Abstract
Chronic kidney disease (CKD) is a worldwide public health issue and has ultimately progressed to an end-stage renal disease that requires life-long dialysis or renal transplantation. However, the underlying molecular mechanism of these pathological development and progression remains to be fully understood. The human gut microbiota is made up of approximately 100 trillion microbial cells including anaerobic and aerobic species. In recent years, more and more evidence has indicated a clear association between dysbiosis of gut microbiota and CKD including immunoglobulin A (IgA) nephropathy, diabetic kidney disease, membranous nephropathy, chronic renal failure and end-stage renal disease. The current review describes gut microbial dysbiosis and metabolites in patients with CKD thus helping to understand human disease. Treatment with prebiotics, probiotics and natural products can attenuate CKD through improving dysbiosis of gut microbiota, indicating a novel intervention strategy in patients with CKD. This review also discusses therapeutic options, such as prebiotics, probiotics and natural products, for targeting dysbiosis of gut microbiota in patients to provide more specific concept-driven therapy strategy for CKD treatment.
Keywords
Chronic kidney disease, gut microbiota, immunoglobulin A nephropathy, diabetic kidney disease, membranous nephropathy, prebiotics, probiotics, natural productsIntroduction
Chronic kidney disease (CKD) is a major public health problem worldwide [1–3]. According to the Kidney Disease Outcomes Quality Initiative, CKD is divided into five stages. Stage 1 is defined by altered renal function (changed markers containing aberrant blood or urine tests or imaging analysis) with normal or raised glomerular filtration rate ≥ 90 mL/min per 1.73 m2; stage 2 is characterized by glomerular filtration rate 60–89 mL/min per 1.73 m2 with evidence of kidney injury; stage 3 glomerular filtration rate 30–59 mL/min per 1.73 m2; stage 4 glomerular filtration rate 15–29 mL/min per 1.73 m2; stage 5 end-stage renal failure with glomerular filtration rate < 15 mL/min per 1.73 m2 [4]. Renal fibrosis including tubulointerstitial fibrosis and glomerulosclerosis is the final outcome of CKD [5–7]. The progression of CKD indicates that patients who irreversibly reach end-stage renal disease (ESRD) require renal replacement therapies such as dialysis and transplantation [8–14]. CKD causes the progressive and irreversible nephron loss that leads to glomerular sclerosis, as well as progressive interstitial fibrosis and tubular injury, and ultimately further reductions in nephron numbers [15–17]. Irrespective of the initial etiology, tubular cell atrophy and interstitial fibrosis are cardinal pathological features of CKD. Renal fibrosis has a typical feature of excessive accumulation and deposition of extracellular matrix components [18]. In terms of underlying molecular mechanism, renal fibrosis is associated with the activated pathways, such as transforming growth factor-β/Smad, Wnt/β-catenin, inhibitory of kappa B (IκB)/nuclear factor kappa B (NF-κB), and Keap1/nuclear factor erythroid 2–related factor 2 (Nrf2) signaling pathways [19–23], as well as the activated cytokines, such as renin-angiotensin system and aryl hydrocarbon receptor [24–27] and the dysregulation of metabolites such as amino acids and lipids [28–30]. However, the underlying molecular characteristics of these pathological development and progression remain to be fully understood. In recent years, increasing evidence has indicated that the dysbiosis of gut microbiota is involved in CKD including immunoglobulin A (IgA) nephropathy, diabetic kidney disease (DKD), membranous nephropathy (MN), chronic renal failure (CRF) and ESRD patients [31–38]. Current review summarizes the dysbiosis of gut microbiota in patintestinal mucosal barrier, allowing live bacteria or their metabolitesients with CKD that reveals the human disease development and progression. This review also discusses therapeutic approaches for modulating microbial dysbiosis in patients to provide more specific concept-driven therapy strategy for CKD interventions.
