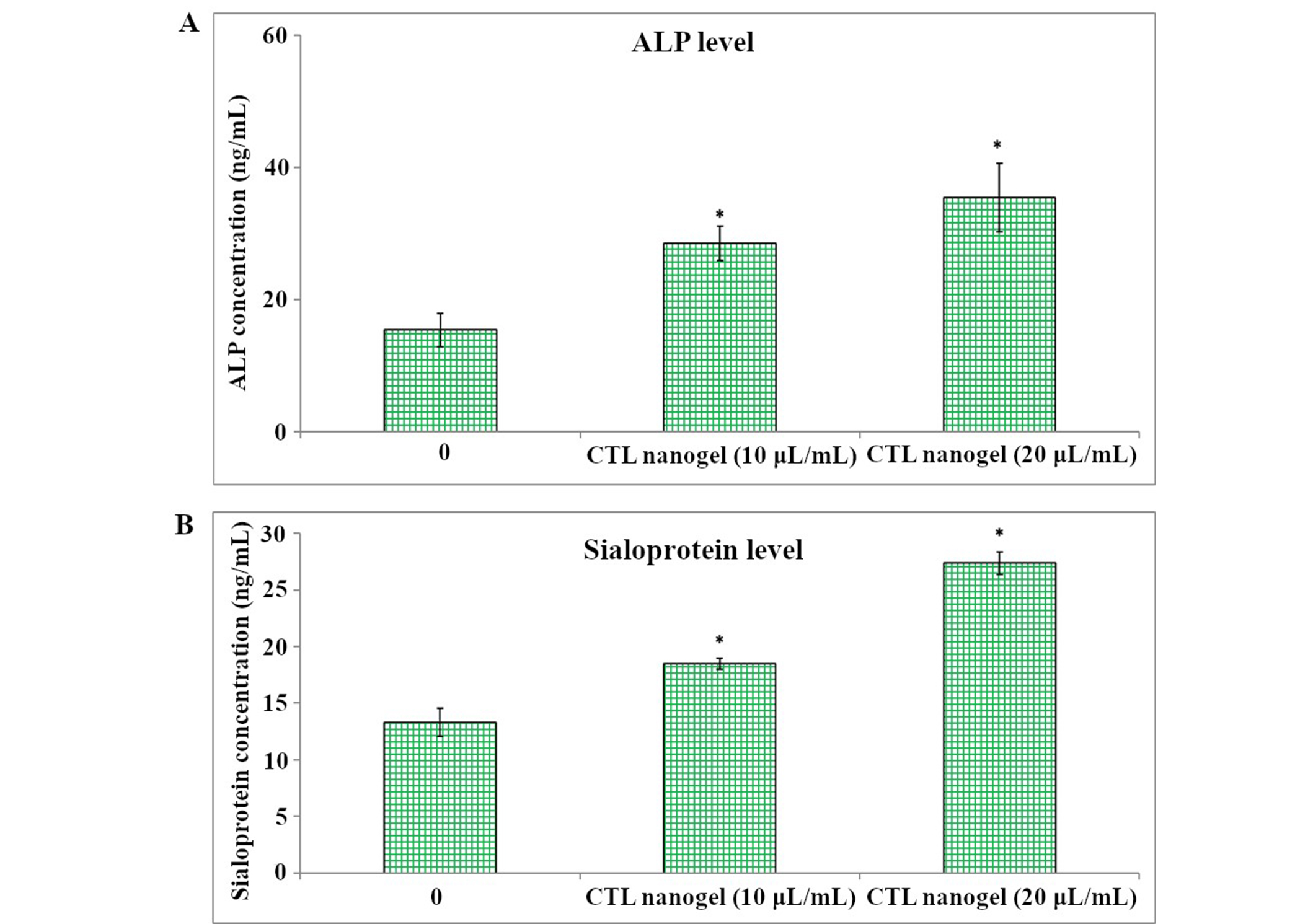
Alkaline phosphatase (ALP) (A) and bone sialoprotein levels (B) in MG-63 cells were assessed by ELISA. The condition media was used from control and CTL nanogel 10 and 20 μL/mL treated cells. Each bar represents the mean ± SD. *Statistically significant difference between control and treated (p < 0.05). SD: standard deviation; CTL: chitosan-thiocolchicoside-lauric
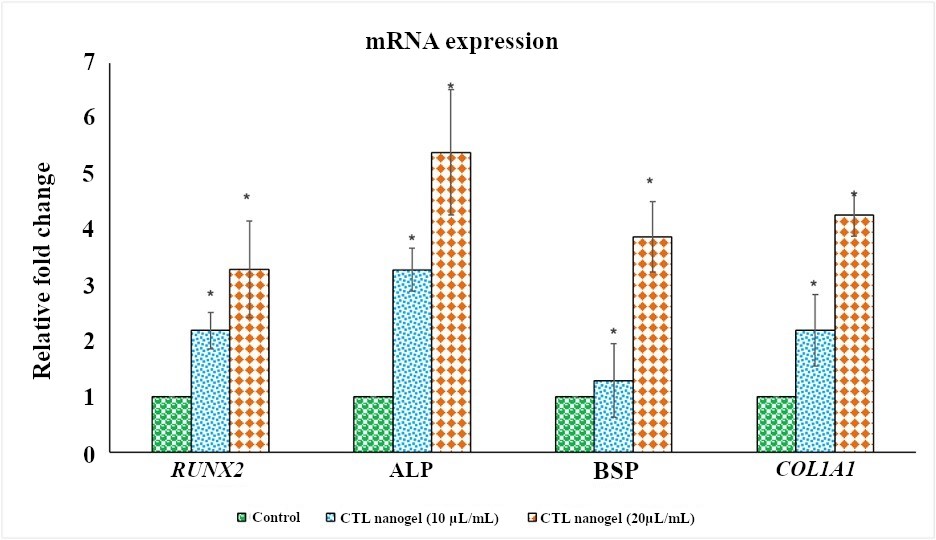
Effect of CTL nanogel (10 and 20 μL/mL) on RUNX2, ALP, BSP, and COL1A1 genes expression in MG-63 cells. Target gene expression is normalized to GAPDH mRNA expression and the results are expressed as fold change from control. Each bar represents the mean ± SEM of three independent observations. *Represents statistical significance between control versus drug treatment groups at p < 0.05 level. CTL: chitosan-thiocolchicoside-lauric; SEM: standard error of the mean; ALP: alkaline phosphatase; BSP: bone sialoprotein
The hatching rates were found to increase with decreasing concentration of the CTL nanogel. At the highest concentration tested (80 µL), the hatching rate was 60%. As the concentration decreased to 40 µL, 20 µL, 10 µL, and 5 µL, the hatching rates increased to 73%, 88%, 92%, and 96%, respectively after 72 h (Figure 6A). Compared to the control group, which had a hatching rate of 100%, the nanogel showed a concentration-dependent reduction in the hatching rate only after 40 µL. The highest concentration tested (80 µL) showed the most significant reduction in hatching rate (60% reduction compared to the control), while the lowest concentration tested (5 µL) showed the slightest reduction in hatching rate (4% reduction compared to the control). The lowest viability rate was observed at 80 µL concentration, with a rate of 61%, and the viability rate increased with decreasing nanogel concentration. The highest viability rate among the treated groups was observed at 5 µL concentration, with a rate of 96%. These results suggest that CTL nanogel may have a concentration-dependent effect on the viability of zebrafish embryos after the concentration of 40 µL after 72 h (Figure 6B). No developmental toxicity was observed, such as spinal cord bent, tail bent, or yolk sac oedema, as shown in Figure 7 after 3 days of treatment with CTL nanogel. This proves the less toxic nature of the synthesized CTL nanogel.
