Abstract
Brain development, a complex process, consisting of several phases, starting as early as two weeks after conception, and continuing through childhood till early adolescence, is crucial for the development of properly functioning body systems, behavioral traits, and neurocognitive abilities. Infancy and childhood are recognized as important periods for initial brain formation, however in later stages of life, such as childhood and adulthood, experiences, together with environmental exposures, can still influence brain physiology. The developing brain is particularly susceptible to epigenetic changes with many factors being proposed as modifiers by directly impacting DNA methylation as well as histone and chromatin modifications within genes implicated in development. These factors include: maternal stress and diet, exposure to pollutants, sleep quality, as well as dietary habits. Evidence indicates exposures to environmental threats can lead to inappropriate neurological, metabolic, and endocrine functioning often mediated by epigenetic mechanisms with symptoms manifesting themselves as early as childhood or in later stages of life. Therefore, the main aim of this review is to evaluate the current studies focused on negative environmental exposures and their consequences on the developing brain directed by epigenetic mechanisms.
Keywords
Environmental factors, epigenetic modifications, brain development, nutrition, maternal factors, sleep quality, neuropsychiatric disordersIntroduction
Infancy and childhood are both critical periods for rapid brain development. Both periods enable the newborn to take in new information, as well as acquire new skills and traits contributing to the proper experience at their later stages of life [1]. The process of brain development begins about 2 weeks after conception and continues until early adulthood, usually 20 years of age. Briefly, this process involves 7 main stages, including neurulation, which involves the divergence of central nervous system (CNS) components (brain and spinal cord) from the neural plate to form the hollow tube [2]. During this time, after the neurulation, 6 stages are involved, namely proliferation, cell migration, differentiation, synaptogenesis, synapse pruning, and final myelination (Figure 1).
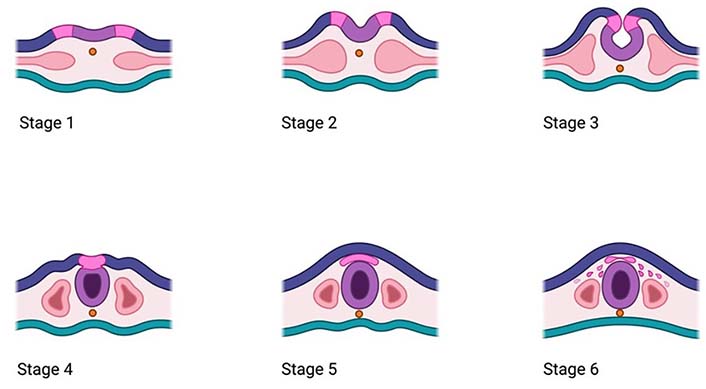
Main stages of the neurulation process including the development of the neural tube
In general, the process of brain development starts from the establishment of the general structure of the neural tube, which once completed, can progress into the second stage, known as proliferation, which includes cell multiplication and formation of the ventricular and marginal zones, being sources of specialized neuronal cells with structures like axons and dendrites, enabling them for further connection and signal transduction. As these progresses, the number of these specialized cells is increasing, which explains why the newborn brain has more neurons than an adult. That overproduction is gradually corrected, depending on the needs during apoptosis, a programmed cell death, being upon genetic control [3]. Once an appropriate number of neuronal cells is produced, these cells can travel to their final destinations outside of the marginal zones, in the next stage known as migration. In that phase, the cells deposited in the deeper layers of the cortical layer, subsequently migrate through formed layers to reside on the most outer neuronal layer. Once these neurons reach their target site, the next phase of differentiation takes place, which allows neuronal cells to form connections with other cells through specialized structures of axons and dendrites; otherwise, they will be deducted through apoptosis. The neurons that successfully form a connection, and then undergo maturation upon the point of contact between synapses of other neurons deposited nearby in the process known as synaptogenesis, which takes place around the 23rd week of gestation. Noteworthy, the rate of synapse production can depend on the acquired learning experiences and exposures, which may further influence synaptic timing of plasticity and synapse formation. As the process of formation is a highly dynamic process, it may result in synapse overproduction, which is corrected in the following stage of synapse pruning, which occurs in childhood, usually between 4 years and 6 years of age. As synapse pruning occurs predominantly in the brain areas involved in the higher cognitive functions, such as self-control, emotional regulation, and behavioral traits, thus allowing for the development of adaptive capabilities depending on the environment and experiences. Finally, once the appropriate number of synaptic connections is formed, the final stage of brain development known as myelination has taken place. This involves myelin sheet formation on the neurons, which as fatty isolation, facilitates neuronal activity and communication by speeding up the transmission of the electrical, which appears to be slower in the case of unmyelinated axons. Consequently, depending on the brain structure, the rate and level of myelination can vary as in the sensory and motor areas this process occurs much sooner than in the prefrontal cortex, which is implicated in the control of higher cognitive abilities and is not completed until early adulthood [1].