Microbiome and gut microbiota
The human gut is extremely populated with microorganisms, dominantly anaerobic bacteria. This internalized ‘microbial organ’ is not encoded in the host genome. Currently, human microbiome is estimated to contain 10 times more cells and 100 times more genes than host, and includes a wide range of organisms, such as bacteria, fungi, protozoa, archaea and viruses. This ecosystem contains numerous native species that permanently colonize gastrointestinal tract, namely autochthonous and several other transient microorganisms, namely allochthonous species. Firmicutes (Gram-positive) and Bacteroidetes (Gram-negative) are the cardinal phyla in gut microbiota, accounting for roughly 60–80% and 20–40% of the total bacterial load. It is estimated that two microbial phyla take up 90% of the total microbiota. Additionally, Gram-positive bacteria, such as Actinobacteria, and Gram-negative bacteria, such as Verrucomicrobia, Proteobacteria, Cyanobacteria and Fusobacteria exist in relative less abundance in adult gastrointestinal tract [39]. Gut microbial community produces a natural defense barrier and influences physiology, immune function, metabolism and nutrition. A variety of clinical and experimental evidence has indicated that microbial environment has played a key role in health and disease, such as cancers, cardiovascular disease, obesity, diabetic mellitus, hypertension, CKD and non-alcoholic fatty liver disease [40–52].
Gut microbiota in CKD patients
CRF and ESRD patients
CRF and ESRD belong to the advanced stages of CKD. CKD leads to the dysbiosis of gut microbiota that destroys intestinal mucosal barrier, allowing live bacteria or their metabolites to enter bloodstream. Leakage and translocation of their metabolites cross gut barrier to the circulation and trigger oxidative stress and inflammation that finally lead to local tissue and vascular dysfunction (Figure 1). Liu and his colleague [53] demonstrated a remarkable difference of gut microbiota between patients with CKD and healthy controls. After adjusting for confounders, lower Chao1 and Shannon indices were observed according to binary logistic regression predicted CKD prevalence. The increased relative abundances of Actinobacteria, Bifidobacterium, Alistipes and Bifidobacterium longum, activation of metabolic signaling pathways such as bacterial toxin and chloroalkane and its degradation might predict CKD [53]. Of note, the depletion of Bifidobacterium and Actinobacteria and decrease in chloroalkene and chloroalkane decomposition indicated high levels of free immunoglobulin light chains [53]. Chen and his colleague [54] demonstrated that Shannon entropy and Simpson indices showed no obvious difference in α-diversity between healthy controls and patients with stage 1–5 CKD. The weighted UniFrac or Bray–Curtis dissimilarity principal coordinate analysis showed that unique bacterial population aggregates were found in the faeces of patients with CKD at distinct stages compared with healthy group, which indicated the differences in composition rather than richness or numerical abundance between healthy controls and patients with CKD [54]. The relative abundance of Klebsiella pneumonia and the relative levels of S-adenosylhomocysteine in the faeces of patients with CKD were demonstrated to show a gradual increase with CKD severity [54]. Further results revealed increased Klebsiella pneumonia was positively correlated with increased S-adenosylhomocysteine in the faeces [54]. Moreover, Lv and his colleague [55] demonstrated that Enterobacteriaceae and Enterococcus showed high relative abundances in patients with CRF compared with healthy controls, while Lactobacillus, Bifidobacterium, Clostridium and Bacteroides showed lower relative abundances. Furthermore, Simpson index was decreased in patients with CRF compared with healthy controls, while Chao1 and Shannon indexes were increased in patients with CRF compared with healthy controls [55]. Flow cytometry analysis showed that the levels of CD3+, CD4+, CD8+ and CD4+/CD8+ were significantly decreased in patients with CRF compared with healthy controls, while the levels of CD14+, CD19+, and CD16+/CD56+ were significantly increased in patients with CRF compared with healthy controls [55]. Chao index was negatively correlated with levels of CD3+, CD4+ and CD8+ in patients with CRF. Chao index was positively correlated with levels of CD14+ and CD16+/CD56+ in patients with CRF [55]. These findings indicated that the dysbiosis of gut microbiota in CKD patients leads to the activation of inflammation and imbalance of immune function.
