Abstract
Around the world, more than 6.2 million individuals have died as a result of coronavirus disease 2019 (COVID-19). According to a recent survey conducted among immunologists, epidemiologists, and virologists, this disease is expected to become endemic. This implies that the disease could have a continuous presence and/or normal frequency in the population. Pharmacological interventions to prevent infection, as well as to treat the patients at an early phase of illness to avoid hospitalization are essential additions to the vaccines. Taurine is known to inhibit the generation of all inflammatory mediators linked to the cytokine storm. It can also protect against lung injury by suppressing increased oxidants production and promoting the resolution of the inflammatory process. Neutrophil lactoferrin degranulation stimulated by taurine may have antiviral effects against SARS-CoV-2, limiting viral replication. It is hypothesized that if taurine is administered early in the onset of COVID-19 disease, it may stop the cytokine storm from progressing, lowering morbidity and mortality.
Keywords
COVID-19, cytokine storm, efferocytosis, hypochlorous acid, macrophage polarization, myeloperoxidase, SARS-CoV-2, taurineIntroduction
In its critical stage, the severe acute respiratory syndrome coronavirus 2 (SARS-CoV-2) may provoke deadly pneumonia [1, 2]. Although coronavirus disease 2019 (COVID-19) caused by SARS-CoV-2 infection is characterized by a mortality rate of 2.2% [3], this disease is more severe in aged patients and patients with underlying maladies [4–6]. For example, the mortality rate of patients with underlying health conditions was shown to reach 12% [4]. Furthermore, in COVID-19 patients who developed acute respiratory distress syndrome (ARDS), the mortality rate can be as high as 40% to 50% [7, 8]. Even though the factors involved in COVID-19-mediated lung damage remain mostly unknown, in the published research, the phrase “cytokine storm”; i.e., a hyper-inflammatory state originating from the uncontrolled release of cytokines by a dysregulated immunological system has been frequently associated with the related pathological alterations [9–37]. In general terms, it represents an overactive immunological reaction distinguished by the production of interferon, interleukins, tumor necrosis factors, chemokines, and many other mediators, such compounds are core components of a well-preserved innate immunological response essential for the effective removal of pathogens. The term “cytokine storm” refers to a scenario in which several cytokines released are harmful to the host [9, 38].
One of the most dangerous COVID-19 complications is the emergence of a severe upper respiratory lung disease, which presents a big challenge to physicians in terms of illness treatment [39]. The majority of sick individuals with SARS-CoV-2 infection experienced minor symptoms, according to a study of 41 patients with COVID-19, however, some of them later displayed serious symptoms and eventually died due to organic failure as a consequence of an excessive cytokine storm [2]. It is worth noting that the production of numerous cytokines is closely linked to the onset of the first symptoms. For example, interferon-alpha (IFN-α) release causes fever, headaches, chills, drowsiness, and tiredness. Tumor necrosis factor-alpha (TNF-α) can induce symptoms similar to the flu, but it can also cause vascular fragility, heart disease, and lung injury. Interleukin 6 (IL-6) release may cause complement activation and clotting cascade stimulation, causing severe symptoms including disseminated intravascular coagulation [40–42].
It is interesting to note that IL-6 may cause cardiomyopathy by increasing cardiac failure, which is common in cytokine release syndrome (CRS) patients. Furthermore, endothelial cell activation could be one of the symptoms of critical CRS [43]. Malfunctions in the endothelia are characterized by capillary leakage, low blood pressure, and coagulation alterations [44]. These data show that immunopathological mechanisms induced by SARS-CoV-2 are important in fatal pneumonia that occurs after coronavirus infections [45]. A cytokine storm is defined as “a potentially deadly immune disorder characterized by accelerated growth and enhanced activation of T cells, macrophages, and natural killer cells, and also elevated release of over 150 inflammatory cytokines and signaling molecules released by immune and non-immune cells” [46]. In viral diseases, aberrant inflammatory cytokine release causes epithelial and endothelial cell death, affecting lung microcirculation and alveolar epithelial cell permeability, and resulting in vascular bleeding, alveolar edema, and hypoxia [39]. Other organ systems affected by COVID-19 outside the lungs include the heart, kidneys, vascular system, liver, and brain [47].
