Abstract
The circadian rhythm is a critical system that governs an organism’s functions in alignment with the light-dark cycle. Melatonin release from the pineal gland plays a crucial role in regulating the internal clock of the body. Multiple neurotransmitter systems in the central nervous system are linked to the release of melatonin. In this review, the relationship between circadian rhythm, melatonin secretion and various neurotransmitter systems are mainly discussed. Serotonin regulates the circadian rhythm through projections from raphe nuclei. Agomelatine is an example of the synergistic interaction between melatonin and serotonin. Melatonergic agents and selective serotonin reuptake inhibitors also exert notable impacts on depression in concomitant use. Dopamine has an inhibitory effect on melatonin release, while melatonin also inhibits dopamine release. This should be taken into account when considering the use of melatonin in Parkinson’s disease. On the contrary, use of melatonin may offer therapeutic advantages for schizophrenia and tardive dyskinesia. The interaction between norepinephrine and melatonin exhibits diurnal variability, with norepinephrine promoting arousal and inhibiting daytime melatonin secretion. Melatonergic neurons also exert a specific protective influence on cholinergic neurons. Interaction between the histaminergic and melatonergic systems is significant, particularly in association with immunity, sleep, and circadian rhythm. Novel ligands with dual-acting properties, interacting with both the histaminergic and melatonergic systems are investigated. Currently, there is a limited number of approved melatonergic agents that primarily demonstrate positive effects in addressing insomnia and depression. However, there is considerable potential in studying new agents that target both the melatonergic and other neurotransmitter systems, which alleviate various conditions, including neurodegenerative diseases, dementia, autoimmune diseases, allergic diseases, epilepsy, and other neuropsychiatric disorders. The ongoing process of developing and evaluating new ligands selectively targeting the melatonergic system remains crucial in understanding the complex relationship between these systems.
Keywords
Circadian rhythm, neurotransmitter, melatonin, neuropharmacologyIntroduction
The pineal gland is responsible for the secretion of melatonin, which primarily serves as a hormone. This compound also known as N-acetyl-5-methoxytryptamine is a methoxyindole derivative that is synthesized from serotonin. It may also exhibit potential autocrine or paracrine properties, especially within the retina or gastrointestinal system [1].
The synthesis of melatonin involves a two-step enzymatic conversion process starting from serotonin. Initially, the conversion of serotonin to N-acetyl serotonin is facilitated by arylalkylamine N-acetyltransferase (AANAT), which serves as the rate limiting step. Following this, melatonin is synthesized by hydroxyindole-O-methyl transferase (HIOMT) [2].
The hormone profile in circulation is indicative of pineal activity, as melatonin is not stored within the pineal gland. Melatonin is predominantly released during the nocturnal period, attaining its peak in plasma concentrations approximately between 02:00 and 04:00. The secretion of melatonin is contingent upon the length of time in which darkness is experienced, and it functions as an intermediary for signals related to darkness, potentially impacting the regulation of circadian rhythms and seasonal variations. Melatonin functions as a messenger of darkness and its role as a sleep signal is not universally consistent, exhibiting variations in interpretation across different animal species [3, 4].
The endogenous clock situated in the suprachiasmatic nucleus (SCN) of the hypothalamus governs the inherent rhythm of melatonin, as well as other circadian rhythms in mammals, including feeding patterns and the sleep-wake cycle [5]. The rhythmic synthesis of melatonin reaches its highest levels during the developmental period spanning from three years to six years of age. Following this, the peak activity during the night gradually diminishes by a maximum of 80% until adulthood and persists in its decline as individuals grow older [1].
The various functions of melatonin are attributed to its ability to readily traverse cell membranes and its widespread distribution of receptors. In addition to manifesting biological effects through distinct membrane receptors, melatonin also engages with other proteins such as calmodulin. Besides its prominent antioxidant effects, melatonin also contributes to a range of physiological functions either directly or indirectly [6, 7].
Melatonin is a more potent free radical scavenger than vitamin E, which is the reference in the field [8]. This action is accomplished through various means. Firstly, melatonin directly neutralizes reactive oxygen and reactive nitrogen species. Secondly, it enhances the activity of antioxidant enzymes while inhibiting the activity of pro-oxidant enzymes [9]. Therefore, pineal melatonin is associated with the perpetuation of oxidative and inflammatory processes, which are known to contribute to the development of various medical conditions such as cancer and neurodegenerative disorders [10–12]. The levels of melatonin produced by the pineal gland exhibit a significant decline of tenfold during the process of aging, spanning from adolescence to the ninth decade of an individual’s life [13]. This decline is closely related to the aging process, and external melatonin administration may slow the deteriorating effects of old age. Regarding this, it has been suggested that melatonin may have potential in delaying and treating age-related diseases [14, 15].