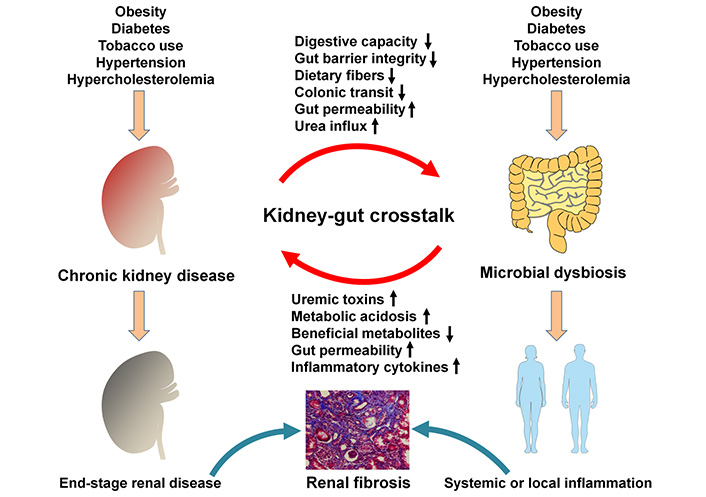
Mechanisms involved in the relationship between gut microbial dysbiosis and CKD. CKD led to the dysbiosis of gut microbiota that destroys intestinal mucosal barrier, allowing live bacteria or their metabolites to enter the bloodstream. Leakage and translocation of their metabolites across gut barrier to the circulation can trigger oxidative stress and inflammation that finally leads to local tissue and vascular dysfunction
The latest study demonstrated that the marked alterations of the microbial diversity, taxonomic composition and functional potential of the microbiome were observed in the faecal samples of patients with ESRD compared with healthy controls [56]. Some increased gut microbial species in patients with ESRD were correlated with clinical indexes and encoded functions that were involved in toxin and secondary bile acids synthesis, which were associations between altered microbial functions and levels of metabolites in serum or faeces [56]. Treatment with microbiota from patients could blunt renal injury in the germ-free mice, while for antibiotic-treated rats it led to high levels of uraemic toxins in serum and exacerbated renal fibrosis compared with healthy controls [56]. Two species including Eggerthellalenta and Fusobacterium nucleatum could increase uraemic toxins production and promote renal damage in the adenine-induced CKD rats [56]. A probiotic Bifidobacterium animalis could reduce relative abundance of these species and decrease levels of toxins and disease severity in rats [56]. Taken together, the dysbiosis of gut microbiota has an important effect on patients with CRF or ESRD.
CKD with different causes and changes
IgA nephropathy
IgA nephropathy is usually considered to be an immune complex-induced or polymerized IgA1-induced glomerulonephritis [57–60]. Zhong et al. [61] reported that although α-diversity exhibited no significant differences in taxon richness and evenness, there was a clear separation in the composition of gut microbiota between patients with IgA nephropathy and healthy controls, indicating that the diversity of gut microbiota was altered in patients with IgA nephropathy. The relative abundances of Bacteroides and Escherichia-Shigella were abundant in patients with IgA nephropathy compared with healthy controls, while the relative abundances of Bifidobacterium and Blautia spp. were decreased [61]. Higher relative abundance of Escherichia-Shigella and lower relative abundance of Bifidobacterium spp. were observed in patients with IgA nephropathy with high urine red blood cell count and proteinuria levels [61]. The relative abundance of Prevotella 7 was negatively correlated with the levels of the galactose-deficient IgA1, lipopolysaccharide binding protein, soluble cluster of differentiation 14, intercellular adhesion molecule-1 and tumour necrosis factor α, whereas the relative abundance of Bifidobacterium spp. showed a significant inverse correlation with lipopolysaccharide binding protein and galactose-deficient IgA1. Moreover, the relative abundance of Escherichia-Shigella was negatively correlated with Prevotella 7 [61]. Collectively, the dysbiosis of gut microbiota has an important effect on patients with IgA nephropathy.
