Abstract
The brain and gut are connected both physically and biochemically. The gut-brain axis includes the central nervous system, neuroendocrine and neuroimmune systems, the enteric nervous system and vagus nerve, and the gut microbiome. It can influence brain function and even behavior, suggesting that dietary interventions may help enhance and protect mental health and cognitive performance. This review focuses on the role of the microbiome and its metabolites in sleep regulation, neurodegenerative disorders, mechanisms of stress, and mood. It also provides examples of nutritional interventions which can restore healthy gut microbiota and aid with risk reduction and management of many disorders related to mental and cognitive health. Evidence suggests a shift in the gut microbiota towards a balanced composition could be a target to maintain brain health, reduce stress and improve quality of life.
Keywords
Gut-brain axis, microbiome, Alzheimer’s disease, Parkinson’s disease, biotics, sleep, stress, blood-brain barrierIntroduction
Mental health and neurodegenerative disorders, like Alzheimer’s disease (AD) and Parkinson’s disease (PD), are among the most common, costly, and debilitating health conditions worldwide [1]. Poor sleep and high stress are prevalent in the general population and may be causal factors in the onset of these issues [2–4]. Available pharmaceutical treatments are often slow or inadequate, leading to an interest in preventative, adjunctive, and alternative therapeutic approaches. The gut has been identified as a critical avenue for investigation, given structural gut-brain connections and the burgeoning literature linking gut microbiome disruptions to central nervous system (CNS) disorders. Anxiety, depression, and dementia are linked to gastrointestinal (GI) microbiome disturbances [5, 6]. Increasingly, preclinical studies support the role of the gut in shaping behavioral and cognitive outcomes [7–9]. The evidence points to nutrition as a feasible target to improve mental wellbeing and maintain healthy cognitive function.
The gut stores neurotransmitters, such as serotonin, a signaling molecule and key hormone that stabilizes mood and feelings of well-being [10] and dopamine (up to half is stored in the gut) which is essential in processes linked to motivation, memory, and attention [11]. The gut-brain axis (GBA) refers to the bi-directional communication between brain and gut, which is often significantly impacted by microbiome [12].
Interestingly, a microbiota-gut-immune-glia (MGIG) axis was described for depressive disorders. It is likely to be activated by gut-associated lymphoid tissue upon changes to gut barrier lining resulting in the aberrant cross-talk between glial-neurons, low-grade inflammation, and depression (reviewed by Rudzki and Maes [13]).
Additionally, cross talk between gut microbiota and CNS has been implicated in several other mental disorders ranging from autism [14], attention-deficit/hyperactivity disorder (ADHD) [15] to alcoholism [14–16]. A three-way interplay with microbiome intimately associated with sleep and cognition has been described [17].
This review focuses on crucial evidence linking the microbiome to stress, sleep quality, and cognitive decline. Recent intervention studies demonstrating how the consumption of nutritional ingredients may offer benefits for associated health conditions are also highlighted.
The GBA
The role of microbiota
The human microbiota consists of trillions of microbial cells in each person [18]. In more technical terms, microbiota refers to all microorganisms found in a selected environment (e.g., gut). However, another widely used term is microbiome which describes the collection of genomes from all the microorganisms. The gut microbiome is vital in the functioning of the nervous system. Gut microbiota produce neuroactive compounds, such as neurotransmitters, amino acids, and metabolites, which affect the brain or indirectly act through the enteric nervous system (ENS). The ENS is an autonomous part of the nervous system which controls mucosal secretions, and motor and endocrine functions [19]. It connects the gut microbiota, hypothalamic-pituitary-adrenal (HPA) axis, and vagus nerve transmitting information from the gut to the brain [19]. Bacterial fermentation products, such as butyrate [20] and gamma-aminobutyric acid (GABA) [21], have neuroprotective effects. For example, GABA-producing Lactobacillus species (Lacticaseibacillus according to new nomenclature) influence the neural network of the host [22]. Germ-free mice (born and raised in sterile conditions) had higher tryptophan (essential in serotonin production) compared to control mice but almost no serotonin [23]. The microbiota-depleted mice were also deficient in vitamin B6 metabolites, which are the molecules that accelerate the production of serotonin and dopamine [23]. The protective effects of butyrate include its capacity to upregulate the melatonergic (melatonin-producing) pathway [24]. At the same time, melatonin also upregulates gut microbiome-derived butyrate [25]. Thus the microbiota plays a vital role in the production of neurotransmitters from the diet through various pathways.