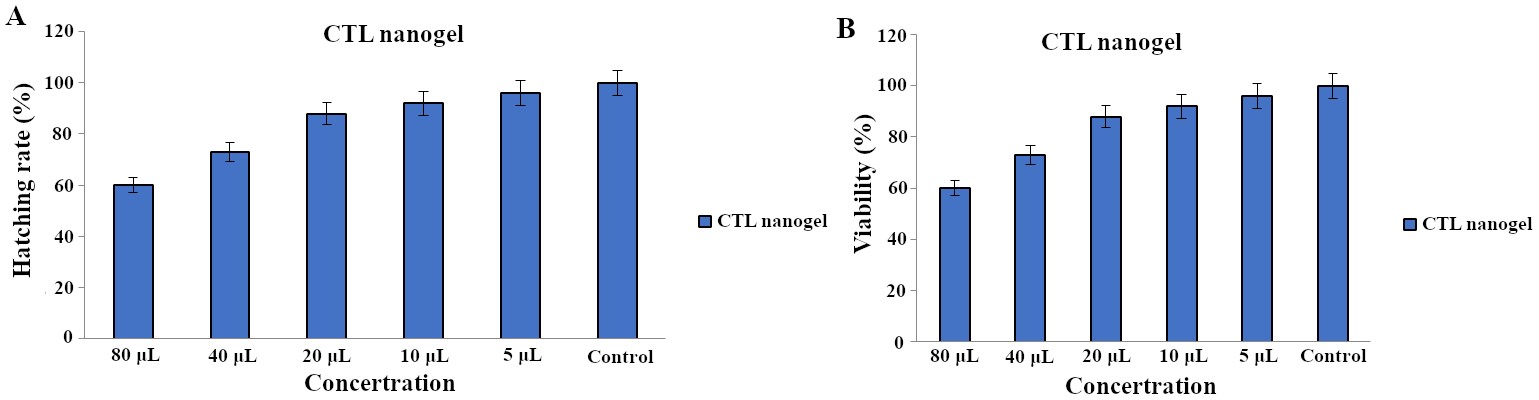
Hatching (A) and viability (B) rate of zebrafish embryos treated with CTL nanogel after 72 h. CTL: chitosan-thiocolchicoside-lauric

Morphological characteristics of zebrafish embryos treated with CTL nanogel (80 µL/mL) on days 1 (A), 2 (B), and 3 (C). CTL: chitosan-thiocolchicoside-lauric
Discussion
The mechanical properties of the biomaterials, like gels which cause early drug release and faster disintegration always affect the bone regeneration potential of the drug as it may end up in poor bioavailability. There is always a need for developing newer drug delivery systems that are biodegradable and non-toxic with enhanced drug loading capacity and controlled release as well as sustained action at the site of delivery [27]. Hence, the chitosan formulations were studied for pH, spread ability, syringe ability, viscosity, gelling temperature and time. The promising drug loading and release study results of CTL nanogel were reported in our previous research [28].
In the previous study, we reported the cytocompatibility of CTL nanogel on human gingival fibroblasts from concentrations 10–80 µL/mL and found increased viability at 40 µL/mL [25]. In the present study, CTL nanogel did not significantly affect the viability of MG-63 cells from 5 to 20 µL/mL. Still, at 30 µL/mL and 40 µL/mL, cells’ viability decreased only by 74% and 60%, respectively, after 24 h. At 80 µL/mL, viability was 30% and at 100 µL/mL, it further decreased to 16%. The MTT results from concentrations 5 µL/mL to 100 µL/mL after 24 and 48 h were plotted. Invitro scratch assay, also known as wound healing invitro assay records the migration of cells after trauma in the single layer of cells and is always considered as a cost-effective and well-developed invitro method. The basic steps involve the creation of a “scratch” in a cell monolayer [29]. Invitro scratch wound healing assay showed faster wound healing and cell migration in CTL nanogel-treated MG-63 cells compared to the control group, similar to the study reported on CTL-treated human gingival fibroblasts [25]. Osteoblasts secrete several proteins like ALP, collagen type I, and BSP to block their differentiation into the chondrocyte and adipocytes [30]. ALP, a membrane-bound enzyme in osteoblast cells, marks the early differentiation and plays a crucial role in the calcification. Collagen type I is an essential substance in the extracellular matrix (ECM) of bone, which mediates cell adhesion, as well as proliferation and differentiation of the osteoblast [31].