DKD
DKD is the most common microvascular complication and cardinal cause of ESRD [62]. He and his colleague [63] demonstrated that patients with DKD showed an increase in Proteobacteria at the phylum levels, Shigella, Selenomonadales, Bilophila, Neosynechococcus and Acidaminococcus at the genus levels, and Escherichia coli, Megasphaeraelsdenii, Bacteroides plebeius and Bilophilawadsworthia at the species levels. The relative abundances of Syntrophaceticusschinkii and Citrobacter farmeri were positively correlated with urinary albumin/creatinine ratio in patients with DKD [63]. Functional analysis revealed the dysregulation of lipopolysaccharide biosynthesis and carbohydrate metabolism in the gut microbiome of patients with DKD [63]. Recently, the study showed that faecal microbial richness and diversity in patients with DKD were decreased compared with healthy controls [64]. The composition of gut microbiota in the faeces of the patients with stage 3 DKD was at the middle levels between healthy controls and patients with stage 4 [64]. The gender and body mass index had slight influence on the gut microbiota profile but the main difference still came from the disease [64]. The latest 16S ribosomal deoxyribonucleic acid gene sequencing analysis showed dysbiosis of gut microbial was demonstrated in patients with diabetic mellitus and DKD, and relative abundance of some short chain fatty acids-producing bacteria was lowered in patients with DKD [65]. Butyrate levels in serum were reduced in patients with DKD compared with patients with diabetic mellitus and healthy controls. Butyrate levels in serum were positively correlated with estimated glomerular filtration rate [65]. Treatment with butyrate could elevate butyrate levels in serum and faeces and inhibit glomerular area enlargement as well as intrarenal fibronectin and collagen IV expressions in the rats with diabetic mellitus [65]. Treatment with butyrate could increase LC3 messenger ribonucleic acid expression and LC3BII/I ratio in renal tissue of diabetic mellitus rats. Intrarenal higher phospho-adenosine monophosphate-activated protein kinase (p-AMPK)/AMPK ratio and lower phospho-mammalian target of rapamycin (p-mTOR)/mTOR ratio were demonstrated in diabetic mellitus rats with sodium butyrate compared with diabetic mellitus rats [65].
Metagenomic analysis indicated that α-diversity and β-diversity were not remarkably different between diabetic and non-diabetic participants [66]. There were no differences in between-sample diversity, which was consistent with the results of an early study that reported no differences in diversity from ESRD patients [67], and results further indicated that diversity did not differ in the patients with CKD in early-stage CKD 3a compared with the non-CKD patients [66]. Taxonomic analysis showed that butyrate-producing Roseburiainulinivorans, Ruminococcus torques and Ruminococcuslactaris were high relative abundant in the patients with non-CKD, whereas Bacteroides caccae and Bacteroides coprocora were high relative abundant in the patients with non-diabetic CKD [66]. Therefore, the dysbiosis of gut microbiota has a critical effect on DKD. Increased evidence has demonstrated that a number of natural products could improve the dysbiosis of gut microbiota in DKD [68–72].
