Abstract
Since obesity is one of the main factors in the development of insulin resistance (IR) and is also associated with increased oxidative stress (OxS) rate, this study aims to review the published literature to collate and provide a comprehensive summary of the studies related to the status of the OxS in the pathogenesis of obesity and related IR. OxS represents an imbalance between the production of reactive oxygen and nitrogen species (RONS) and the capacity of the antioxidant defense system (AOS) to neutralize RONS. A steady-state of RONS level is maintained through endogenous enzymatic and non-enzymatic AOS components. Three crucial enzymes, which suppress the formation of free radicals, are superoxide dismutases, catalases, and glutathione peroxidases. The second line of AOS includes non-enzymatic components such as vitamins C and E, coenzyme Q, and glutathione which neutralizes free radicals by donating electrons to RONS. Emerging evidence suggests that high RONS levels contribute to the progression of OxS in obesity by activating inflammatory pathways and thus leading to the development of pathological states, including IR. In addition, decreased level of AOS components in obesity increases the susceptibility to oxidative tissue damage and further progression of its comorbidities. Increased OxS in accumulated adipose tissue should be an imperative target for developing new therapies in obesity-related IR.
Keywords
Antioxidant defense system, inflammation, insulin resistance, obesity, oxidative stress, reactive oxygen and nitrogen speciesIntroduction
Oxidative stress (OxS) derives from an imbalance between the production of reactive oxygen and nitrogen species (RONS) and the capacity of the antioxidant defense system (AOS) to neutralize RONS [1]. Primarily, RONS are crucial physiological modulators of the redox state signaling molecules and pathways. In OxS, RONS are directly or indirectly involved in oxidative degradation of nucleic acids, proteins, and lipids, leading to cell and tissue damages. The balanced level of RONS is maintained through a highly conserved biochemical mechanism—AOS, which comprises endogenous enzymatic and non-enzymatic antioxidant components. The most important, first line of AOS, is comprised by the action of three vital enzymes: superoxide dismutases (SOD), catalases (CAT), and glutathione peroxidases (GPx) which prevent or suppress the formation of reactive species in cells. Non-enzymatic components of AOS include, among others, vitamins C and E, coenzyme Q, and glutathione (GSH), representing the second line of AOS, which neutralizes free radicals by donating electrons to RONS [2, 3]. Accumulating evidence suggests that RONS directly activate cellular pathways involved in the generation of OxS, leading to the progression of many different diseases, including carcinogenesis [4], neurodegeneration [5], acute brain ischemia [6], atherosclerosis [7, 8], and aging [9]. Furthermore, RONS-generating systems are involved in various pathophysiological processes such as inflammation, hypertension, and vascular remodeling, contributing to type 2 diabetes mellitus (T2DM), obesity, and hypercholesterolemia [10–15].
Obesity is a foremost underlying risk factor of several chronic diseases, including metabolic syndrome (MS), T2DM, cardiovascular diseases, fatty liver diseases, and cancer [16–24], all of which share a common pathological condition, insulin resistance (IR) [25]. Fat accumulation in the visceral tissues and organs in obesity leads to free fatty acids (FFAs) release into the portal circulation and further impairment of glucose metabolism. High glucose and lipids levels in circulation increase the energy substrates delivered to cellular metabolic pathways, increasing RONS production [26]. The association of elevated RONS production and generation of OxS in obesity is related to activation of the innate immune system in adipose tissue and subsequent low-grade chronic systemic inflammation [27]. Excessive adipose tissue is a source of pro-inflammatory cytokines that increase RONS production and lipid peroxidation rate [28], leading to OxS [27].
Numerous human and animal studies reported that the amount and activity of AOS components are decreased in obesity, increasing susceptibility to oxidative tissue damage [29–34]. Furthermore, an increase in RONS levels in adipose tissue of obese patients contributes to the generation of OxS and further progression to IR [25]. Additionally, factors that also contribute to OxS in obesity include hyperleptinemia [35], chronic inflammation [36], and postprandial ROS generation [37]. This review aims to explore, discuss, and summarize the latest literature data and current knowledge regarding the OxS in the pathogenesis of obesity and related IR.