The GI metabolism is closely connected to brain function via the circulatory system and vagus nerve, creating GBA. Ninety percent of the neural connection in GBA is through the vagus afferent nerve fibers, which travel from the gut to the brain [26]. Although conceptual borders between hormones, neurotransmitters, and cytokines are blurred (i.e., certain compounds can act as both hormone and neurotransmitter, such as adrenaline), GBA components are well-defined. They can work separately or in unison (Figure 1). Also, changes on either side of GBA communication may affect the other. For example, gut microbiota communicates with the brain through cytokines released by mucosal immune cells, serotonin produced by endocrine cells, and the vagus nerve. In contrast, efferent signals (away from the brain) related to stress, such as sympathetic neurotransmitters (e.g., GABA), influence gut microbiome and secretion [27]. For example, increased stress can cause changes in gut microbiome (e.g., lower levels of lactic acid bacteria) [28]. In contrast, modulation of the microbiome with probiotics or diet can help elevate mood and reduce anxiety [29].
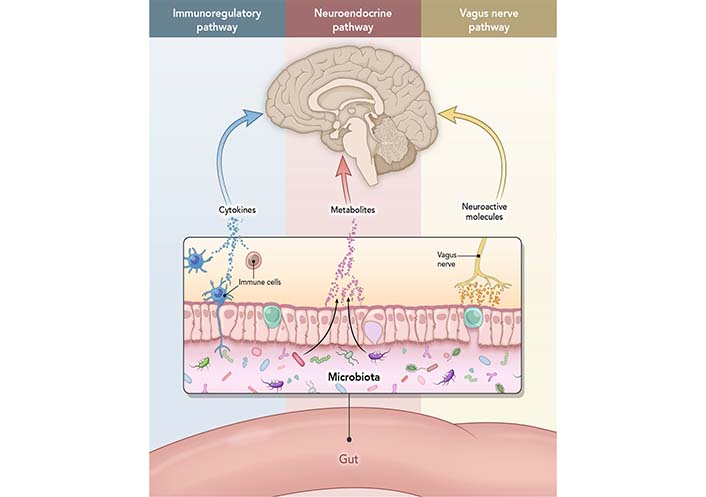
The interactions that occur at the GI mucosa level, and via neural, endocrine, or immune activity. Immunoregulatory pathway: a finely balanced system where microbiome interacts with immune cells to train and mature the components of the immune system while the immune system allows vital microbiome and host cell symbiosis. Neuroendocrine pathway: microbiome affects the HPA axis and the CNS via secretion of neurotransmitters, such as GABA, serotonin, melatonin, histamine, and acetylcholine. Vagus nerve pathway: vagus nerve establishes the connection between the brain and the GI tract and sends information about its state to the brain via afferent fibers
Along with the increase in age, there is a shift in the microbiome. The microbiome changes are associated with impairment in sleep, development of anxiety, and depression disorders [30]. Aging also reshapes the gut microbiome structure with a high abundance of pro-inflammatory bacteria (e.g., Pseudomonadota and previous nomenclature Proteobacteria) and fewer anti-inflammatory bacteria (e.g., Lactocaseibacillus or Bifidobacterium species), causing local systemic inflammation and permeability of the GI tract [31]. This further impairs the blood-brain barrier (BBB) function, leading to neuroinflammation and neuronal damage.
Crossing the BBB
The BBB is composed of multiple cell types and is the protective system that restricts the entry of blood-derived molecules and pathogens into the CNS (Figure 2), which would be harmful to the brain. The BBB comprises endothelial cells (which regulate inflammatory reactions), glia (cells with various supporting functions in the brain, e.g., astrocytes), neurons, and extracellular matrix components that maintain the CNS. Neurons, glial cells, and immune cells share intercellular signals, while some signals can cross the BBB in both directions. Preclinical data demonstrated the role GBA plays in maintaining BBB integrity. For example, in mice deprived of microbiome (i.e., germ-free), the BBB is more permeable, with decreased tight junction protein expression and disorganized tight junctions [32]. Microbiota products, such as short-chain fatty acids (SCFAs), restore BBB stability [33]. Sleep loss and severe stress impact the function of BBB by disrupting the interaction of pericytes and endothelial cells [34, 35]. Sun and colleagues [36] demonstrated that learning and memory were impaired in rats with increased BBB permeability. Those authors described a strong correlation between sleep deprivation and BBB, suggesting a molecular basis for those changes.