Brain development is a complex process, whose rate can evolve through the life cycle. Although the majority of the anatomical changes occur predominantly in the prenatal period and early childhood, the formation and refinement of neural networks can be modified at later life stages, thereby allowing for adjustments in the behavior and movements, which may depend not only on the genetics and environmental factors [1], but after 5 initial years of life may also be modified by acquired life experiences [4]. To date, various environmental factors have been implicated, the exposure to toxic substances (e.g., alcohol, heavy metals, smoke, air pollutants) and certain life factors, like those related to both infant and mother nutrition, sleep, and stress during pregnancy, seems to have the most significant impact on the brain development and function at any life stage [1]. The exact mechanism of how environmental exposures can impact brain development through epigenetic modifications [5] may explain why early life experiences can have lifelong effects. Consequently, exposure to environmental factors by impacting on epigenetic regulation through DNA methylation, histone modification, and accessibility of genetic material located in the chromatin, can significantly influence the expression of genes that are involved in the development and regulation of brain function [1]. For instance, among environmental factors, nutrition and the presence of certain nutrients appear to be the most critical owing to the evidence supporting their ability to directly impact on epigenetic control by suppressing key enzymes such as DNA methyltransferases (DNMTs) and histone deacetylases (HDACs) or by changing the availability of the substrate required for certain enzymatic activities, nutrients can exert their effects on epigenetic modification. In turn, this alters the way important genes express themselves, influencing our general wellbeing and longevity [6].
Accounting for the important role of environmental exposures in the epigenetic regulation of brain development, the main aim of this review is to evaluate the present knowledge in the context of early life.
Role of epigenetic modifications in brain development
Epigenetics is the study of genetic control that does not change the underlying DNA sequence but can regulate which genes are turned on or off [7]. It involves influencing the accessibility of certain genes to transcription factors by chemically modifying DNA bases or histone tails and changing chromatin structure. The major epigenetic mechanisms include DNA methylation, which is associated with heterochromatin. It works by adding a methyl group onto the C5 position of the cytosine. The methylation is laid down by various enzymes known as DNMTs. There are various types of these enzymes: DNMT1, DNMT3a, and DNMT3b. DNMT1 is particularly abundant in brain, maintaining genetic heritability of DNA methylation by replicating this mark at each cell division [8].
Post-translational histone modifications such as methylation, acetylation, phosphorylation, and ubiquitination, can also modulate the compaction of chromatin [9]. Histone acetylation is usually associated with euchromatin as an acetyl group is added to lysine, reducing the histone’s positive charge, hence its attraction to the negatively charged DNA [10]. Histone methylation however does not change the charge of the histone and can be associated with both active and silent genes depending on the degree of methylation and the location of the methylated section [11]. Epigenetic marks, such as DNA methylation, histone acetylation, or non-coding RNA, can change through the lifespan to direct proper development but can also be altered depending on the environment of the individual.
Soon after conception most of the gametic epigenetic patterns are completely erased and reprogrammed to allow the formation of genetic material of the new organism in a stable way. Global DNA demethylation takes place, which is then followed by re-methylation to reprogram the efficient gene expression regulation. As the fertilized egg develops, the record of inherited epigenetic tags on the DNA is modified depending on both internal and external stimuli reaching the developing foetus; thereby making early life the most vulnerable period for acquiring any potential epigenetic errors, which could lead to serious impairments in the foetus [12].
The failure of adequate epigenetic control during this critical process of foetal development, especially in the genes implicated in neurodevelopment, can play a role in the inheritance of disorders such as autism spectrum disorder (ASD). This can happen through processes such as aberrant methylation of the gene encoding methyl 5’-C-phosphate-G-3’ (CpG) binding protein 2 (MECP2), which is critical for the maturation of hippocampal neurons [13]. Furthermore, epigenetic dysregulation was found in schizophrenia and epigenetic/environment disturbances in early nervous system development may play a role in this [14]. After birth, in early childhood as well as in early adolescence, the organism is exposed to a greater variety of environmental factors and remains sensitive to epigenetic modifications, which are involved in shaping neuronal circuits of the developing brain, as well as the timing of sexual behavior and regulation of the menstrual cycle. Interestingly, in adulthood, life experiences as well as long-term exposures to environmental stressors (e.g., ranging from drugs, diet, exercise, social interaction, pollution, or menopause) can still modify epigenetic marks, gene expression, and thus the body’s functioning [12].
To date, there is growing evidence suggesting that epigenetic modifications might mediate the effects of acquired life experiences and environmental exposures on brain development; thereby influencing gene expression and cellular function in brain structures. For example, maternal care during infancy led to changes in DNA methylation patterns in the hippocampus of rats, which were associated with differences in stress reactivity and cognitive function later in life [15]. This study will be elaborated on below. Consequently, epigenetic modifications can serve as a mechanism through which environmental exposures and life experiences can shape brain development and function. Moreover, recent studies have shown that lifestyle targeted interventions [16] may have the potential to reverse adverse epigenetic modifications. These interventions include treatment programs aiming to improve diet and sleep quality, incorporating physical exercise and relaxation techniques, and calibrating circadian rhythm. Epigenetic modifications are reversible with the aid of epigenetic regulators, which lay the theoretical basis for aging modulation and make them promising targets for aging-intervention strategies [17]. Nevertheless, this early concept needs to be further investigated in order to better understand the modificatory role of environmental exposure in shaping human health in a long-term perspective.