In addition, it is well known that patients with COVID-19 may have a worse prognosis if their blood glucose levels are high, increasing the likelihood of mechanical ventilation, shock, and multiple organ failure, requiring intensive care unit (ICU) therapy [48]. During COVID-19 infection, people with type 2 diabetes mellitus had a higher incidence, severity of symptoms, and mortality [49]. According to current findings, glycemic management has a significant impact on the effectiveness of the immunological response in patients receiving a messenger ribonucleic acid (mRNA) vaccine against SARS-CoV-2 [50]. Certainly, hyperglycemia during vaccination impairs the immune response: a recent investigation found that at 21 days after the first vaccine dose, neutralizing antibody titers and CD4 cytokine responses involving type 1 helper T cells were lower in type two diabetic patients with glycosylated hemoglobin (HbA1c) levels > 7% than in individuals with HbA1c levels ≤ 7% [50].
In patients with acute myocardial infarction, the significance of SARS-CoV-2 in causing endothelial dysfunction and enhanced coagulation is well documented [51]. Furthermore, enhanced angiotensin-converting enzyme 2 (ACE2) glycation and transmembrane serine protease 2 (TMPRSS2) expression in cardiac myocytes as a result of diabetes mellitus have been shown to facilitate SARS-CoV-2 entry in the host cell; as a consequence, about 50% of COVID-19 patients with diabetes mellitus who were hospitalized experienced myocardial injury [52].
Taurine is one of the most prevalent non-essential amino acids in mammals, with several physiological activities in the neurological, cardiovascular, renal, endocrine, and immunological systems. Inflammation causes taurine to be halogenated in phagocytes, resulting in taurine chloramine (TauCl) synthesis. It is formed in active neutrophils by a reaction with hypochlorous acid (HOCl) produced by the halide-dependent myeloperoxidase (MPO) system. Following apoptosis, TauCl is released from active neutrophils and inhibits the synthesis of inflammatory mediators such as superoxide anion, nitric oxide, TNF-α, interleukins, and prostaglandins in inflammatory cells and tissues. TauCl also stimulates the expression of antioxidant proteins in macrophages, including heme oxygenase 1, peroxiredoxin, thioredoxin, glutathione peroxidase, and catalase [53]. Because taurine protects many tissues from oxidative stress [54–58], the scientific rationale for employing taurine as a therapeutic in the early stages of COVID-19 will be presented in this work.
The neutrophil leukocytes function in COVID-19 disease
The pathological changes associated with severe COVID-19 disease are characterized by neutrophil quantity, phenotype, and performance alterations. Following SARS-CoV-2 infection, an increased number of neutrophils were found in the nasopharynx [59] and after in the more distant areas of the lung [60]. Elevated neutrophil number in the bloodstream has been recognized as a marker of COVID-19 [8, 61], and neutrophil activation pathways are major components of the severe cases [62, 63]. The neutrophil-to-lymphocyte ratio (NLR), a recognized biomarker of infection and generalized inflammation, has shown that COVID-19 patients have an increased inflammatory reaction [8].
Because acute respiratory distress syndrome is the most frequent cause of death in COVID-19 sufferers, high NLR rates predict a grave prognosis [64]. When severe COVID-19 patients who were sent to the ICU were compared to other COVID-19 patients who were not sent to the ICU, the former patients had low lymphocyte count and increased neutrophil number and NLR, according to a recent study [65]. Furthermore, some COVID-19 sick people also showed an increased number of neutrophils and a decreased lymphocyte number through the critical stage [45]. Pulmonary capillaries of the COVID-19 patients had considerable neutrophil infiltration, according to another research [66].