BSP, a non-collagenous protein, helps matrix mineralisation by incorporating calcium and forming nodules [32]. ALP and BSP showed statistically significant expression in MG-63 cells after CTL nanogel treatment in the present study (p < 0.05). Runt-related transcription factor 2 (RUNX2) is the critical transcription factor initiating osteodifferentiation of the mesenchymal cell, which was found to have significant expression after treatment with 10 and 20 μL/mL of CTL nanogel in MG-63 cells when compared to the control group in the present study. After treatment with CTL nanogel, there was also significant expression of ALP, BSP, and COL1A1 genes in MG-63 cells, showcasing the bone regeneration potential of CTL nanogel.
It is crucial to remember that MG-63 cells, as a cell line originating from osteosarcoma, would not be able to perfectly mimic the actions of mesenchymal stem cells or actual osteoblasts. Their fast pace of proliferation and changed genetic background may affect how the nanogel is interpreted by cells. Therefore, the results would be improved by further validation utilizing primary osteoblasts or invivo models. In the previous research, we reported the anticancer activity of CTL nanogel by inhibition of KB-1 oral cancer cell proliferation by inducing cell cycle arrest in cancer cells [28].
The use of zebrafish embryos as a model for developmental toxicity testing has been extensively researched. The embryonic zebrafish model provides the advantages of whole-animal investigations with the convenience of cell culture, making it a valuable toxicity testing platform. The zebrafish embryo model offers a valuable alternative to mammalian testing for developmental toxicity testing, acting as a bridge between cell and protein-based assays and mammalian testing [33]. As long-term toxicity assessment using early stages of human and rodent lives is limited, zebrafish embryos are regarded as an excellent model for biosafety assessments of drugs as it boasts an invivo model of toxicity analysis with all the advantages of an invitro assay [24].
Developmental exposure to perfluorooctanesulfonic (PFOS) acid caused an increase in the concentrations of lauric acids in zebrafish embryos. The study also suggested that PFOS exposure during the embryonic period can impact embryonic nutrition, pancreatic morphology, and adiposity in zebrafish embryos. However, no information is available regarding the specific effects of lauric acid on zebrafish embryos. These findings suggest that the embryonic period is a vulnerable window of metabolic programming in zebrafish [34]. Several studies have investigated using various nanogels in zebrafish embryos and larvae. Zebrafish embryos and larvae have been proposed as model organisms for developmental behavioural studies [35]. These studies demonstrate the potential of using zebrafish embryos and larvae as model organisms for toxicological and pharmacological research, particularly in developing and testing new materials and drugs. Zebrafish embryos and larvae have short life cycles, are cost-effective, easily obtained, and have a well-characterized developmental process that can be used to monitor the effects of these materials on embryonic development and behaviour. This model’s optical clarity and reproducible characteristics add to its advantages [36].
Moreover, using zebrafish as a model organism provides an ethical alternative to animal testing, as it reduces the need for vertebrate animals and allows for the analysis of large sample sizes. Overall, these studies have contributed to the growing body of literature on using zebrafish embryos and larvae as a model organism for biomedical research, highlighting the potential of this model in evaluating the safety and efficacy of new materials and drugs. Zebrafishes are genetically similar to humans, with sequenced genomes with 25 chromosomes. The brain, heart, kidneys, muscles, liver, and other tissues are anatomically, morphologically, physiologically, and molecularly similar to humans and hence widely used for biomedical research [37]. However, it should be noted that zebrafish embryos differ significantly from mammalian systems in terms of skeletal development and remodeling, even with these benefits. Specifically, zebrafish have a lower prevalence of endochondral ossification and a different control of osteoclast activity than mammals [38]. These variations imply that although zebrafish offer insightful information about developmental toxicity, additional research employing mammalian models is required to completely confirm the CTL nanogel’s capacity for bone regeneration.