Environmental exposures and their role in epigenetic regulation
Developmental Origins of Health and Disease (DOHaD) is a hypothesis explaining the impact of exposure to environmental factors occurring during foetal development in determining the health status of the newborn, as well as the health condition in the later life stages [18]. To date, evidence has shown that any interference with adverse environmental exposures occurring in the foetal life can lead to health complications in the foetus [19]. It can be reflected in later life, leading to impairments in the metabolic or endocrine function, as well as adverse neurodevelopment, resulting in the neurodevelopmental disorder or mental health problems [19]. Furthermore, exposure to environmental factors, especially in the vulnerable period between conception to early childhood, can significantly impact on epigenetic regulation, also which is implicated in the process of brain development [20]. Many factors have been proposed as potential initiation of epigenetic modifications: inadequate maternal nutrition, such as starvation [21], as well as experiencing traumatic events [22] during pregnancy, and high maternal levels of cortisol, a stress hormone also implicated in brain development and maturation of the CNSs [23], all of which can be fatal for the developing child. These factors in the context of initiating epigenetic modifications will be discussed in the sections below.
Maternal stress and foetal environment
Research exploring the impact of maternal care during the first week of life on acquired epigenetic modifications in the offspring, along with their long-term consequences present in later life, provided early evidence implicating the role of early life experiences in shaping brain development [15]. For example, a study using rats found an association between the patterns of epigenetic markers in the hippocampal tissue of the offspring and the level of maternal care, characterized by licking/grooming and arched-back nursing (LG-ABN) behavior [15]. By categorizing animals to those with high LG-ABN and low LG-ABN behavior, significant variation in epigenetic regulation of the nuclear receptor subfamily 3 group C member 1 gene (NR3C1) promoter in the adult offspring of high and low LG-ABN mothers was observed. NR3C1 encodes glucocorticoid receptors (GRs), which are proteins that regulate the transcription rates of glucocorticoid-responsive genes positively or negatively. Glucocorticoids, such as cortisol, are vital hormones important for controlling the body’s response to stress [24]. Adult rat males raised by high LG-ABN mothers had significantly less methylation of GR DNA compared to adult males raised by low LG-ABN mothers. Therefore, the GR gene of adult males raised by high LG-ABN mothers was more accessible to transcription factors. In addition, adult offspring of high LG-ABN mothers had significantly greater histone H3 lysine 9 (H3-K9) acetylation than the offspring from low LG-ABN mothers [15]. Therefore, offspring with low LG-ABN mothers produced offspring with differential hypothalamic-pituitary-adrenal (HPA) responses to stress [15].
There is some evidence indicating that adverse life experiences, like trauma, death of a close relative, environmental disasters, unemployment, or poverty, have been linked to the shorter duration of the pregnancy, as well as low birth weight; being explained by the prolonged exposure to glucocorticoids, which are steroid hormones produced by the adrenal axes as a response to stressful events [25]. They can pass through the maternal placenta and can affect the foetus [26]. In addition, prenatal anxiety might also disrupt the function of enzymatic barriers of the placenta, including enzyme 11β-hydroxysteroid dehydrogenase type 2 (11β-HSD2), leading to higher glucocorticoids and cortisol levels in the foetal environment. The overexposure to stress hormones in the developing foetus, because of prolonged maternal stress has been associated with the reduction of the frontal, temporal, and limbic thickness of the developing foetal brain, followed by the subsequent alterations in the emotional and behavioral regulation in later stages of life [27]. A human study found the correlation between stress during pregnancy and changes in brain epigenetic patterns of offspring [28]. Eighty-three mother-child dyads who were exposed to higher maternal anxiety during the third trimester produced offspring with higher methylation levels of four CpGs from NR3C1 (i.e., CpG3, CpG5, CpG6, CpG10) at 4 years of age. However, weak evidence was found to directly show how this affected child behavioral measures at four years of age [28]. Furthermore, prenatal exposure to inflammation has been implicated in the development of schizophrenia, ASDs, and bipolar disorder [29]. A study conducted on female and male mice found evidence supporting the role of prenatal infection on epigenetic changes seen in neurodevelopmental disorders [30]. A maternal immune activation (MIA) model was administered to pregnant dams on gestation day 9. They observed long interspersed element 1 (LINE-1) hypomethylation, in the adolescent rodent brain. Disruption of LINE-1 transposon methylation status could disturb the stability of the genome, especially in early development. In fact, an elevated LINE-1 expression has been reported in schizophrenia, showing the possibility that epigenetics may mediate the link between prenatal infection and neurodevelopmental problems later in the life of offspring [30]. In addition, the MECP2 gene promoter region was significantly hypomethylated in the hypothalamus of MIA-exposed mice, with its aberrant expression also being associated with autism [30].
Exposures to toxins and pollutants
Maternal substance abuse is an important environmental factor, which can have a negative impact on the developing brain. For example, tobacco use during pregnancy can have serious consequences for the foetus; and has been shown to adversely impact on the foetal brain development and lead to altered gene expression upon acquired DNA methylation [31] of the genes implicated in the neuronal differentiation. For example, transcription factor octamer-binding transcription factor 3 (Oct3)/Oct4 can possibly affect the process of embryogenesis [32]. A study exploring the effect of maternal smoking during pregnancy on the global profiles of DNA methylation in the areas of the developing dorsolateral prefrontal cortex (DLPFC) during the second trimester of gestation, found genome-wide DNA methylation changes. These epigenetic markers were significantly reduced in the foetal cortex of newborns exposed to maternal smoking, showing alterations in cell-type differentiation [31]. In addition, the results of this study also reveal a potential link between maternal smoking-associated DNA methylation and increased risk for neurodevelopmental abnormalities as the methylation changes were associated with reduced mature neuronal content. Furthermore, this study found that the degree of methylation in the promoter region of the glutamate receptor gene was associated with the severity of neonatal abstinence syndrome (NAS) in opioid-exposed infants. NAS is a constellation of symptoms that occur in newborns exposed to opioids in-utero, and its severity is thought to be an indicator of the extent of prenatal opioid exposure [31].