The role of MPO and HOCl in the cytokine storm
MPO, or myeloperoxidase, is an enzyme found mostly in neutrophils and macrophages [67]. When chemically reacts with superoxide, hydrogen peroxide, and chloride, MPO induces the synthesis of HOCl [67]. Although the MPO/HOCl complex is required for phagocytes to kill bacteria and viruses, MPO can be released outside the cell, increasing the risk of tissue harm [68]. HOCl is the oxidant with the highest reactivity and generated in considerable amounts in our bodies, and has a reaction that is many times greater with most substrates than hydrogen peroxide, hydroperoxides, and peroxynitrite [69]. HOCl has been shown in vitro to increase nuclear factor-kappa B (NF-κB) activity, the attachment of a phosphoryl group to tyrosine in T and B lymphocytes, calcium signaling, and TNF-α production [70, 71].
Many COVID-19 patients, especially the elderly, who eventually developed respiratory failure, had hypoxemia and hypocapnia without any indications of respiratory distress [72]. This condition is also called “happy hypoxemia” or “quiet hypoxemia”, and it was first seen in patients during the Wuhan outbreak [72]. The exact cause of this asymptomatic hypoxia is unknown, although the accumulation of HOCl in the blood during COVID-19 has been suggested to cause tissue hypoxia due to a lack of normal hemoglobin (Hb) activity [73]. Interestingly, it is notorious that Hb has a higher affinity for HOCl than for O2; that is, HOCl acts as a competitive inhibitor with O2 for heme binding sites, thus resulting in a decreased percentage of oxygen in the blood [73, 74]. Furthermore, HOCl is involved in a plethora of oxidative reactions, including iron oxidation of the Hb-heme complex, heme breakdown, and the final liberation of free iron, which together promote harmful tissue damage by generating reactive oxygen species (ROS) and using nitric oxide (NO). Linking these reactions in an integral model might explain the significant cellular injury, vascular constriction, and extreme hypoxemia as reported in COVID-19 sick people with clinical worsening [73].
Notably, taurine has been shown to prevent MPO degranulation selectively, safeguarding organs from oxidative harm caused by exaggerated MPO release. This inhibition of MPO release produced a significant reduction in neutrophil extracellular traps (NETs) [75].
NETs are extracellular webs of DNA, histones, microbicidal proteins, and oxidant enzymes that are released by neutrophils to control infections; however, when not properly regulated, NETs have the potential to initiate and propagate inflammation, thrombosis [76, 77], and respiratory arrest [78, 79]. SARS-CoV-2 does cause, in fact, a prothrombotic condition with dangerous consequences including deep vein thrombosis [80]. Increased NETs, which can propagate inflammation and microvascular thrombosis in individuals with SARS-CoV-2 infection, are primarily responsible for this outcome [81, 82]. In addition, SARS-CoV-2 infection has been shown to directly cause NETs formation and release in healthy neutrophils, which induces lung epithelial cell death in vitro [81].
The discovery that taurine-mediated inhibition of MPO release produced a significant reduction in NETs is transcendental [75] since NETs generation was discovered to be increased in severe COVID-19 cases, and it is linked to immuno-thrombosis and the development of acute lung injury/acute respiratory distress syndrome (ALI/ARDS) [83]. Of note, inhibition of excessive neutrophil function and NETs formation by an anti-MPO antibody was protective in various models of influenza-associated ARDS [84].
Taurine reprograms macrophage M1 polarization by inhibiting mitophagy
Alveolar macrophages are known to remove billions of air pollutants, allergens, and microorganisms per day [85, 86]. Macrophages may shift into M1 or M2 phenotypes to preserve alveolar homeostasis. Macrophages have been classified as “polarized” either towards M1 mode (proinflammatory, regulating pathogen resistance) or M2 mode (anti-inflammatory, facilitating tissue remodeling) (Figure 1) [86, 87].