The antioxidative mechanisms of melatonin have also been observed to decrease the toxicity of harmful drugs [16]. This property of melatonin is especially important for counteracting the effects of neurotoxic agents such as methamphetamine [9].
Melatonin is known to have both preventive and therapeutic anticancer properties [17]. It has also been shown that melatonin has the ability to enhance the sensitivity of treatment-resistant cancers to different therapeutic agents [18]. Therefore, it can be said that melatonin is a versatile support agent in cancer treatment [19].
The presence of a significant concentration of melatonin within mitochondria is believed to contribute to its ability to effectively counteract oxidative stress and prevent cellular apoptosis [20]. Experimental studies have also shown that melatonin might exert a mitochondria-targeting antioxidant effect [21].
In this review, the relationship between circadian rhythm and melatonin secretion will be discussed. Then, the association of melatonin with neurotransmitter systems and pharmacologic agents acting through the melatonergic system will be evaluated. Thus, the complex role of melatonin in neurological systems will be elaborated.
Circadian rhythm and melatonin
Time-dependent changes, or fluctuations, are observed in biological functions across various levels, ranging from intracellular organelles to the entire organism. The alterations described are commonly known as “biological rhythms”. These periodic patterns, which exhibit a regularity that can range in duration from milliseconds [as observed in electroencephalography (EEG) or electrocardiography (EKG)] to months (as seen in the menstrual cycle), seasons, and even years (as observed in hibernation among mammals). The aforementioned elements collectively constitute the focal point of the scientific discipline known as “chronobiology” [22]. Rhythms with a duration of less than a day are commonly known as ultradian rhythms (t < 20 h), rhythms with a duration of approximately 24 h are referred to as circadian rhythms (20 h ≤ t ≤ 28 h), and rhythms lasting longer than 28 h are generally classified as infradian rhythms (t > 28 h). Many physiological rhythms observed in humans, such as blood pressure, display circadian characteristics. In the context of infradian rhythms, one can observe periodicities that occur on a weekly (circaseptan), monthly (circavigintan), or yearly (circannual) basis [22].
There are two primary factors hypothesized to be responsible for the aforementioned rhythmicities: first is the exogenous stimuli, also known as exogenous zeitgebers, and the other is endogenous stimuli, also known as endogenous zeitgebers or internal clocks. Exogenous stimuli include factors such as the light/dark cycle, seasons, and meal times. On the other hand, endogenous stimuli are generated internally and include the SCN neural circuits and melatonin, among other factors. Basically, the human circadian rhythm, which operates on a 24-h cycle, is regulated by exogenous zeitgebers. These zeitgebers synchronize human internal clock mechanisms [23, 24].
Circadian rhythms have been discovered throughout many organisms beyond mammals and provide distinct evolutionary benefits in each species. As an example, in the context of bacterial organisms, it is hypothesized that the nocturnal timing of cell division serves as a protective mechanism against the mutagenic impact of ultraviolet radiation [25]. The circadian rhythm additionally plays a crucial role in governing cellular and molecular functions within the body, such as metabolic processes and endocrine functions. The current conviction is that the fundamental basis of the circadian rhythm is a transcriptional molecular clock mechanism that exhibits a high degree of precision with respect to a 24-h cycle, and is ubiquitously present in all cellular entities [25].
SCN, located in the hypothalamus, serves as the primary regulator of the circadian rhythm in mammals. SCN is activated via the retinohypothalamic pathway. This stimulation elicits the activation of neurons through glutamatergic mechanisms, resulting in the entry of calcium ions into the intracellular space. The elevation of intracellular calcium levels initiates transcriptional-translational feedback loops within the genes responsible for governing the molecular clock [26]. The primary feedback loop within this system functions in the following manner: Initially, circadian locomotor output cycles kaput (CLOCK) and brain and muscle ARNT-like protein 1 (BMAL1) proteins establish heterodimers within the neuronal cytoplasm. The CLOCK-BMAL1 heterodimers subsequently translocate into the nucleus where they proceed to interact with the regulatory regions of DNA. Thus, there is an elevation in the expression of period family (PER1, PER2, PER3) and cryptochrome family (CRY1, CRY2) of genes. Following that, the levels of PER and CRY proteins are elevated within the cytoplasm, leading to their formation of heterodimers and subsequent translocation into the nucleus. Once in the nucleus, these proteins exert an inhibitory influence on the CLOCK-BMAL1 complexes. Therefore, they show an inhibitory effect the transcription of genes responsible for their synthesis.