AOS
A steady-state of RONS level is maintained through a complex AOS that includes endogenous enzymatic and non-enzymatic antioxidants. Non-enzymatic components of AOS are vitamins A, C, and E, polyphenols, alpha-lipoic acid, thioredoxin, GSH, melatonin, coenzyme Q, and β-carotenoids. Additionally, some proteins, such as ferritin, transferrin, lactoferrin, caeruloplasmin, act as antioxidants, binding and sequestering transition metals that may start oxidative reactions. The antioxidant enzymes include SOD, CAT, GPx, glutathione reductases (GR), glutathione-S-transferases, thioredoxin reductase, peroxiredoxins, and reduced nicotinamide adenine dinucleotide phosphate (NADPH):ubiquinone oxidoreductase [2, 3]. The components of AOS act at several different levels that may be radical preventive, radical scavenging, and radical-induced damage repair. The first line of defense antioxidants (SOD, CAT, and GPx) acts to prevent or suppress the formation of RONS in cells. The second line of defense antioxidants neutralizes free radicals by donating electrons, becoming free radicals of lesser damaging effects (vitamins C and E, coenzyme Q, and GSH) [2, 3]. There is also a third and fourth line of defense antioxidants based on defense lines. The DNA repair enzyme systems and proteolytic enzymes constitute the third line of defense antioxidants, which repair the damage caused by free radicals to biomolecules, and remove oxidized or damaged DNA [38], proteins, and lipids to prevent their toxic accumulation in the cell [39]. Fourth line defense antioxidants such as adaptation fundamentally implicate an adaptation mechanism in which the signal for the production and actions of free radicals induces formation and transport of the appropriate antioxidant to the right site [39].
Obesity and IR
A state of obesity is characterized by an increase in body mass and excessive fat accumulation in the visceral tissues and organs. Under continuous nutrient and energy exposure, the organism loses the ability to adapt and maintain homeostasis, and the consequent emergence of metabolic stress leads to inflammation and organelle dysfunction [40, 41]. As one of the leading endocrine organs in the body, adipose tissue is responsible for the production and secretion of multiple cytokines, chemokines, hormones, and other inflammatory mediators, collectively called adipokines [42]. Adipocytes represent the major cell type of adipose tissue and the primary source of adipokines, including adiponectin and leptin [43]. However, macrophages and T cells accumulate in adipose tissue of obese animals, leading to the increased expression of inflammatory marker molecules, including tumor necrosis factor-alpha (TNF-α) and inducible nitric oxide synthase [44]. Secreted adipokines act in a paracrine fashion, amplifying inflammation within the adipose tissue [45]. At the same time, adipokines pass into the circulation, promoting important systemic effects, which precedes a significant increase in circulating insulin levels [46]. The pancreatic β-cells synthesize and release supraphysiological insulin levels, keeping glucose metabolism equilibrated. Over time, IR arises, a pathological condition that implies the inability of peripheral tissues to sufficiently respond to insulin stimulation due to nutrient accumulation, OxS, and chronic inflammation. As the pancreas fails in overcoming IR with more insulin release, hyperglycemia and, eventually, diabetes develop [47].
OxS in obesity and IR
The production of RONS and generation of OxS associated with obesity are strongly related to the activation of the innate immune system in adipose tissue and subsequent low-grade chronic systemic inflammation [27]. Furthermore, during OxS, RONS are responsible for widespread lipid peroxidation in cells. Cell and tissue destruction mediated by radicals often enhance lipid peroxidation since the level of antioxidants is decreased, and transition metal ions, which stimulate the peroxidation process, are released from disrupted cells [27].
The presence of excessive adipose tissue has been identified as a source of pro-inflammatory cytokines TNF-α, interleukin-1 (IL-1), and IL-6, which generate an increase in RONS production and lipid peroxidation rate [28]. On the other hand, RONS induce the further release of pro-inflammatory cytokines and expression of adhesion molecules and growth factors [48] via activation of redox-sensitive transcription factors, such as nuclear factor kappa B (NFκB) and activator protein-1, and also the NADPH oxidase pathway (Figure 1) [49, 50].