These results were consistent with another study, investigating the developing brain of mice pups born by mothers who were exposed to electronic cigarette (e-cigarette) aerosols [33]. The epigenetic analysis conducted on the brain tissue samples at 1 day, 20 days, and 13 weeks after birth indicated increased levels of global DNA methylation in the brain samples of pups born from dams exposed to e-cigarette aerosols. The change in DNA methylation patterns can also potentially alter epigenetic stability for later life as maternal exposure to e-cigarette aerosols, with or without nicotine, leads to a decreasing trend in the Dnmt3a/Dnmt3b gene expression level, particularly in adulthood [33]. As DNA methylation is maintained by enzymes known as DNMTs, exposure to nicotine might negatively impact their function [34].
Furthermore, maternal exposure to e-cigarette aerosols with nicotine during pregnancy leads to short-term memory deficits in offspring, confirmed by the novel object recognition (NOR) test, where this group did not show an increase in the recognition index. In mice pups not exposed to nicotine, this did not occur. Interestingly, however, pups exposed to e-cigarettes with nicotine showed reduced anxiety and were more prone to take risks and explore new environments when compared to pups exposed to smoke with no nicotine. Epigenetic modifications can also arise as the result of chronic drug use. An experimental study conducted on pregnant mice, which were administered cocaine twice daily on gestational days 8–19, reported to have increased methylation status on multiple CpG islands in genes involved in neuronal functioning in the hippocampus in offspring of cocaine-exposed mothers [35]. One of the hypermethylated genes was the G-protein-coupled receptor (GPCR)-73 which functions to support neuronal survival in the CNS. Interestingly, upon chronic exposure to cocaine multiple gene promoter regions were found to be hypomethylated CpG islands, such as those encoding coenzyme Q7 with roles implicated in mitochondrial respiration and neurogenesis. Finally, the researchers reported elevated expression of DNMTs, DNMT1 and DNMT3b, in hippocampal pyramidal cells and increased global methylation of the offspring analyzed 30 days after birth with cocaine-exposed mothers [35]. These factors suggest that substance abuse during pregnancy may cause significant genomic and neurochemical implications in later life [35].
Similarly, to tobacco, air pollutants [e.g., nitrogen oxides, sulfur dioxide and carbon dioxide, and particulate matter (PM)] are considered as other important factors that can adversely impact brain formation, due to increased emissions caused by anthropogenic activity. It is estimated that over 90% of people breathe the air that does not meet the WHO standards [36]. Early life exposure to air pollutants has been shown to lead to impaired neurodevelopment and has been linked with neurological dysfunctions [37], such as functional impairment of the CNS [38] or ASD, which has been positively linked to exposure to air pollution [39]. Furthermore, there is an increased probability of developing neurological diseases and other health problems later in life [40], such as Alzheimer’s disease [41]. Epidemiological evidence obtained from the cohort studies have shown that exposure to air pollution, defined as the average concentration of air pollutants (e.g., PM, nitrogen dioxide, sulfur dioxide, and ozone) in the first trimester of the pregnancy can lead to foetal underdevelopment and preterm birth [42]. Observations conducted in a Polish cohort, consistently indicated that in-utero exposure to polycyclic aromatic hydrocarbons (PAHs) was associated with a lower intelligence quotient (IQ) score in children at the age of 5 years old [43]. Similarly, exposure to environmental toxins, such as lead and bisphenol A (BPA), has been shown to lead to epigenetic changes in the developing brain, which may contribute to the development of neuropsychiatric disorders [44]. Also, inhalation of PM, a major component of air pollution, emitted from various sources upon industrial activities [45] has been shown to increase the risk of neural tube deformation and anencephaly in newborns [46] as well as delayed neurodevelopment in early childhood [47]. Long-term exposure to air pollution has been linked to cytogenetic damage, epigenetic changes, changes in gene expression, and increased risk of cancer development [48]. Furthermore, air pollution is thought to trigger pulmonary inflammation via inducing epigenetic changes in the lungs [49].
Caffeine consumption during pregnancy may affect foetal neurodevelopment, as caffeine can cross the placenta and reduce the metabolic rate. Animal studies have shown that caffeine may interfere with brain zinc fixation, which alters sleep, locomotion, learning abilities, and anxiety, but human studies have only found an increased risk of hyperkinetic disorders and attention-deficit/hyperactivity disorder (ADHD) in children when mothers consume more than 10 cups of coffee per day during early pregnancy [50]. One study found that the offspring of mothers with a caffeine intake higher than 200 mg per day had a 2-fold higher risk of altered cognitive development and IQ impairment at 5.5 years old [51]. More research is needed to confirm the association between extreme levels of maternal caffeine consumption and neurodevelopmental conditions in offspring.