It was reported that the other chitosan formulations like sodium glycerophosphate or gelatin hydrogels using erythropoietin, aspirin, ibuprofen, meloxicam, and antimicrobials like clindamycin, doxycycline, ornidazole was found to be effective in the management of periodontitis and helps in bone regeneration [27]. CTL nanogel offers a unique approach to drug delivery, with precise control and targeting capabilities that can enhance therapeutic efficacy while reducing unwanted side effects [39–41]. But, invivo research in mammalian models is always necessary to validate the wound healing activity as well as to explore the bone regeneration efficacy of the nanogel, which is considered as the limitation of the present study. Gene expression analysis of an extended panel can give an extensive view of nanogel effects. Therefore, we recommend animal studies in upcoming future to analyse the bone regeneration potency of CTL nanogel.
Conclusions
In conclusion, this study evaluated the invitro bone regenerative and wound healing potential of CTL nanogel using MG-63 osteoblasts-like cells. The nanogel also did not cause developmental toxicity in zebrafish embryos and is cytocompatible to MG-63 cells. The results also highlight the importance of using zebrafish embryos as a model organism to evaluate the toxicity of newer materials, as they can provide rapid, cost-effective, and reliable data on the potential toxicity of these materials. Further in vivo research in the mammalian model is warranted for exploring the wound healing and bone regenerative potential of CTL nanogel, which could be a boon in treating bone defects and helping in wound healing.
Abbreviations
ALP: | alkaline phosphatase |
BSP: | bone sialoprotein |
CTL: | chitosan-thiocolchicoside-lauric |
HDL: | high-density lipoprotein |
PCR: | polymerase chain reaction |
PFOS: | perfluorooctanesulfonic |
Declarations
Acknowledgments
The authors would like to acknowledge Prof. Luis Eduardo Almeida, native English speaker and associate professor at the Department of Surgical Sciences, School of Dentistry, Marquette University, Milwaukee, Wisconsin, USA for reviewing and correcting the scientific English of the article.
Author contributions
AM: Conceptualization, Methodology, Formal analysis, Investigation, Data curation. MAI: Methodology, Investigation. KR: Conceptualization, Data curation. EP and RS: Software. MMM: Writing—review & editing. MC: Writing—review & editing, Project administration. GM: Writing—original draft, Writing—review & editing, Supervision. All authors read and approved the final manuscript.
Conflicts of interest
Giuseppe Minervini who is the Guest Editor of Exploration of Medicine had no involvement in the journal review process of this manuscript. The other authors declare no conflict of interest.
Ethical approval
The research protocol was approved by the Institutional Human Ethical Committee of Saveetha Dental College and Hospitals, Saveetha Institute of Medical and Technical Sciences, Chennai (IHEC Ref No: IHEC/SDC/PhD/OPATH-1954/TH-001) and (SRB Ref No: SRB/SDC/PhD/OPATH-1618/TH-006).
Consent to participate
Not applicable.
Consent to publication
Not applicable.
Availability of data and materials
The datasets used and/or analyzed during the current study are available from the corresponding author (Giuseppe Minervini, giuseppe.minervini@unicampania.it) upon reasonable request.
Funding
Not applicable.
Copyright
© The Author(s) 2025.
Publisher’s note
Open Exploration maintains a neutral stance on jurisdictional claims in published institutional affiliations and maps. All opinions expressed in this article are the personal views of the author(s) and do not represent the stance of the editorial team or the publisher.