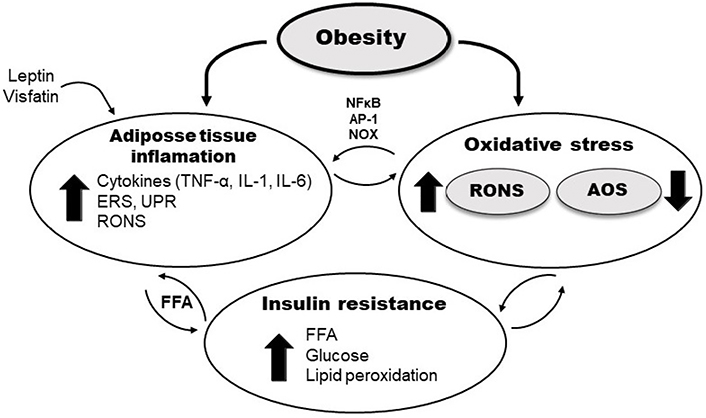
Schematic presentation of different pathways involved in OxS in obesity and IR. AP-1: activator protein-1, ERS: endoplasmic reticulum stress; NOX: NADPH oxidase; UPR: unfolded protein response
Additionally, OxS is associated with endoplasmic reticulum stress, during which misfolded proteins activate the UPR, responsible for protein folding and degradation of aberrantly packaged proteins. Prolonged UPR and oxidative protein folding machinery cause elevated RONS production followed by the systemic release of FFA and pro-inflammatory mediators [51]. Furthermore, some of the adipokines also induce the production of RONS, generating OxS and irregular production of other different adipokines [36]. The hormone leptin, secreted in adipocytes, exhibits pro-oxidative and pro-inflammatory effects, increasing macrophages’ phagocytic activity, inducing the synthesis of pro-inflammatory cytokines, and increasing levels of endothelial cell dysfunction and activation markers [52]. In addition, visfatin is an adipokine that also shows pro-oxidant and pro-inflammatory effects, mediated by NFκB signaling pathway (Figure 1) [53].
An additional, important mechanism involved in generating OxS in obesity results from excessive accumulation of fat in the adipocytes, and this leads to a pathological increase of FFA in the serum, impairment of glucose metabolism, and accumulation of fat and glucose in the heart, liver, muscles, and pancreas [26]. As a result, mitochondrial and peroxisomal oxidation and the production of RONS increase, leading to OxS, mitochondrial DNA injury, depletion of ATP, and lipotoxicity [54, 55]. Moreover, a chronic increase in intracellular RONS levels in adipocytes due to mitochondrial dysfunction interferes with insulin signaling pathways and leads to the development of IR [56]. Also, high circulating glucose in obesity-induced IR could further enhance lipid peroxidation. Hyperglycemia may directly affect oxidative lipid and protein modification via the formation of glucose-derived free radicals in the protein glycation process [57]. Furthermore, β-cells of the pancreas exposed to hyperglycemia may produce RONS, suppressing insulin secretion induced by glucose [58]. Also, RONS themselves decrease the activity of the antioxidant enzymes, including CAT and GPx activity [59], and copper (Cu)/zinc (Zn)-SOD is reported to be inactivated by the glycation of specific lysine residue during hyperglycemia [60].
AOS in obesity and IR
β-cells of the pancreas have relatively low expression of many antioxidant enzymes, which makes β-cells susceptible to RONS-induced damage [61]. Glucotoxicity and lipotoxicity induce pancreatic β-cell dysfunction and liver IR, which are critical factors causing T2DM [62]. Accumulation of fat in the liver reduces the efficiency of antioxidant mechanisms in this organ, favoring OxS-related obesity [63]. Activities of SOD, CAT, and GPx are inversely related to body mass index (BMI) in obese children and adults [25, 64, 65]. AOS activity is also reduced in patients with MS [66, 67]. Furthermore, antioxidant defenses in obesity can also be impaired by deficiencies in minerals and non-enzymatic antioxidants [68].