Sleep
Sleep, being defined as a recurrent state of reduced responsiveness to the external environment, is essential for proper functioning at any stage of life [52]. Not surprisingly, high-quality sleep, appropriate to age, is crucial for brain regeneration, as well as consolidation of cognitive functions, including memory and decision-making processes. For children up to 5 years of age, both night sleep as well as short napping throughout the day can bring benefits for cognitive functioning and promote declarative memory performance. It is particularly important for younger children, such as 15-month infants, as a nap taken in the span of 4 h after being exposed to new content, increases information recollection after 24 h, in comparison to after not taking a nap [53].
Sleep can be divided into two main stages, which include rapid eye movement (REM) and non-rapid eye movement (NREM) sleep. NREM sleep is also known as relaxation for the body and the brain. On the other hand, REM sleep is characterized by REM and muscle twitching. It is believed to be the stage where dreaming is most likely to occur [54], as well as responsible for memory consolidation [55]. Other functions are believed to be the maturation of the visual cortex [56], and the motor circuit [57, 58]. The required amount of REM sleep throughout life is related to intense brain development, including synaptogenesis and neuronal plasticity, thereby indicating a crucial role in brain maturation. Interestingly, it has been hypothesized that in the later stage of life, REM sleep can be exchanged for wakefulness, as the percentage of NREM increases along with the age. As both REM sleep and wakefulness are considered as the time of high neural activity, developing organisms would need to spend more time in wakefulness to receive information, while more NREM sleep would be needed for regeneration and rest [59]. Evidence to date supports the role of REM sleep in brain maturation. It has been shown that muscle twitching present in the REM sleep stage might be involved in the activation of cellular responses within the sensorimotor networks, which in turn, can stimulate the sensorimotor cortices and distant structures of the brain, such as the brainstem and hippocampus. It has been shown that infants with decreased muscle twitching during REM had poor behavioral and neurological outcomes at a later age [60], which might be explained by the role of REM sleep in the neuronal stimulation needed for early brain development, in particularly synaptogenesis [61, 62].
In addition to REM, NREM sleep also plays a significant role in brain development; especially the third stage of NREM, namely slow wave sleep (SWS), also known as ‘deep sleep’, being characterized by the slow brain wave frequency, followed by decreased neuronal activity, often reflected by slowing down in breathing and heartbeat. The reduced brain activity occurring in the SWS phase of NREM sleep allows it to consolidate and integrate newly acquired information and prune unnecessary neural networks [63]. This might be of particular importance in the critical periods of brain development through synaptic depression, the decrease in the probability of synapses firing [64, 65]. These periods include infancy and adolescence [66] when brain plasticity is the highest and susceptible to environmental influences [67].
Not surprisingly, sleep deprivation, as well as poor sleep quality, especially experienced in the early life periods, have been shown to negatively impact brain development, leading to impaired neurodevelopmental functions. In an early experimental study conducted on cats, reduced REM sleep caused lower plasticity in brain regions stimulated before, which may indicate that sleep deprivation, especially a shortening REM cycle, can lead to reduced brain plasticity [56]. The following observations were also reflected in the human studies, which demonstrated an association between sleep duration and grey matter density in the hippocampal region in the brain in children aged between 5 years old and 18 years old [68]. The hippocampus is believed to be responsible for learning, memory, and spatial navigation [69], decreased grey matter density could lead to impairment of those functions in affected individuals. In addition, studies are investigating the effects of obstructive sleep apnea (OSA), a common sleep disorder resulting from spontaneous temporal relaxation of throat muscles or narrowing airway pathways, leading to difficulties in breathing, have shown that children with moderate to severe OSA, tend to wake up during the night and they have significantly reduced grey matter volume in the brain [70]. In addition, children with OSA also were reported to have a decreased brain matter density in the brain areas, such as superior frontal, prefrontal, and lateral cortices, which are responsible for mood state and cognitive functions [71].
Although there are many mechanisms in how disrupted sleep patterns can adversely impact on brain development, there is some indication that they can also lead to epigenetic modification in the developing brain, inducing DNA methylation or histone acetylation. Some recent studies conducted on the murine models have shown that sleep deprivation in rats during a critical period of brain development can lead to changes in DNA methylation patterns in the expression of memory and neurotransmitter levels [72]; whereas chronic sleep restriction results in the changes in noradrenaline receptor density causing neurocognitive impairments [73]. These early findings may suggest that altered sleep patterns can have long-lasting effects on brain development through epigenetic modifications, which may have implications for the development of neuropsychiatric disorders, such as schizophrenia and depression [74, 75]. Evidence shows that SWS of NREM sleep can increase histone acetylation and other epigenetic modifications in the brain regions responsible for learning and memory processes [76] contrastingly to sleep deprivation, which has been shown to impair histone acetylation in the hippocampus and negatively affect memory consolidation and information recall [77]. These epigenetic modifications may be critical for the consolidation of spatial memory in murine models [78] thus targeting that pathway should be further explored as a promising therapeutic approach for enhancing learning and memory in individuals with cognitive impairments, such as those with Alzheimer’s disease or other neurodegenerative disorders.