MN
MN is the most common cause of non-inflammatory autoimmune antibody-related nephrotic syndrome in adults [73–76]. Zhang et al. [77] showed that patients with idiopathic MN (IMN) exhibited an altered α-diversity and β-diversity compared to patients with CKD and healthy controls. IMN patients exhibited increased relative abundances of Fusobacteria and Proteobacteria but reduced relative abundance of Firmicutes compared with healthy controls. At genus level, the relative abundances of Megasphaera, Akkermansia, Megamonas, and butyrate-producing bacteria Lachnospira, Fusobacterium and Roseburia were abundant in healthy controls compared with patients with CKD and IMN [77]. Compared with healthy controls, the decreases in propionate and butyrate were observed in faeces of IMN patients. The abundance of Parabacteroides was increased in patients with CKD and IMN compared with healthy controls. In addition, the relative abundances of Oscillospira and Ruminococcus were abundant in patients with CKD compared with patients with IMN and healthy controls. At the genus level, ten bacterial taxa were abundant in healthy controls. The relative abundances of Providencia and Myroides were abundant in patients with IMN [77]. Dong et al. [38] showed that the relative abundances of Streptococcus, Escherichia-Shigella and Peptostreptococcaceae_incertae_sedis were abundant, while the relative abundances of Lachnospira, Lachnospiraceae_unclassified, Clostridium_sensu_stricto_1 and Veillonella were decreased in patients with kidney biopsy-proven MN. The relative abundances of Megasphaera and Bilophila were abundant, whereas the relative abundances of Megamonas, Veillonella, Klebsiella and Streptococcus were decreased in patients with kidney biopsy-proven IgA nephropathy (IgAN) than in those patients with MN [38]. A negative correlation was revealed between the relative abundance of Escherichia-Shigella and proteinuria levels, the relative abundances of Klebsiella and Bacteroides presented a positive correlation with MN stage [38]. Additionally, Yu et al. [78] demonstrated MN patients showed more severe dysbiosis of gut microbiota than patients with DKD. A higher number of pathogens in MN were major contributors to microbiome changes in MN. Metabolic pathway analysis exhibited remarkably elevated pentose/glucuronate interconversion and membrane transport in association with adenosine triphosphate-binding cassette transporters and phosphotransferase system in MN [78]. Treatment with faecal microbiota transplantation could treat patient with MN and chronic diarrhea, which could attenuate symptoms and enhance kidney function [79].
Antineutrophil cytoplasmic antibody-associated vasculitis with kidney injury
Increasing evidence suggested that gut microbiota played critical roles in developing autoimmune diseases. Antineutrophil cytoplasmic autoantibody-associated vasculitis is characterized by necrotizing injury to small-vessel vasculitis and mostly occurs in kidney or lung tissues [80]. The latest studies demonstrated that decreased α-diversity indexes were observed in antineutrophil cytoplasmic antibody-associated vasculitis patients with kidney injury compared with healthy controls, while significant differences of β-diversity indexes were observed in antineutrophil cytoplasmic antibody-associated vasculitis patients with kidney injury, patients with lupus nephritis and healthy controls [81]. The relative abundances of Deltaproteobacteria, unclassified_o_Bacteroidales, Prevotellaceae, Desulfovibrionaceae Paraprevotella, and Lachnospiraceae_NK4A136_group were negatively correlated with serum creatinine levels and the relative abundances of Deltaproteobacteria, unclassified_o_Bacteroidales, Lachnospiraceae_NK4A136_group, Desulfovibrionaceae and Paraprevotella had a positive correlation with estimated glomerular filtration rate in patients with antineutrophil cytoplasmic antibody-associated vasculitis [81].
Intervention strategies through regulating gut microbiota in CKD patients
Increasing publications have demonstrated that CKD could be ameliorated by improving the dysbiosis of gut microbiota via some intervention strategies [82, 83]. Mo and his colleague [84] demonstrated that treatment with α-Ketoacid could decrease the levels of serum creatinine, blood urea nitrogen and 24 h proteinuria, as well as retarded interstitial fibrosis and glomerulosclerosis in the adenine-induced CKD rats. Moreover, treatment with α-ketoacid might improve intestinal cell epithelial barrier and increase relative abundances of Blautia, Methanobrevibacter, Anaerositipes and Akkermansia while reducing the relative abundance of Anaerovorax and Coprococcus_3 at genus level [84]. This study also indicated that treatment with α-ketoacid might reduce the levels of indoxyl sulfate, betaine, cholesterol and choline. Spearman’s correlation analysis exhibited that relative abundance of Coprococcus_3 was positively correlated with the levels of trimethylamine N-oxide, indoxyl sulfate, cholic acid, deoxycholic acid and betaine, in serum [84]. Merino-Ribas and his colleague [85] demonstrated that a 5-month treatment with either calcium acetate or sucroferric oxyhydroxide did not affect baseline diversity and bacterial composition in patients with hemodialysis, also revealed high-variability profiles of gut microbiome among patients with hemodialysis. Moreover, a randomized controlled trial indicated that treatment with β-glucan prebiotics significantly changed intestinal-derived uremic toxin levels and favorably affected gut microbiome [86].