Recently published data suggest that obesity is strictly linked to changes in redox state. Significant association of antioxidant defense parameters with anthropometric, lipid, and inflammatory markers has also been shown in obese young adults with increased risk of cardiovascular diseases [69, 70]. In addition, the latest advances in the field of obesity-related OxS biology are explorations regarding telomere length [71] and mitochondrial OxS [72, 73]. It shows that telomeres shorten according to the length of obesity phenotype and also to the degree of OxS influenced by obesity [71]. Furthermore, recent studies show the relevance of mitochondrial OxS in metabolic alterations associated with obesity and mitochondrial OxS in the dysbiosis associated with a high-fat diet (HFD) in rats [72, 73].
Evidence from animal studies
At the onset of obesity, antioxidant enzymes expression and activity increase in tissues to counteract the damaging effects of OxS which was reported in studies on animal models (Table 1). In vitro studies showed that extracellular (EC)-SOD expression is up-regulated in differentiated 3T3-L1 adipocytes of mice co-cultured with stimulated macrophages [74]. Thus, SOD may be stimulated to protect adipocytes from OxS generated by infiltrated macrophages [75]. In the heart of HFD-fed male mice, production of hydrogen peroxide (H2O2) increases and rapidly up-regulates CAT, probably to protect mitochondria from oxidative damage [76]. In the soleus muscle of the 4-week-old male Wistar rats fed on HFD for 14 days, a significantly lower total GSH level was observed, although there were no significant changes in the expression of GPx, CAT, and manganese (Mn)-SOD [77]. A fructose-enriched diet induces the development of IR in the white adipose tissue of the adult female Wistar rats, although it did not lead to obesity and systemic IR. Thus, the protein levels of SOD1 and GR were increased in white adipose tissue of fructose-fed female rats, compared to the control group, which probably serve to prevent intracellular RONS accumulation and oxidative damage of macromolecules [78]. However, as obesity progresses, the amount and activity of the AOS components become depleted, increasing susceptibility to oxidative tissue damage [79]. The model of genetically obese (fa/fa) Zucker rats (ZR) share many features with human MS, such as obesity, IR, and hyperlipidemia [80]. In the study of Martinelli et al. [30], SOD activity was decreased in the plasma samples and the heart of obese male ZR compared to their lean littermates, while the activity of GPx was not significantly changed [30]. Additionally, the analysis showed an increase of oxidized proteins concentration and the expression of lipid-aldehyde 4-hydroxynonenal in the heart of obese male ZR. Increased pro-oxidative elements and the decreased components of AOS indicate a condition of obesity-related OxS in obese male ZR [30]. In male mice fed an HFD, the hepatic contents of GSH, GPx, and GR were significantly decreased [31], whereby serine supplementation increased the content of cysteine, one of the major determinants of GSH synthesis, thus protecting the liver against HFD-induced dysfunction of the GSH AOS. Krautbauer et al. [29] showed that the protein level of Mn-SOD is reduced in the liver of HFD-fed male mice [29]. In the visceral adipose tissue of young female Wistar rats, 9-week fructose-enriched diet led to a significant reduction of the protein levels of Mn-SOD and GPx, while protein levels of Cu/Zn-SOD and GR were unchanged [81]. Madani et al. [82] demonstrated that a fructose-rich diet led to a considerable increase of the plasma triglycerides and FFA levels, development of IR, and a substantial decrease of CAT, SOD, and GPx activities in adipose tissues in male Wistar rats. In male Albino rats, a high-fat and carbohydrate diet (HFCD) led to induction of IR, the increase of the malondialdehyde (MDA) level, and the decrease of GSH level. Moreover, the administration of the insulin-sensitizing drug pioglitazone to HFCD-induced IR rats reduced MDA level and improved GSH level, compared to the non-treated IR-induced group of rats [83].