Nutrition
The importance of the diet in achieving optimal wellbeing is dictated by both the quantity and quality of the nutrients provided in the consumed foods, which should sustain adequate amounts of essential macro and micronutrients. Consequently, early life nutrition as well as maternal dietary habits during pregnancy seem to play a significant role in the child’s health, particularly during periods dedicated to neurodevelopment and failure to sustain nutritional demands in that early life stages might adversely influence the newborn growth and formation of metabolically active tissues [79]. Examples include folate deficiency, which can cause foetal neurodevelopmental defects, vitamin D3 deficiency, which can increase the risk of developing ADHD or ASD, and iron deficiency, potentially causing reduced language ability, fine motor skills, and intelligence, as well as behavioral and memory deficits [80, 81].
Undernutrition, defined as insufficient intake of energy and nutrients to meet an individual’s needs to maintain good health during gestation has been shown to negatively impact the foetal development of the brain [82]. A developing brain with highly metabolically active tissue can consume more than half of the supplied energy, and an insufficient amount of its main source—glucose, a simple carbohydrate can adversely impact neural proliferation, and cause neurodevelopmental deceleration [83]. Similarly, shortage of protein has been also linked with negative effects on neuronal differentiation, as well as mitochondrial function, and can lead to apoptosis by causing cellular stress and triggering apoptotic pathways that result in programmed cell death [81, 84, 85]. Although undernutrition can negatively impact on developmental processes, it can also induce epigenetic changes. For instance, malnutrition has been correlated with the expression of genes encoding for crucial regulators of the HPA, such as GR and proopiomelanocortin hormone in the foetal hypothalamus [86]. The HPA axis is a key regulator of the body’s response to stress, and it plays an important role in brain development and function, changes in the HPA axis function can result in increased susceptibility to stress related psychiatric disorders such as depression and anxiety as well as alterations in cognitive functions and brain structure [87, 88].
Maternal undernutrition leads to intrauterine which several studies have linked with lower IQ scores [89]. A systematic review and meta-analysis of 35 studies involving over 8,000 children found that those born with intrauterine growth restriction (IUGR) had, on average, lower IQ scores than those born with normal birth weights [90]. In addition to lower IQ, IUGR has also been linked to an increased risk of schizophrenia. A meta-analysis of 15 studies involving over 5,000 individuals found that those born with IUGR had a more than twofold increased risk of developing schizophrenia compared to those born with normal birth weights [91].
In similar manner, overnutrition, a result of excessive intake of nutrients, leading to the accumulation of body fat that negatively impacts on health and general wellbeing, such as consumption of high-fat diet (HFD) during pregnancy has been shown to negatively impact on brain development in newborns [81, 92–94]. That imbalanced nutrition exceeding the energy needs of the organism may result in increased cell proliferation in the hypothalamus and decreased rate of apoptosis. Additionally, HFD has been linked with higher risks of mental and behavioral disorders (e.g., anxiety, ADHD, and depression) in newborns, resulting from inflammatory alterations of the serotonergic neural system, the source of the neurotransmitter serotonin [95].
To date, studies have shown that newborns exposed to HFD during gestation have exhibited inflammatory mechanisms [96]. These mechanisms are caused by inflammatory cytokines, such as interleukin-6 (IL-6) and tumor necrosis factor-α (TNF-α), which can cross the placenta and affect foetal brain development by altering neurotransmitter systems, synaptic plasticity, and neuronal connectivity [96]. This can increase the risk of mental disorders, as children of obese mothers seem to have a 30% higher probability of developing ADHD and a 10% higher probability to suffer from ASD [97, 98]. Research has shown that mothers who regularly consumed lard, a source of saturated fats, during pregnancy, had a higher likelihood of giving birth to children with impaired spatial memory and learning abilities [99, 100]. This is thought to be due to the activation of microglial cells in the brain and decreased production of brain-derived neurotrophic factor (BDNF) in the hippocampus. The microglial activation and reduced BDNF production are believed to contribute to the cognitive impairments observed in these children [99, 100]. Interestingly, the results of a systematic review conducted on studies investigating the association between maternal overweight and obesity status before pregnancy and child neurodevelopmental outcomes indicated that children born of obese mothers had an increased risk of ADHD, ASD, developmental delay, as well as emotional and behavioral problems [101].
There is growing evidence that diets high in sugars may be a risk factor for pediatric mental health and neurodevelopmental disturbances. A study showed that maternal sugar consumption during pregnancy was associated with an increased risk of behavioral problems, including hyperactivity and aggression, in their children [102].
These effects might be explained by the increased inflammation resultant from maternal obesity, which may affect the establishment of appropriate signaling on certain neural pathways, such as those including serotonin and dopamine pathways, involved in behavior regulation and cognitive performance may increase child susceptibility to acquiring mental disorders. Furthermore, perturbed glucocorticoid secretion and maternal hyperactivation of HPA axis, followed by higher pro-inflammatory cytokine expression in the foetus might induce epigenetic modifications, that can be a cause of increased neurobehavioral morbidity [103].