Mounting studies have highlighted that the natural products have been long demonstrated to protect against organ fibrosis in a variety of refractory diseases including renal disease [87–93]. Increasing publications have demonstrated that natural products could attenuate renal fibrosis via improving the dysbiosis of gut microbiota [68]. Pivari and his colleague [94] demonstrated that treatment with curcumin could lower plasma proinflammatory mediators including C-C motif chemokine ligand 2, interferon-γ and interleukin-4 as well as lipid peroxidation in patients with CKD. Treatment with curcumin for patients with CKD could lower the relative abundance of Escherichia-Shigella, while increasing the relative abundance of Lachnoclostridium after six months. Meanwhile, significantly increased relative abundance of Lactobacillaceae spp. was observed in the last three months by curcumin supplementation [94]. Rhubarb is one of the most important natural products and is usually used for treating renal diseases [95–98]. Treatment with rhubarb enema could decrease the levels of trimethylamine N-oxide and trimethylamine in serum, suppress the expression of interleukin-6, tumour necrosis factor α and interferon-γ and mitigate tubular atrophy, inflammatory cell infiltration and tubulointerstitial fibrosis in the 5/6 nephrectomized rats [82]. Moreover, treatment with rhubarb enema could enhance the relative abundance of symbiotic bacteria and probiotics, while lower the relative abundance of the pathogens. Spearman’s correlation analysis showed that romboutsia and lachnospiraceae were positively correlated with serum trimethylamine N-oxide levels [82]. In addition, Peron and his colleague [99] demonstrated that polyphenol-rich-diet could significantly increase serum indole 3-propionic acid level in individuals with normal renal function, but not in individuals with injured renal function, while other gut microbiota-tryptophan metabolites were not affected. Comparison of baseline gut microbiota composition showed shifts in Bacteroidales and higher abundance of Clostridiales in individuals with normal renal function. During the trial, variations of indole 3-propionic acid are related to alternations in C-reactive protein level and gut microbiota, particularly with the Clostridiales and Enterobacteriales [99].
Antibiotics have an important effect on the gut microbiota. Nazzal et al. [100] showed that vancomycin could lead to the reduced levels of seven colon-derived uremia solutes in the plasma of patients with ESRD. Vancomycin showed a significant effect on the microbiota structure with a reduced α-diversity and altered β-diversity [100]. Multiple taxa could reduce with vancomycin including Bacteroides and Clostridium at the genus levels, while microbiome could recover after stopping vancomycin. However, recovery in the uremia solutes was highly variable between subjects [100].
Renal replacement therapies such as hemodialysis, peritoneal dialysis and renal transplantation are the mainstay therapies for patient with ESRD [101–110]. The dysbiosis of gut microbiota was involved in these renal replacement therapies [111–114]. Hu and his colleague [115] demonstrated that different dialysis treatments affected relative abundance of butyric acid-producing bacteria including Rosella and Phascolarctobacterium and conditional pathogens Escherichia spp. in patients with ESRD. Furthermore, butyric acid-producing bacteria presented a positive correlation with protein–energy wasting and exhibited a negative correlation with Escherichia [115]. Escherichia coli peritonitis is a serious complication of peritoneal dialysis. Other study reported that faecal community diversity exhibited a significant alternation in patients with Escherichia coli peritonitis, where the relative abundances of Synergistetes and Bacteroidetes were significantly increased, while Bacilli and Lactobacillus were significantly decreased at different levels compared with normal group, which were associated with metabolic pathways was observed in patients with Escherichia coli peritonitis [116]. In addition, Ma and his colleagues [117] revealed that gut microbial diversity was significantly decreased and the relative abundance of many bacteria including Enterococcus and Streptococcus were significantly changed after transplantation, which were accompanied by changed levels of many amino acids and peptides in serum and faeces. Furthermore, the relative abundance of Enterococcus was correlated with renal functions and the levels of metabolites reflecting renal injury. Collectively, targeting the dysbiosis of gut microbiota could be considered as an important regimen for CKD intervention.