AOS in obesity and IR in animal model studies
Animal tissue/cell type | Components of AOS defense | Effect of obesity/IR | Ref |
---|---|---|---|
3T3-L1 adipocytes of mice | EC-SOD expression | Increased | [75] |
Perirenal, epididymal, and brown adipose tissues of male Wistar rats | SOD, CAT, GPx activities | Decreased | [82] |
Liver of male C57BL/6 mice | Mn-SOD protein level | Decreased | [29] |
Heart of male C57BL/6J mice | CAT protein level/CAT activity | Increased | [76] |
Visceral adipose tissue of female Wistar rats | Mn-SOD, GPx protein levels/Cu/Zn-SOD, GR protein levels | Decreased/unchanged | [81] |
Primary hepatocytes of male C57BL/6J mice | GSH content, GPx, and GR activities | Decreased | [31] |
Soleus muscle of Wistar male rats | Total GSH level/Mn-SOD, CAT, GPx expression | Decreased/unchanged | [77] |
Plasma samples and heart of male fa/fa ZR | SOD/GPx activities | Decreased/unchanged | [30] |
Serum of male Albino rats | MDA level/GSH level | Increased/decreased | [83] |
White adipose tissue of female Wistar rats | SOD1 and GR protein levels | Increased | [78] |
Ref: reference
Evidence from human studies
There is mounting evidence that attenuation of antioxidant enzymes and increased RONS production in obese subjects may contribute to further complications in obesity-related IR (Table 2). Irie et al. [33] found a significant decrease in serum GSH levels in male and female patients with non-alcoholic fatty liver disease, which can reduce the productivity of GSH-related antioxidant enzymes, such as GPx and GR. Depletion of GSH and reduced activities of GPx and GR have also been found in the serum of female T2DM patients [32]. A study showed that treating male and female T2DM patients with liraglutide led to an increase in serum GSH levels and a decrease of serum lipid hydroperoxides, thus reducing OxS in these patients [84]. In obese women, GPx activity significantly increases in the serum after mass body reduction [85]. In erythrocytes of obese women, Cu/Zn-SOD, GPx, and CAT activities were significantly lower compared to the same cell type of a normal-weight group [86]. In adipose tissue of obese male and female T2DM patients and non-diabetic obese subjects and non-obese diabetic subjects, the mitochondrial Mn-SOD and GPx show decreased activities [87]. In peripheral blood mononuclear cells of obese children of both sexes, Mn-SOD and CAT gene expressions were significantly reduced compared to normal-weight children [34]. Obese children showed significantly higher levels of OxS markers MDA and 3-nitrotyrosine, and increased SOD activity compared with normal-weight children. Meanwhile, CAT concentration and the GSH/oxidized glutathione (GSSG) ratio correlated negatively with BMI. However, in children with overweight, SOD, CAT, and GSH/GSSG all correlated negatively with BMI [88]. In male and female patients with MS, the levels of oxidative markers, substances reactive to thiobarbituric acid and carbonyl protein, were increased, while the non-enzymatic antioxidants vitamin C and GSH were decreased [89]. Several experimental and clinical studies have shown a decrease in the serum paraoxonase (PON)-1 activity in obese subjects, which is positively correlated with BMI [90]. The PON family of antioxidant enzymes has an essential role in cardiovascular diseases and diabetes mellitus associated with obesity. PON-1 protects low-density lipoproteins and circulating cells against oxidative damage, preventing inflammatory responses in the arterial wall [91]. Selenium (Se) and Zn, which are important cofactors for GPx and SOD activities, are decreased in obese children and adolescents of both sexes [92, 93]. Magnesium (Mg), Se, Zn, and iron (Fe) have been reported deficiently in morbidly obese patients [94]. In the study by Aasheim and Bohmer [95], morbidly obese male and female patients have the most noticeable reduction in vitamins A, B6, C, D, and E. The cross-sectional study of Barzegar-Amini et al. [96] showed a significantly lower serum level of vitamin E in male and female patients with MS than patients without MS. Low carotenoids, vitamins C and E are related to increased BMI [97–99].