Quality and quantity of certain macro and micronutrients, as essential components of a well-balanced diet, have been also implicated in the process of brain development and growth in early life. Among all macronutrients, fats, particularly polyunsaturated fatty acids (PUFAs), appear to be the most important for developing brain development. PUFAs are a group of essential fatty acids that play important roles in brain development and function which depending on the structure can be classified into two main families, omega-3 (n-3) and n-6. n-3 PUFAs, such as docosahexaenoic acid (DHA) and eicosapentaenoic acid (EPA), have been shown to be particularly important for brain health and cognitive function, owing to their important role in the formation of neuronal membranes, synapse maturation, and myelination [104]. An adequate intake of PUFA, especially during the third trimester of the pregnancy, has been linked with improved cognitive abilities and reduced risk of neuropsychiatric disorders in newborns, including schizophrenia, bipolar disorder, and anxiety [105]; as well as lower risk of decreased IQ values [81].
Maternal n-3 PUFA, particularly DHA during pregnancy, has been shown to be essential for the establishment of well-functioning brain structures, including dendritic growth and synaptogenesis in the developing foetus, as well as children of young age. For example, in 4- and 7-year-olds, adequate maternal DHA status in pregnancy, benefits brain function in childhood, which is demonstrated by better mental and sequential processing scores, as well as verbal abilities and visual acuity [106, 107]. In contrast, an insufficient supply of n-3 PUFA was proposed as a potential cause of impaired neurogenesis, neurotransmitter metabolism (specifically dopamine and serotonin), and learning processes [108]. Inadequate maternal levels of n-3 PUFA have been associated with impaired postmitotic cell migration, which refers to the movement of non-dividing cells, such as neurons and glial cells, during brain development to form functional neural circuits [109]. This impairment can lead to decreased embryonic cell development and underdevelopment of the hippocampus, a crucial brain region responsible for learning and memory [110]. Excessive intake of n-6 PUFA, such as linoleic acid, may result in altered stress response and thigmotactic behavior or avoidance of open spaces [111].
Protein is another important macronutrient for early life development. Although access to high-quality protein is required at any stage of life, it appears to be an essential nutrient during the second and third trimesters of pregnancy, when tissue growth is accelerated [112]. Interestingly, research has shown that high-protein diets during pregnancy can positively impact the motor and cognitive abilities of children, in areas responsible for information processing, numeracy, and vocabulary [113]. Contrastingly, protein restriction during foetal development can impair hippocampal neurogenesis, decrease brain and neuronal volume, and reduce myelin production, thereby leading to a higher risk of neuropsychiatric disorders [114].
Similarly, to macronutrients, an inadequate supply of certain micronutrients during pregnancy can influence foetal neurodevelopment, leading to long-term neurological deficits in newborns. In terms of minerals, iron deficiency during the first trimester can lead to long-term neurodevelopmental impairment, including memory alterations, motor deficiencies, social dysfunction, and low academic performance [115]. Certain important processes involved in neurodevelopment e.g., myelination, dendritogenesis, synaptogenesis, and neurotransmission, are dependent on iron-containing enzymes and hemoproteins [116]. Chronic foetal hypoxia can also contribute to developmental abnormalities. Both excess and deficient iron can affect offspring, with poor foetal iron status through reduced language ability, fine motor skills, and intelligence, as well as behavioral and memory deficits [117, 118].
Zinc deficiency during gestation can reduce cell counts and decrease brain mass in various regions of the brain. Zinc’s role in the metabolism of carbohydrates and proteins, nucleic acid synthesis, and cell division and differentiation play an important part in the neurodevelopment of the foetus [119]. Experimental studies conducted in rodents have shown that deficiency of zinc during gestation can lead to decreased cell counts and reduced regional brain mass in the cerebellum, limbic system, and cerebral cortex [120]. Similarly, iodine plays a vital role in foetal neurodevelopment as it is essential for thyroid hormone synthesis, whose absence can cause irreversible neurological damage [121]. A deficiency of iodine can cause a disruption of foetal neurogenesis, neural migration, synaptogenesis, and myelination. Maternal iodine deficiency during pregnancy can result in neurological damage in offspring, including impaired cognitive and motor function, learning difficulties, and decreased IQ scores [122].
In terms of vitamins, a deficiency of folate has been shown to alter foetal neurodevelopment through incorrect DNA methylation [123]. A sufficient intake of folate is required for proper neural cell proliferation, migration, differentiation, vesicular transport, and synaptic plasticity [124]; whereas vitamin D affects neurodevelopment by regulating the expression of genes. An insufficient intake of vitamin D in the maternal diet results in persistent changes in the structure and function of the brain throughout adulthood, affecting neuronal differentiation, neurotransmission, synaptic plasticity, axonal connectivity, and other biological pathways like oxidative phosphorylation, cytoskeleton maintenance, calcium homeostasis, chaperoning, and post-translational modifications. These changes suggest that vitamin D has a role in regulating gene expression and epigenetic processes [125, 126]. Current research has produced evidence showing the relationship between insufficient prenatal vitamin D intake and an increased risk of developing ADHD or ASD [127]. Lastly, the metabolites of vitamin A and retinoic acid have been shown to play a role in neural patterning, the development of neurons, the extension of neurites, and the elongation of axons. An insufficient intake can increase the risk of preterm delivery and maternal anemia [128].