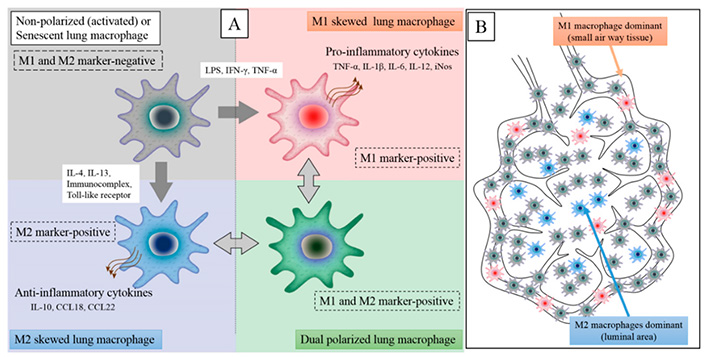
The M1 and M2 macrophage polarization states have distinct phenotypic properties. An inflammatory stimulus in the lungs, such as virus infection, lipopolysaccharide (LPS), dust particles, or IFN-γ, causes local macrophages to transition from M2 to M1 phenotype. Furthermore, circulating monocytes will be attracted into lung tissues and activated by the inflammatory stimulation, with M1 macrophages accounting for the majority of macrophages in the inflammatory zone (M1 dominant). Inducible nitric oxide synthase (iNOS), IL-6, IL-12, IL-1β, and TNF-α are inflammatory cytokines secreted by M1 macrophages. M2 macrophages release anti-inflammatory cytokines: C-C motif ligand 18 (CCL18), CCL22, and IL-10
Note. Adapted from “Lung macrophage phenotypes and functional responses: role in the pathogenesis of COPD,” by Yamasaki K, van Eeden SF. Int J Mol Sci. 2018;19:582 (https://doi.org/10.3390/ijms19020582). CC BY.
The CD206 receptor is routinely expressed by alveolar macrophages, according to the results of a blood cytometry study of 72 donors without lung pathology [88]. Considering that CD206 is a characteristic M2 marker, human alveolar macrophages appear to be biased toward an M2-like phenotype, which is not ideal for virus propagation [89]. SARS-CoV-2 sabotages alveolar macrophages for its reproduction and dissemination, leading to enhanced pathology in the lungs; it was also discovered that SARS-CoV-2 may induce the M1 profile in alveolar macrophages by releasing inflammatory cytokines [89].
These cytokines, such as TNF-α, may promote macrophage polarization toward an M1-like state [90, 91]. Interestingly, the reduction of CD206+ M2 macrophages in vivo exacerbates acute lung damage, which is associated with neutrophil migration to the lungs [92]. If the viral infection in the alveoli surpasses a certain threshold, SARS-CoV-2 may directly reprogram alveolar macrophages to an M1 phenotype, and the resulting pro-inflammatory cytokines [93] may further promote M1 polarization, allowing viral transmission via alveolar macrophages [89]. In addition, bronchoalveolar liquid from patients with severe COVID-19 disease had greater M1 macrophage counts, but such fluid from less severe infected people and healthy controls had a higher M2 macrophage count [60].
High intracellular MPO activity is required for macrophage activation [94], and MPO-mediated HOCl production has been demonstrated to be a major cause of macromolecular oxidative injury as reported in several investigations [69, 95, 96]. Concretely, cellular contact with HOCl can have a variety of negative consequences by affecting the cellular redox state [97], so avoiding more HOCl generation during the cytokine storm is critical to controlling excessive MPO activity [73]. In this regard, it is known that TauCl “inhibits macrophage production of inflammatory mediators like macrophage inflammatory protein-2 (MIP-2), monocyte chemoattractant protein-1 and 2 (MCP-1 and 2), nitric oxide, nitrites, prostaglandin E2 (PGE2), TNF-α, and IL-6” [98–101]. Monocytes and macrophages are transformed from an inflammatory (M1) to a basal (M2) phagocytic state by these down-regulation pathways [102].