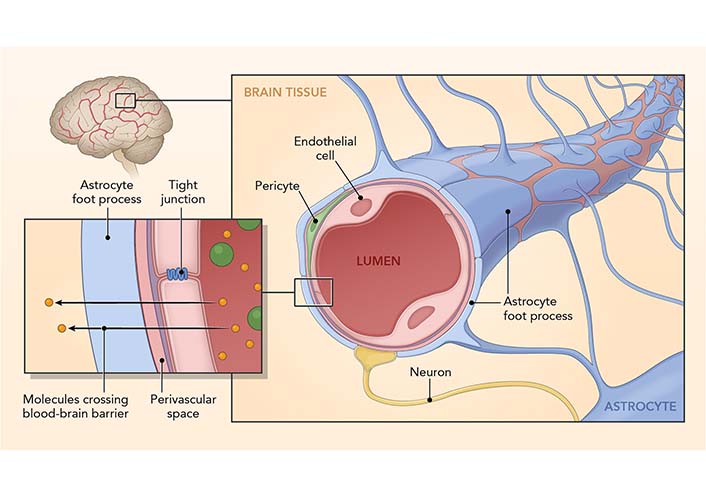
The BBB. The BBB is a structural and functional impediment to undesirable components or microorganisms that would harm the brain. It comprises endothelial cells (which regulate inflammatory reactions), astrocytes (with various supporting functions in the brain), neurons, and extracellular matrix components that function together in the maintenance of CNS
Sleep
An essential physiological process impacted by GBA is sleep. Sleep is regulated by 1) sleep pressure, the accumulation and binding of adenosine (nucleoside presents in all human cells) to its receptor and further signaling the need for sleep, and 2) circadian rhythm. Circadian rhythm is a biological process that follows an about 24-hour cycle based on the body’s internal clock, e.g., the sleep-wake cycle. Aging involves changes to sleep patterns, like an increase in the time it takes to fall asleep (sleep latency) and an overall decline in rapid eye movement (REM) sleep [37]. With age, people tend to have more difficulty falling asleep (sleep latency increases) and more trouble staying asleep (shallow and fragmented sleep). Sleep is critical in neural biological regulation by allowing the elimination of metabolites via BBB (reviewed by Cuddapah et al. [38]).
Factors that alter sleep pressure can also cause sleep disturbances [39]. Dementia, impaired immunity, and stress are correlated with disrupted sleep, leading to cognitive decline (reviewed by Hughes et al. [40]). There is an intricate connection between sleep and mood states, and the relationship between sleep quality, and anxiety and depression has been described [41–45]. Aging is one of the risk factors for insomnia, coupled with fragmented sleep [46] and gut dysbiosis [47]; there seems to be a confluence of risk factors that lead to insomnia and subsequent many mental issues seen in old age [30].
Microbiome structure and diversity also exhibit diurnal rhythms [48]. In a preclinical study, depletion of microbes eliminated serotonin in the gut, affecting the sleep-wake cycles [23]. Stress exposure also disrupts sleep (circadian misalignment) and causes gut microbial imbalance (dysbiosis). Preclinical studies show that microbiota has a profound effect on sleep. Germ-free mice had more REM and non-REM sleep (compared to animals colonized with bacteria) during the time when mice are typically active. The same study also showed that the absence of bacteria caused less daytime non-REM sleep, in contrast to normal behavior when mice typically sleep [23]. Those sleep disturbances could be related to low levels of serotonin, a key neurotransmitter associated with the GBA [41]. In their recent review, Han et al. [49], conclude that abnormal sleep patterns and duration affect the composition and diversity of the microbiota.
Several preclinical and clinical studies have demonstrated a link between sleep and cognitive disorders such as AD and PD [17] (further reviewed here [50]).