The suppression of melatonin synthesis released by the pineal gland is a significant neuroendocrine response that arises from the activation of the SCN. The compound melatonin, possessing a brief half-life, undergoes rapid elimination from various bodily tissues. Hence, melatonin is regarded as a dependable and consistent indicator of the circadian rhythm [27]. Melatonin is the end product of tryptophan-serotonin pathway as mentioned above. The entrainment of the circadian rhythm in organs is significantly influenced by its role. The reception of melatonin stimulation plays a crucial role in triggering the transition of tissues into a state commonly referred to as “night mode”, while a decrease in melatonin stimulation prompts tissues to shift into a state known as “daytime mode” [28]. The effects of melatonin are mediated by the activation of MT1 and MT2 melatonin receptors. Furthermore, it is widely recognized for its significant antioxidant properties within various bodily tissues [29, 30]. The distribution of melatonin receptors is extensive throughout the central nervous system (CNS) [31]. Research has shown that in addition to its role as a circadian rhythm regulator, melatonin also exerts regulatory effects on neurogenesis, immunomodulation, and metabolism [32]. Melatonin also exhibits regulatory functions on neurotransmitter systems. Such as it hinders the release of dopamine, amplifies the effects of the gamma-aminobutyric acid-ergic (GABAergic) system, regulates the influence of serotonin receptors, and augments the efficacy of opioid analgesics [32]. There is an existing literature that suggests melatonin has an effect on cholinergic neurons [33, 34]. The relationship between the histaminergic system and melatonin also holds a specific importance, primarily because of their substantial roles as immunomodulators (Figure 1) [35].
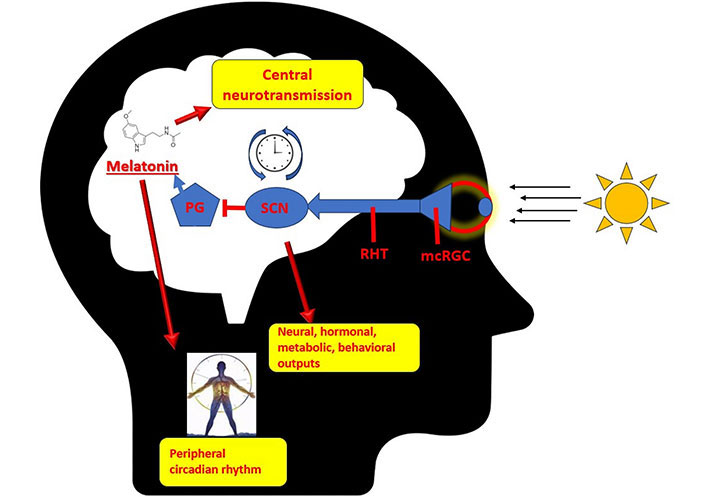
Circadian rhythm and pineal gland. PG: pineal gland; RHT: retinohypothalamic tract; mcRGC: melanopsin-containing retinal ganglion cells
Neurotransmiter systems interacting with melatonin
Serotonergic system
The synthesis of melatonin occurs within pinealocytes through the conversion of the neurotransmitter serotonin. The enzyme AANAT is responsible for the conversion of serotonin into N-acetyls serotonin, which serves as the precursor for melatonin. Additionally, this enzyme plays a regulatory role in the circadian clock [36]. It has been shown that N-acetyl transferase activity increases 150 times with noradrenergic stimulation. Consequently, this heightened enzymatic activity leads to an augmented synthesis of melatonin specifically during the nocturnal period [37]. It is known that the pineal gland exhibits a consistent release of serotonin. This constant release also shows a diurnal pattern, characterized by elevated levels during daylight hours and reduced levels during nighttime [38, 39]. N-Acetyl serotonin is also released from the pineal gland and has been shown to stimulate tropomyosin receptor kinase B (TrkB) receptor-mediated release of brain-derived neurotrophic factor (BDNF) [40]. The primary inference that can be drawn from all this information is that the noradrenergic, serotonergic, and melatonergic systems are closely intertwined and intricately related to each other.