Finally, a recent work discovered that taurine suppresses macrophage M1 polarization by inhibiting mitophagy (mitochondrial autophagy) and increasing the synthesis of M2-markers (CD206 and IL-10) by decreasing the production of M1 inflammatory factor genes [103]. Without an adequate number of mitochondria, macrophages turn to glycolytic metabolism, which is linked to the M1 phenotype; this change is triggered in alveolar macrophages by SARS-CoV2, which favors viral replication [89]. Excessive M1 polarization thus induces a pro-inflammatory state, that can develop in a cytokine storm if it is not adequately managed [20]. Taurine reprograms the metabolic activity of M1 macrophages by sustaining a high number of mitochondria; this restricts the transition of energy metabolism to glycolysis, which is essential for the metabolism of M1 macrophages [103]. All of these findings lead us to propose that if taurine is given early in the disease, it may be able to stop the cytokine storm that is linked with severe COVID-19 cases.
Taurine neutralizes HOCl-induced tissue damage
HOCl is formed within the phagocytic vacuole when neutrophils internalize and destroy bacteria or viruses, and while it is a potent bactericide, it can also be hazardous since it oxidizes many biological components [104]. Taurine chemically reacts with HOCl in leukocytes to produce TauCl (a more balanced, less aggressive, and specific oxidant compared with HOCl) [105–107]. In biological systems, TauCl is thought to operate as a general antioxidant (scavenger), protecting cells from self-destruction during activities that create excessive HOCl [100]. Taurine may also protect epithelial cells of the lungs against HOCl/hypochlorite (OCl–)-mediated lysis by lowering chlorination of cell components, rather than preventing sulfhydryl group oxidation [108].
Notably, TauCl promotes nuclear factor erythroid 2 (Nrf2) to enter the nucleus and triggers the production of several anti-oxidant proteins [109], and prevents the generation of inflammatory cytokines [110, 111]. Because NF-κB is known to influence inflammatory cytokine gene production [112], TauCl is thought to decrease NF-κB activation. The chemical mechanism underpinning TauCl-induced suppression of NF-κB activation was unknown at the time, but it was eventually discovered to be oxidization of the inhibitor of nuclear factor kappa B alpha protein (IκBα) at methionine45 [112].
Taurine protects against lung damage by mitigating oxidative stress
Taurine is a functionally important molecule, and it has been discovered to be a powerful cell guardian, lowering oxidative stress and inflammatory factor production [113, 114]. Taurine pre-treatment of rats before acute ozone exposure reduces oxidant-mediated lung damage by preventing the generation of nitrite and the release of TNF-α, which are both related to tissue damage [101]. Taurine has been shown to counteract paraquat-mediated reductions in glutathione (GSH) level and GSH peroxidase function in other studies [113], inhibits oxidative stress-mediated cell apoptosis and also lowers malondialdehyde (MDA) production [115]. In research mimicking limb ischemia reperfusion-induced lung damage, taurine increased superoxide dismutase (SOD) and catalase functions by decreasing stress in the endoplasmic reticulum [115].
Taurine also synergized the protective effect of dexmedetomidine against sepsis-induced lung damage by blocking the NF-κB pathway and lowering IL-6 and IL-1 expression levels [114]. In a recent study, taurine’s beneficial effects on sepsis-mediated lung injury were investigated, and it was discovered that taurine administration reduced sepsis-mediated elevations in TNF-α and IL-1 expression in lung cells. These beneficial effects were linked to the control of inflammation and oxidant stress via interruption of the p38/mitogen-activated protein kinase (MAPK) and NF-κB regulatory pathways [116].