Neurodegenerative disorders
AD
AD is a neurodegenerative CNS disorder accounting for 60–80% of all dementias [51]. Clinical features include a progressive decline in cognitive function (including a severe memory impairment) that adversely impacts daily functioning and quality of life. The hallmark pathology of AD is characterized by the extracellular deposition of beta-amyloid (a small piece of a larger protein) as neuritic plaques and intracellular hyperphosphorylated tau protein as tangles [52]. The BBB functioning is severely affected in AD patients, as shown by magnetic resonance imaging (MRI) studies [53].
There is evidence of an aberrant gut microbiome resulting in inflammation, primarily through microglia, which impacts synapse and pathophysiology of AD (reviewed by Bairamian [54]). Studies increasingly suggest that microbes likely contribute to the progressive neurodegeneration observed in AD [55]. The microbiome of elderly patients with AD shows a lower proportion of bacteria producing butyrate, which promotes anti-inflammatory activity, and more bacterial taxa known to cause pro- inflammatory states [56, 57]. Cattaneo et al. [57] observed a shift towards pro-inflammatory and lower anti-inflammatory bacteria (e.g., Bacillus fragilis, Eubacterium rectale, Faecalibacterium prausnitzii, and Bacteroides fragilis) in amyloid-positive patients compared to healthy subjects. Interestingly, patients with depression, bipolar disorder, and anxiety also tend to have fewer butyrate-producing bacteria and more pro-inflammatory bacteria [58].
In the mouse AD model, brain infection with Salmonella typhimurium promoted beta-amyloid plaque formation close to Salmonella attachment sites long before the natural occurrence of plaques in uninfected mice [59]. Bacteria such as Bacillus subtilis secrete large amounts of lipopolysaccharides (LPS, an endotoxin that can induce anxiety-like behavior) and amyloid proteins that cross the BBB [60]. Changes in gut microbial composition enhance the release of LPS and amyloid proteins, which likely contribute to AD pathogenesis, especially during aging when both the GI tract epithelium and BBB are more permeable. LPS and amyloid proteins directly pass through a compromised GI tract or BBB and trigger the passage of cytokines which likely drive AD [59].
PD
In PD, neurons producing dopamine in the mid-brain (substantia nigra and striatum) degenerate, resulting in the deficiency of this critical neurotransmitter [61] and the buildup of alpha-synuclein aggregates in the remaining neurons. Metabolites produced by bacteria trigger an immune response inducing inflammation and development of PD [62]. With lower levels of the intestinal hormone ghrelin, researchers observed increased Lactobacillaceae and decreased Prevotellaceae in the gut [61]. The microbiome of PD patients had fewer butyrate-producing bacteria and more pro-inflammatory Proteobacteria, which may trigger misfolding of alpha-synuclein and the development of PD [63].
Nutritional interventions—modulating the gut microbiome
With the demonstrated link between the microbiome and both AD and PD, modulating the gut microbiome with diet or biotics could lead to promising prophylactics and solutions. A preclinical study with fecal microbiota transfer therapy in AD mice demonstrated changes in microbiome which ameliorated amyloidosis, tau pathology, and cognitive impairment [64]. In another study, probiotic Bifidobacterium breve A1 increased the plasma acetate levels in mice; this probiotic also suppressed inflammation-related genes induced by beta-amyloid accumulation in the brain [65]. In mouse AD models, mice fed fructooligosaccharide (FOS) were resilient to induced cognitive deficits and performed similarly to controls in a water maze [66]. Dietary 2’-fucosyllactose (2’-FL), a human milk oligosaccharide, has a potential cognition-boosting effect [67]. 2’-FL contains a monosaccharide, fucose, found in the synapses and is vital in cognitive function [68]. Vagotomy prevented the effects of oral 2’-FL on long-term potentiation (key mechanism in learning and memory processes) in rats [69]. Wang and colleagues [70] reported that the subdiaphragmatic vagus nerve plays a vital role in the pathogenesis of depression and is a major modulatory pathway between the brain and gut microbiota. The same group showed that subdiaphragmatic vagotomy blocked the development of depression-like phenotypes in mice treated with antibiotics after fecal matter transfer [71].
In clinical studies, supplementation with B. breve A1 helped improve cognitive function in older adults who experience mild cognitive impairment [72–74]. In another clinical study with probiotics, Georgescu and colleagues [75] have shown that Lactobacillus acidophilus and Bifidobacterium infantis can reduce abdominal pain and bloating in PD patients. Tamtaji et al. [76] administered probiotic treatment or placebo for 12 weeks to PD patients and found decreases in motor symptoms, C-reactive protein, malondialdehyde (a marker for oxidative stress), and increased glutathione (antioxidant).