Serotonergic neurons exhibit a strong association with the SCN. SCN receives direct input from serotonergic neurotransmission that originates from the median raphe nucleus. Additionally, it receives indirect input through the neuropeptide Y (NPY) pathway from the intergeniculate leaflet, which is regulated by the dorsal raphe nucleus. Subsequently, the SCN transmits polysynaptic efferent signals to the midbrain serotonergic nuclei, predominantly via the dorsomedial hypothalamus and its associated projections [41]. Both serotonin and NPY are involved in the regulation of the circadian system’s response to light, as well as in the modulation of the central clock’s period and phase through feedback mechanisms related to behavioral arousal and locomotor activity [42, 43]. It is believed that the serotonergic and circadian systems have a clinical correlation. In non-seasonal depression, it has been observed that circadian light therapy enhances and accelerates the therapeutic effects of selective serotonin reuptake inhibitors (SSRIs) [44]. In addition to the effect of light exposure on mood, melatonergic receptor agonists such as agomelatine which is being used to treat depression, further demonstrates the interdependence of both systems [45]. On the other hand, according to studies, melatonin itself does not demonstrate antidepressant properties. However, it has the potential to enhance the effects of other antidepressant medications [46, 47]. In contrast, previous studies have demonstrated that the acute administration of citalopram (SSRI) has the effect of heightening the responsiveness of the circadian rhythm to light stimuli and intensifying the inhibition of melatonin production [48]. Hence, it can be suggested that a reciprocal pharmacological interaction exists between the serotonergic and melatonergic systems. The observation that antidepressants exert a suppressive influence on melatonin, whereas melatonergic agents and melatonin are employed in the management of depression, underscores a paradox that calls for additional clarification [49]. In addition, this point also introduces the potential for chronobiological approaches, which propose that the timing of melatonergic agent administration could impact the effectiveness of depression treatment. A considerable body of research in the fields of pharmacokinetics and pharmacodynamics has demonstrated that the efficacy of numerous antidepressant medications can be influenced by the timing of their administration [50]. Serotonin may also be produced by mitochondria in all cells. This hypothesis places melatonin production at the center of all cellular metabolism. From this point of view, the use of SSRIs may also increase the amount of cellular serotonin that is readily available and accelerate the release of melatonin from all cells, central and peripheral [51]. Although it is difficult to draw definitive and universally applicable conclusions, the interconnectedness of the melatonergic and serotonergic systems can potentially aid in resolving this matter. Additional investigation into the complex interplay among these systems has the potential to yield valuable insights.
Noradrenergic system
Norepinephrine and epinephrine demonstrate a circadian rhythm of secretion within the human body. Plasma concentrations in humans exhibit a decrease during nighttime and an increase during daytime. Furthermore, it has been observed that during the sleep period, there is an additional reduction of approximately 30% in their plasma levels [52]. In contrast, the release of norepinephrine by central sympathetic nerve terminals is 100 times higher at night [30]. This observation suggests that there are variations in noradrenergic rhythms across different physiological regions. Norepinephrine, through the activation of beta-2 receptors, stimulates the synthesis of melatonin from serotonin in the pineal gland, which is characterized by a rich noradrenergic innervation. The prevailing hypothesis proposes that the mechanism underlying this induction is primarily mediated via the cyclic adenosine monophosphate/protein kinase A (cAMP/PKA) signaling pathway [53, 54]. Based on this hypothesis, it is postulated that heightened levels of cAMP, as a consequence of sympathetic noradrenergic stimulation, trigger the phosphorylation of AANAT. This enzyme plays a pivotal role in the synthesis of melatonin. The activation of PKA is responsible for this process. During the period of daylight, when the stimulation of noradrenaline ceases, the degradation of this enzyme occurs rapidly [55]. Therefore, norepinephrine carries a crucial function as a prominent stimulus in triggering the secretion of melatonin during nocturnal periods.
Yet it is also necessary to mention that norepinephrine serves as a central neurotransmitter that plays a role in the regulation of wakefulness. While it is involved in the stimulation of melatonin production, it also plays a crucial role in maintaining wakefulness [56]. The previously mentioned plasma circadian rhythm of norepinephrine serves as an indication of its involvement in the regulation of wakefulness. This role of norepinephrine is closely linked to the presence of light. The light signals originating from the retina that are transmitted to the SCN also project to the dorsal medial hypothalamus (DMH). The dorsomedial hypothalamus transmits these signals to the regions responsible for promoting wakefulness and enhancing arousal, thereby acting as an activating stimulus. The locus coeruleus is a notable region responsible for promoting wakefulness, as it plays a central role in the release of norepinephrine within the brain [56, 57]. Melatonin, on the other hand, acts on the SCN via MT1 receptors, inhibiting the firing of these neurons and promoting sleep induction [56].