Acute lung damage is a frequent manifestation of Gram-negative bacterial infections and a primary origin of disease complications and deaths in humans [117]. The internal toxin LPS, which is located in the external membranes of bacteria, is a frequent cause of ALI [118]. In contrast to control animals’ lungs, LPS-treated mice’s lungs exhibited increased cellular apoptosis, lipid peroxidation, reduced concentrations of glutathione catalase, glutathione peroxidase, and superoxide dismutase, and inflammation limited to the parenchyma [119]. All of these changes were greatly reduced after taurine supplementation, showing that this amino acid has anti-inflammatory and antioxidant capabilities that help defend the lungs from the toxic effects of Gram-negative bacterial endotoxin [119].
TauCl contributes to the resolution of inflammation
The death of neutrophils is required for inflammation to be resolved; however, this does not always occur, and neutrophils keep driving the inflammatory process [120]. MPO has been found to exert an unforeseen function in affecting neutrophil destiny and, as a result, the persistence of inflammation—MPO extended the lifespan of neutrophils by regulating the constitutive cell death pathway (apoptosis), postponing the resolution of inflammation [120]. Individuals suffering from sepsis-associated ARDS were recently discovered to have dysfunctional alveolar macrophage efferocytosis (phagocytic engulfment of apoptotic neutrophils), thus provoking an important inflammatory reaction as the result of subsequent neutrophil necrosis, which also leads to a severe clinical course, including death [121].
Previous research has established that TauCl stimulates the resolution of inflammation by boosting efferocytosis [122] and decreasing MPO production by neutrophils [75]. Macrophages secrete more IL-10, a cytokine that modulates excessive inflammatory reactions, and less of the cytokines that promote inflammation, like the TNF-α, IL-1, and IL-12 during efferocytosis [123]. A contemporary study demonstrated that sick people with COVID-19 had fewer airway regulatory T cells (Tregs) than healthy controls, reinforcing the hypothesis that a Treg deficit at the lung location is contributing to more severe illness [124]. Interestingly, taurine has been recently shown to increase the generation of CD4+CD25+FOXP3+ Tregs [125].
TauCl stimulates lactoferrin release
Acute inflammation is the body’s active reaction to microbial pathogens and physical trauma; when neutrophils are drawn to an inflamed region, they get active and experience an oxidative burst, which is a key event in the protective immunity. As a result, there are abundant reactive oxygen species, of the kind that neutrophil leucocytes use to destroy and remove pathogenic microorganisms [122]. The major molecule MPO, second molecule lactoferrin (LF), and third molecule matrix metalloproteinase (MMP)-9 are discharged to the fluid that surrounds cells during degranulation [75].
Neutrophil LF degranulation is also induced by TauCl [75]. LF is a glycoprotein present in a variety of excretory fluids from mammals, and within neutrophil secondary granules [126]. LF exhibits antibacterial and antiviral effects on a wide range of pathogens, including several kinds of bacteria, as well as encapsulated and non-encapsulated viruses [127–129]. LF is an antiviral molecule that works mostly during the initial stage of infection but also at the internal cellular level (for example, during hepatitis C infection) [130–132]. According to the generally accepted framework, LF can prevent SARS-CoV-2 infection of permissive cells by inhibiting the spike protein attachment, adhesion to ACE2 receptors, or by exerting a competitive inhibition with molecules such as heparan sulfate and sialoside glycosaminoglycans, or by impairing viral replication. LF may be able to inhibit the triggering of the cytokine storm cascade, preventing systemic effects and illness worsening, due to its high ability to penetrate cells and get into the nucleus [126].