SCFAs, the primary metabolites produced by bacteria in the gut, affect GBA and psychological function via the GI tract. Ho et al. [77] found that select SCFAs, especially butyric, valeric, and propionic acids, reduce beta-amyloid accumulation, which suggests the hope for SCFAs in managing AD. PD patients, too have fewer SCFA-producing bacteria and lower SCFA levels in the stool samples compared to healthy controls [78], suggesting that beneficial effects of SCFAs may extend to other neurodegenerative conditions.
Stress
Mechanisms of stress
Stress impacts the body via GBA, and gut bacteria profoundly impact well-being and response to stress and anxiety [79–81]. Daily or prolonged stress, with substantial increases during the COVID-19 pandemic [82], can detrimentally affect physical, mental, and cognitive health.
When experiencing a stressful event, the amygdala (a brain area that controls emotions) sends a signal to the hypothalamus (brain command center) [83], which consequently alerts the nervous system. CNS then releases a flood of stress hormones, including adrenaline and cortisol (neuroendocrine stress marker) (Figure 3). Stress-induced corticotropin-releasing hormone (CRH) from the amygdala and hypothalamus initiates the HPA axis; it also acts on mucosal mast cells to increase the release of tumor necrosis factor-alpha which leads to increased gut permeability [84].
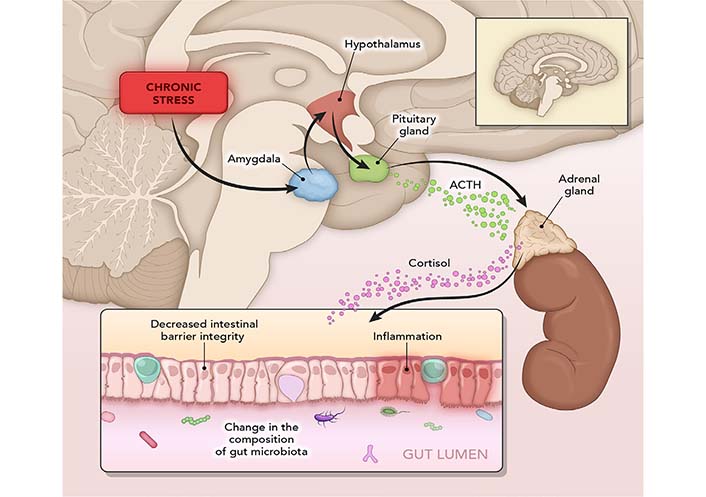
Mechanisms of stress. In a stressful event, the amygdala sends a signal to the hypothalamus, which triggers an alert through the nervous system. This releases a flood of stress hormones, including adrenaline and cortisol. Fight-or-flight response slows down digestion and increases gut sensitivity. Stress harms the intestinal barrier and can initiate a vicious cycle of inflammation. ACTH: adrenocorticotropic hormone
Chronic stress can manifest as physical symptoms such as headache, sleep loss, difficulty in concentrating, and skin issues [85]. In addition to the fight-or-flight response, repeated exposure to stress can initiate a vicious cycle of inflammation and negatively impacts the intestinal barrier [86, 87]. Stress hormones, such as cortisol, can affect intestinal barrier integrity and immune signaling within the gut and change gut microbiota composition. Indeed, Geng et al. [88] demonstrated how psychological stress alone had significant effects on the composition and diversity of gut microbiota in rats. Those researchers further characterized the negative impact of stress on intestinal and BBB, including decreased expression of tight junction proteins, looser tight junctions, and broken basement membrane.
Dietary supplements—modulating the gut microbiome
Preclinical data
In rats, prebiotics polydextrose (PDX; branched polymer of glucose and sorbitol) and galactooligosaccharides (GOS; linear polymer of galactose) were a promising treatment for behavior, using the model which mirrors symptoms of human depression and anxiety. These prebiotics also reduced the expression of a stress-evoked marker for neuronal activity (c-fos) in the dorsal raphe nucleus (a brainstem structure important for learned helplessness behaviors). It modulated gene expression in neural circuits essential in stress resistance [89]. Rats injected with LPS that received GOS in drinking water demonstrated fewer anxious behaviors than those not fed GOS [90]. Similarly, rats fed GOS were more exploratory, more social, and showed fewer depression- like behaviors compared to controls [91]. In young pigs, a diet with PDX impacted exploratory behavior, recognition memory, and neurochemistry [92].