Gut microbiota-associated metabolites in patients with CKD
Metabolism regulation is one of three philological functions. The dysbiosis of gut microbiota leads to the disorder of metabolites. The latest study revealed that relative abundances of thirteen microbial species and levels of six serum metabolites were significantly changed across early to advanced stages of CKD patients [118]. Decreased relative abundance of Prevotella sp. 885 was related to urea excretion, while the levels of decreased caproic acid and increased p-cresyl sulfate were positively and negatively correlated with glomerular filtration rate, respectively [118]. Microbial genes related to secondary bile acid biosynthesis were enriched at the early stage, while pathway modules associated with lipopolysaccharide biosynthesis and lipid metabolism were enriched in microbiome at the advanced stage of CKD [118]. The results further showed identified biomarkers from metagenomics and metabolomics to discriminate cases of different severities from controls, among which Bacteroides eggerthii was of important value in early diagnosis of CKD patients [118]. Therefore, targeting the dysbiosis of gut microbiota could be considered as an alternative therapeutic strategy for CKD treatment (Figure 1).
Several studies have demonstrated that the dysbiosis of gut microbiota was associated with the dysregulation of metabolites in patients with IgA nephropathy. Wu et al. [119] demonstrated that Enterococcus and Streptococcus showed high relative abundances in patients with IgA nephropathy, whereas Bacteroidetes and Bacteroides showed low relative abundances, which led to dysregulated metabolites, especially free amino acid, polyunsaturated fatty acids, and oligopeptides, and activated phenylalanine metabolism. PE (17:0), trimethoprim, bilirubin, stearamide, phenylalanine and cis-9,10-epoxystearic acid were associated with metabolic pathway and networks in gut and serum. Of note, 5-hydroxyeicosatetraenoic acid and 5-hydroxy-6E,8Z,11Z-eicosatrienoic acid were related to glomerulosclerosis classification [119]. The latest study demonstrated that the levels of intestinal fatty acids, mostly unsaturated fatty acids, were significantly decreased in patients with IgA nephropathy [120]. Disorders of linoleic acid, arachidonic acid, anti-/pro-inflammatory fatty acid metabolites, and intestinal aryl hydrocarbon receptor signaling deficiency might indicate intestinal mucosal barrier injury in patients with IgA nephropathy [120]. Moreover, high levels of galactose deficient IgA1, interleukin-22 and tumour necrosis factor α while lower level of 3-indolepropionic acid were related to kynurenine metabolic pathway from tryptophan metabolism, indicating tryptophan metabolites including 3-indolepropionic acid, kynurenine and indoleacrylic acid had synergistic effects on modulating immuno-inflammatory responses in patients with IgA nephropathy [120]. In addition, Chai and his colleague [121] demonstrated that α-diversity and β-diversity were remarkably different between patients with IgA nephropathy and healthy controls, which were accompanied by decreased levels of caproic acid, acetic acid, propionic acid, butyric acid and isobutyric acid in faeces of patients with IgA nephropathy compared to healthy controls. Further, the relative concentrations of butyric acid and isobutyric acid in faeces were negatively correlated with urea acid concentrations, while faecal butyric acid concentrations were negatively correlated with blood urea nitrogen concentrations; faecal caproic acid concentrations exhibited negative correlation with 24 h proteinuria concentrations [121]. Acetic acid concentrations in faeces were positively associated with Clostridiales and Eubacterium_coprostanoligenes. Butyric acid concentrations in faeces were positively associated with the relative abundance of Alistipes. Their relative abundances were significantly reduced in patients with IgA nephropathy compared with healthy controls [121].