Furthermore, choline appears to be another nutrient whose adequate supply is important for brain development. Choline is an essential component of cell structures, including those in the brain, it is required for the synthesis of phosphatidylcholine and sphingomyelin, two major phospholipids incorporated into cellular membranes; it is also involved in the production of acetylcholine, an important neurotransmitter responsible for memory and mood [129]. In addition, there is increasing evidence linking choline status with epigenetic modifications followed by lifelong changes in memory function in later life stages. An experimental study conducted in mice has shown that choline deficiency can result in changes in global methylation pattern changes in the developing hippocampus of foetal brains [130]. In the study, mice given diets deficient in choline content from days 12 to 17 of pregnancy had alerted DNA methylation at specific CpGs in the main proliferation area of the hippocampus, such as Ammon’s horn ventricular and subventricular zones, when compared to animals on standard diet. Interestingly, dietary choline deficiency induced hypomethylation of genes encoding proteins cyclin-dependent kinase 4 inhibitor B (p15INK4b) and cyclin-dependent kinase-associated protein phosphatase (KAP)—cyclin-dependent kinase inhibitors, further leading to their aberrant expression, which was associated with increased neural cell apoptosis in Ammon’s horn ventricular and subventricular zones [130].
Conclusions
Early life stages, such as infancy and childhood are known as critical periods for rapid brain development, during which new information is acquired, and new skills and traits are developed. The rate of brain development can be influenced by genetic and environmental factors, and even after the initial five years of life, acquired life experiences can modify brain development. Environmental factors such as exposure to toxic substances and certain life factors related to nutrition, sleep, and stress during pregnancy have a significant impact on brain development and function. Epigenetic modifications, including DNA methylation and histone modification, play a crucial role in mediating the effects of environmental exposures and life experiences on brain development [131–134]. It has been shown that neurodevelopmental disease indicates that epigenetic modifiers influenced by environmental stimuli, such as hypoxia regulating DNA methylation and histone remodeling are critical for normal brain development [133]. Noteworthy, the quality of parent-offspring interactions induces epigenetic changes in the developing brain that account for variation in response to stress, cognition, and sociality in children [15, 134].
Evidence to date indicates that several early life exposures linked with environmental insults, as well as those arising from maternal factors can influence brain development through epigenetic modifications [15, 49, 78, 94, 123, 134, 135]. For example, maternal care during infancy can lead to changes in DNA methylation patterns in the hippocampus, affecting stress reactivity and cognitive function later in life [15, 49, 134, 135]. Lifestyle interventions that improve diet and high sleep quality may have the potential to reverse adverse epigenetic modifications [78, 94, 123]. Epigenetic markers can also serve as predictors and diagnostic markers for aging, brain development, and neuropsychiatric disorders.
Several environmental factors during pregnancy have been associated with altered brain development. Inadequate maternal nutrition, traumatic events, maternal stress, prenatal infection, and substance abuse can all have negative effects on foetal brain development through epigenetic modifications. Air pollutants, caffeine consumption, sleep deprivation, and poor nutrition also impact brain development negatively.
Undernutrition during gestation can negatively affect brain development, including neural proliferation and neuronal differentiation. Protein and glucose shortages, as well as malnutrition, can induce epigenetic changes and alter the function of the HPA axis. IUGR has been linked to lower IQ scores and an increased risk of schizophrenia. In the opposite manner, overnutrition, such as consumption of HFD during pregnancy, can also negatively impact brain development. It can lead to increased cell proliferation in the hypothalamus and inflammation of the serotonergic neural system, increasing the risk of mental and behavioral disorders in newborns.
Overall, the evidence to date suggests that epigenetic modifications play a crucial role in mediating the effects of acquired life experiences and environmental exposures on brain development and function. Understanding these mechanisms can provide insights into the long-term effects of environmental exposures and help develop strategies for promoting optimal brain development and reducing the risk of neuropsychiatric disorders.
Abbreviations
ADHD: |
attention-deficit/hyperactivity disorder |
ASD: |
autism spectrum disorder |
CNS: |
central nervous system |
CpG: |
5’-C-phosphate-G-3’ |
DHA: |
docosahexaenoic acid |
DNMTs: |
DNA methyltransferases |
e-cigarette: |
electronic cigarette |
GRs: |
glucocorticoid receptors |
HFD: |
high-fat diet |
HPA: |
hypothalamic-pituitary-adrenal |
IQ: |
intelligence quotient |
IUGR: |
intrauterine growth restriction |
LG-ABN: |
licking/grooming and arched-back nursing |
LINE-1: |
long interspersed element 1 |
n-3: |
omega-3 |
NR3C1: |
nuclear receptor subfamily 3 group C member 1 gene |
NREM: |
non-rapid eye movement |
OSA: |
obstructive sleep apnea |
PM: |
particulate matter |
PUFAs: |
polyunsaturated fatty acids |
REM: |
rapid eye movement |
SWS: |
slow wave sleep |
Declarations
Acknowledgments
The authors of this manuscript would like to acknowledge members of the educational board of the Akademeia High School in Warsaw (Poland) for facilitating the opportunity of working on the manuscript.
Author contributions
MKF, JW, MR, GR, and AN: Conceptualization, Investigation, Writing—original draft, Writing—review & editing. JMJ: Conceptualization, Data curation, Writing—review & editing, Supervision. All authors read and approved the submitted version of manuscript.
Conflicts of interest
The authors declare that they have no conflicts of interest.
Ethical approval
Not applicable.
Consent to participate
Not applicable.
Consent to publication
Not applicable.
Availability of data and materials
Not applicable.
Funding
Not applicable.
Copyright
© The Author(s) 2023.