Sialylation contributes to the structural diversity of glycoconjugates (sugars linked to other biological molecules) which have a critical role in neural development, synapses functioning, cognition development, and memory formation [93]. In a mouse model, a social disruption stressor caused anxiety-like behavior in control mice but not in mice fed acidic oligosaccharides, 3’- or 6’-sialyllactose (3’-SL or 6’-SL) for two weeks before stress induction. Their results suggested that sialyllactose helped to maintain baseline behavior [7]. Diet with 3’-SL and 6’-SL also protected colonic microbiota from stress (e.g., maintained beta diversity) [7]. Rodents in the stress group fed 3’-SL and 6’-SL demonstrated behavior like non-stressed controls, pointing to a protective effect of sialyllactose.
In a double-blind, randomized placebo-controlled study, participants received 5.5 g/day of GOS, FOS, or maltodextrin (placebo) for three weeks [94]. GOS intake reduced anxiety behavior, accompanied by physiologically reduced waking cortisol and fewer prominent rises and falls in cortisol. Sleep quality, stress, and gut microbiome were assessed in adults 30–50 years of age who consumed a dairy-based product with added GOS and vitamins and minerals over three weeks (1 h before bedtime) [95]. The test diet reduced salivary cortisol and stimulated beneficial bacteria. Another clinical study reported better cognitive flexibility and sustained attention performance in healthy adults receiving PDX supplement [96]. Finally, supplementation with oligofructose-enriched inulin improved memory tasks and mood [97].
Patients with chronic fatigue syndrome (N = 35) showed improved anxiety symptoms after two months of supplementation with Lacticaseibacillus casei [98]. In another randomized, double-blind study (N = 40), a combination of L. acidophilus, L. casei, and Bifidobacterium bifidum for eight weeks improved the Beck Depression Inventory scores [99]. Recently, Nishida and colleagues [100] reported the stress-relieving effects of probiotic Lactobacillus gasseri CP2305 and suggested that bacterial cell components may contribute to this activity. Several studies have indicated that other probiotics may also have the potential to help reduce physiological markers of stress [101, 102].
Conclusions
Nutrition and diet can influence the gut microbiome and impact cognitive function and behavior. Unbalanced nutrition, aging, and a sedentary lifestyle cause disturbances in gut microbiota and may lead to chronic inflammation. This can further result in neuronal loss, with unfavorable changes potentially leading to the release of gut-derived inflammatory mediators and the pathogenesis of AD, PD, and other disorders. Restoration of healthy gut microbiota via nutritional supplementation might provide a practical approach for preventing and managing many disorders. In addition, the development of probiotic strains that modulate the GBA could be promising as a complementary approach for enhancing mental and cognitive health.
Abbreviations
2’-FL: | 2’-fucosyllactose |
3’-SL: | 3’-sialyllactose |
AD: | Alzheimer’s disease |
BBB: | blood-brain barrier |
CNS: | central nervous system |
GABA: | gamma-aminobutyric acid |
GI: | gastrointestinal |
GBA: | gut-brain axis |
GOS: | galactooligosaccharides |
HPA: | hypothalamic-pituitary-adrenal |
LPS: | lipopolysaccharides |
PD: | Parkinson’s disease |
PDX: | polydextrose |
REM: | rapid eye movement |
SCFA: | short-chain fatty acids |
Declarations
Acknowledgments
We want to express our thanks to Dr. Jennifer Wampler for reviewing our draft. We thank the medical illustrators at www.haderermuller.com who helped us to visualize many concepts in this manuscript.
Author contributions
MC contributed conceptualization, original draft, and editing; JC, NF, NP review and editing. All authors contributed to the article and approved the submitted version.
Conflicts of interest
The authors declare that they have no conflicts of interest.
Ethical approval
Not applicable.
Consent to participate
Not applicable.
Consent to publication
Not applicable.
Availability of data and materials
Not applicable.
Funding
Not applicable.
Copyright
© The Author(s) 2022.