A number of evidence has showed the dysregulation of metabolites in CKD patients. Several studies have indicated that the dysregulation of metabolites was involved in the dysbiosis of gut microbiota in patients with DKD. Zhang and his colleague [122] demonstrated that eleven bacteria and 239 serum metabolites were found between DKD patients with ESRD and DKD patients without ESRD. Serum 192 metabolites that were related to eleven bacteria were high enriched in six metabolic pathways. Of note, tryptophan and phenylalanine metabolic pathways were related to the progression of DKD. Hippuric acid, 3-phenyllactic acid, dihydro-3-coumaric acid and 3-hydroxy-cinnamate in the phenylalanine metabolic pathway and indole-3-acetic acid in the tryptophan metabolic pathway were positively correlated with the progression of DKD, while tryptophan presented a negative correlation with the progression of DKD [122]. The relative abundances of norank Peptococcaceae and Abiotrophia were positively correlated with enhancing renal function and hippuric acid [122]. The relative abundances of Lachnospiraceae_NC2004_group were negatively correlated with improving renal function as well as 3-phenyllactic acid and indole-3-acetic acid in serum [122].
Summary and perspectives
The dysbiosis of gut microbiota plays an important role in the pathogenesis of CKD, which significantly affects the number, composition and functions of gut microbiota in patients with CKD. Proteins and nitrogenous endogenous compounds are metabolized by gut microbiota, producing the metabolites especially harmful microbial metabolites uremic toxins such as trimethylamine-N-oxide, indoxyl sulfate and p-cresyl sulfate in patients with CKD. The levels of these metabolites correlate with disease progression. Increased uremia toxins leaked into the bloodstream through the intestinal epithelial cell barrier damage that triggered endotoxemia and mediated systemic or local inflammation, which aggravated declining renal function in patients with CKD (Figure 1). The complex interaction between gut microbiota, intestinal epithelial cell barrier, bloodstream microbial-derived metabolites and kidney tissues might orchestrate subclinical changes in the pathogenesis of CKD and contribute to CKD progression. A number of clinical trials have indicated that targeting gut microbiota regulation via intervention approaches, such as prebiotics, probiotics and synbiotics supplementation as well as dietary guidance could ameliorate CKD and inhibit progressive renal fibrosis. Further studies should be conducted by systems biology including metagenomics-based gut microbiota analysis to identify gut microbiota community, metabolic signaling pathways and microbial genes, metabolomics-based blood metabolite identification and illumination of metabolite-mediated underlying molecular mechanism in host organs. Therefore, the modulation of gut microbiota could be considered as a promising therapy in complement to traditional drugs and dialysis treatment for patients with CKD. However, the investigation should be performed for identifying gut microbiota at the early disease stages to provide references for dietary intervention for CKD patients. These future studies may provide a clue for disease etiology, metabolic pathways and alternative treatment for CKD.
Abbreviations
CKD: | chronic kidney disease |
CRF: | chronic renal failure |
DKD: | diabetic kidney disease |
ESRD: | end-stage renal disease |
IgA: | immunoglobulin A |
IMN: | idiopathic membranous nephropathy |
MN: | membranous nephropathy |
Declarations
Author contributions
The author contributed solely to the work.
Conflicts of interest
The author declares that he has no conflicts of interest.
Ethical approval
Not applicable.
Consent to participate
Not applicable.
Consent to publication
Not applicable.
Availability of data and materials
Not applicable.
Funding
This study was supported by the National Natural Science Foundation of China (Nos. 82074002, 818729858). The funders had no role in study design, data collection and analysis, decision to publish, or preparation of the manuscript.
Copyright
© The Author(s) 2022.