Discussion
If adequate therapy is not given at the proper moment, the COVID-19 disease can be lethal. According to research findings, “approximately 20% of COVID-19 patients tend to have a severe or critical disease, with a mortality rate of 50% or more in critical cases” [133–135]. The polarization (change from the M2 to the M1) of alveolar macrophages caused by SARS-CoV-2 could disrupt efferocytosis, preventing neutrophil death and perpetuating the inflammatory process [121, 136]. It has been postulated that much of the damage to lung cells in complicated COVID-19 cases is associated with an increase in HOCl generation by neutrophils and M1 macrophages rather than by the virus itself [73]. Taurine is a powerful scavenger for HOCl, and so neutralizes its harmful effects, according to compelling data [100]. Taurine also restricts the generation of all pro-inflammatory molecules linked to the cytokine storm, prevents damage to the lungs by decreasing oxidative stress, and promotes the resolution of the inflammatory process [98–101]. Neutrophil LF degranulation driven by taurine may have direct antiviral actions against SARS-CoV-2, suppressing viral replication [126].
Several studies have reported that taurine levels in mild/moderate COVID-19 patients are usually higher during active disease compared to healthy subjects [137], while others have found decreased taurine levels in asymptomatic, mild, moderate, severe, and critical patients [138]. The latter authors proposed that because taurine is abundant in leucocytes, a lack of it is thought to impair immune cell activity, thus in COVID-19 physiopathology, sepsis is comparable to the cytokine storm, and the low taurine level in this study could be one of the causes of the aberrant immune reaction in COVID-19 sufferers [138]. Our explanation of the different taurine levels found in COVID-19 patients is that during the acute phase, taurine levels increase as a response directed at trying to neutralize excessive oxidative damage. Taurine concentration in organs is derived from two sources: biosynthesis and diet. Most severely affected patients do not feed correctly, so the taurine’s external supply is compromised. Furthermore, it has been reported that hyperglycemia downregulates the human taurine transporter [139]. Interestingly, a group of researchers discovered that an increased glucose concentration significantly promoted viral proliferation and inflammatory cytokine production, demonstrating the essential role of glycolysis. These findings can explain why the uncontrolled serum glucose levels in diabetes patients are a determinant factor for complicated COVID-19 cases [140]. So, during the severe and critical phases, taurine levels could be depleted by SARS-CoV-2-induced hyperglycemia, thus explaining the decreased taurine levels found in several studies. During the recovery phase, inflammation is controlled and taurine levels gradually increase, except in long COVID-19 patients.
Based on the data reported in this review, we propose that taurine may be able to prevent the cytokine storm from occurring if administered at the early COVID-19 stages. Interestingly, taurine derivatives like taurolidine reduce inflammation in peripheral blood mononuclear cells by inhibiting the release of the proinflammatory cytokines TNF-α, IL-1β, IL-6, and IL-8; and a previous work postulated that taurine derivatives should be considered a promising available treatment strategy in the management of COVID-19 patients [141]. To test the validity of this hypothesis, clinical trials are required.
Abbreviations
ARDS: | acute respiratory distress syndrome |
COVID-19: | coronavirus disease 2019 |
Hb: | hemoglobin |
HOCl: | hypochlorous acid |
ICU: | intensive care unit |
IL-6: | interleukin 6 |
LF: | lactoferrin |
LPS: | lipopolysaccharide |
MPO: | myeloperoxidase |
NETs: | neutrophil extracellular traps |
NF-κB: | nuclear factor-kappa B |
NLR: | neutrophil-to-lymphocyte ratio |
SARS-CoV-2: | severe acute respiratory syndrome coronavirus 2 |
TauCl: | taurine chloramine |
TNF-α: | tumor necrosis factor-alpha |
Tregs: | regulatory T cells |
Declarations
Author contributions
ARC and EMR contributed to conceptualization and project design. ARC, RCG and RB contributed to the literature analysis. VNU, EMR and RB contributed to the acquisition and interpretation of data, data curation, formal analysis, writing, and review of the manuscript. All authors have read and agreed to the published version of the manuscript.
Conflicts of interest
The authors declare they have no conflicts of interest.
Ethical approval
Not applicable.
Consent to participate
Not applicable.
Consent to publication
Not applicable.
Availability of data and materials
Not applicable.
Funding
Not applicable.
Copyright
© The Author(s) 2022.