AOS in obesity and IR in human studies
Human tissue/cell type | Components of AOS defense | Effect of obesity/IR | Ref |
---|---|---|---|
Serum of obese women | GPx activity | Decreased | [85] |
Plasma of obese children of both sexes | Zn concentration | Decreased | [93] |
Serum of morbidly obese male and female patients | Vitamins A, B6, C, D, E concentrations | Decreased | [95] |
Serum of obese adolescents | Se concentration | Decreased | [92] |
Erythrocytes of obese women | Cu/Zn-SOD, CAT, GPx activities | Decreased | [86] |
Serum of male and female T2DM patients | GSH content/GPx, GR activities | Decreased | [32] |
Adipose tissue of obese and/or T2DM male and female patients | Mn-SOD, GPx activities | Decreased | [87] |
Serum and liver of male and female NAFLD patients | GSH level | Decreased | [33] |
Peripheral blood mononuclear cells of obese children of both sexes | Mn-SOD, CAT expression | Decreased | [34] |
Serum of MS male and female patients | Vitamin E concentration | Decreased | [96] |
Blood samples of obese and overweight children | SOD activity/GSH/GSSG ratio, CAT activity | Increased/decreased (in obese); all decreased (in overweight) | [88] |
Blood samples of MS male and female patients | Vitamin C, GSH levels | Decreased | [89] |
NAFLD: non-alcoholic fatty liver disease
Conclusions
OxS has been implicated in the development of comorbidities in obesity and could be an early marker of metabolic dysfunction in obesity-related IR. Furthermore, obesity per se may induce systemic OxS, and increased OxS in accumulated adipose tissue is, at least in part, the underlying cause of adipocytokine dysregulation and MS development [100]. The excess supply of energy substrates to metabolic pathways in obesity may increase mitochondrial dysfunction and RONS production [101]. Notwithstanding, RONS are essential signaling molecules, if not well controlled, they can cause damage to cellular proteins, lipids, and DNA, potentially having detrimental effects on functions. While mounting evidence suggests that RONS overproduction in obesity leads to altered signaling and IR, other data reported that RONS is essential for insulin secretion by β-cells as well as insulin sensitivity [102, 103]. Increased OxS in accumulated adipose tissue should be an imperative target for developing new therapies in obesity-related IR. A wide-ranging approach designed to decrease oxidation markers and improve antioxidant defenses in obese subjects includes weight loss associated with physical activity and different dietary factors, which could be helpful to prevent and treat obesity comorbidities.
Abbreviations
AOS: | antioxidant defense system |
BMI: | body mass index |
CAT: | catalases |
Cu: | copper |
FFAs: | free fatty acids |
GPx: | glutathione peroxidases |
GR: | glutathione reductases |
GSH: | glutathione |
GSSG: | oxidized glutathione |
HFD: | high-fat diet |
IL-1: | interleukin-1 |
IR: | insulin resistance |
MDA: | malondialdehyde |
Mn: | manganese |
MS: | metabolic syndrome |
NADPH: | reduced nicotinamide adenine dinucleotide phosphate |
NFκB: | nuclear factor kappa B |
OxS: | oxidative stress |
PON: | paraoxonase |
RONS: | reactive oxygen and nitrogen species |
Se: | selenium |
SOD: | superoxide dismutases |
T2DM: | type 2 diabetes mellitus |
TNF-α: | tumor necrosis factor-alpha |
UPR: | unfolded protein response |
Zn: | zinc |
ZR: | Zucker rats |
Declarations
Author contributions
AP and JS wrote the manuscript and contributed conception. ERI and ESM designed, wrote, and supervised the manuscript. All authors contributed to manuscript revision, read and approved the submitted version.
Conflicts of interest
The authors declare that they have no conflicts of interest.
Ethical approval
Not applicable.
Consent to participate
Not applicable.
Consent to publication
Not applicable.
Availability of data and materials
Not applicable.
Funding
This work was funded by the Ministry of Education, Science and Technological Development of the Republic of Serbia (Contract No#451–03–9/2021–14/200017). The funder had no role in the study design, data collection and analysis, decision to publish, or preparation of the manuscript.
Copyright
© The Author(s) 2022.