Based on the available evidence, it can be suggested that there exists a bidirectional relationship between norepinephrine and melatonin [56]. Norepinephrine plays a role in facilitating the state of wakefulness, both at the physiological level within the body and through central nervous mechanisms that involve the locus coeruleus. Furthermore, it has been observed that it enhances the secretion of melatonin during nighttime via metabolic pathways. The melatonin that is released subsequently inhibits norepinephrine signaling, resulting in a decrease in wakefulness. The complete understanding of the intricate equation presented remains unclear and the investigation into the mechanism by which norepinephrine promotes wakefulness in specific contexts while inducing sleep in others necessitates additional studies.
Dopaminergic system
Dopamine, as a neuromodulator, plays a significant role in regulating both central and peripheral circadian rhythms. Its functions involve locomotor activity, reward mechanisms, and learning processes. Multiple studies have been conducted on both the SCN and peripheral brain regions, yielding a substantial body of evidence indicating that dopamine exerts an impact on neural clock genes and proteins [58].
Dopamine is found in the retina as a neuromodulator and plays an important role in facilitating adaptation to light [59]. Research has demonstrated that dopamine displays diurnal rhythms not only in the retina, but also in the olfactory bulb [60]. It has been shown that the rhythmic functioning of dopamine in the retina is dependent on the presence of melatonin. When melatonin is not present, the regularity of this rhythmic pattern comes to an end [61]. Research has also demonstrated that dopamine, by interacting with D2 receptors, exerts an inhibitory effect on the activity of AANAT. Thus, it is suggested that a reciprocal inhibition exists between melatonin and dopamine within the retina [58]. However, it has been reported that the rhythmic activity of dopamine is not limited to the retina but also occurs in other regions such as the striatum, midbrain, and hypothalamus, exhibiting diurnal activity [58].
It has been demonstrated that melatonin inhibits the hypothalamic release of dopamine. This inhibition is closely associated with the secretion of prolactin and is most pronounced in the tuberoinfundibular pathway [62, 63]. In addition, it has been shown that melatonin inhibits the release of dopamine via the nigrostriatal pathway [64, 65]. Additionally, the inhibitory effect of melatonin on striatal neurons may be associated with the suppression of glutamatergic signaling through N-methyl-D-aspartatic (NMDA) receptors [62]. This circumstance has significant clinical implications. Melatonin, which is being evaluated for its neuroprotective properties in Parkinson’s disease, may exacerbate the condition by inhibiting dopamine release. Animal studies support this hypothesis, and it has even been suggested that melatonin antagonism could be beneficial in the treatment of Parkinson’s disease [62, 66]. Therefore, it is advised to exercise caution when using melatonin in neurodegenerative diseases.
Impairments in melatonin release have been observed both in the pathogenesis of schizophrenia and secondary to antipsychotic drug use. Both decreased melatonin release and phase shift have been reported in schizophrenia patients [67, 68]. Accordingly, the use of melatonin as a supplement may replace the impaired pineal gland function seen in schizophrenia and may also prevent possible adverse effects of antipsychotics [69]. Thus melatonin’s suppressive effects on the dopaminergic system may have therapeutic potential in tardive dyskinesia, which is an adverse effect of antipsychotic use, characterized by involuntary facial and body movements. It is believed that dopamine receptor hypersensitivity contributes to this adverse effect. In treating tardive dyskinesia, the modulatory effect of melatonin on the dopaminergic system may therefore be beneficial. There are positive reports despite some contradictory findings from clinical studies in this area [70–72].
Cholinergic system
Cholinergic systems exhibit a distinct circadian rhythm in mammals. The amount of acetylcholine (ACh) in the brain increases during wakefulness and physical activity. In general, the circadian rhythm in the cholinergic system is characterized by an increase in ACh release during the active phase (dark phase for nocturnal species and light phase for diurnal species), as well as an increase in choline acetyltransferase activity and a decrease in acetylcholinesterase activity. In addition to exhibiting diurnal rhythmicity, the cholinergic system is regarded as an arousal-inducing mechanism [73, 74].
Cholinergic signaling originating from the laterodorsal tegmental (LDT) and pedunculopontine tegmental nuclei (PPT) and the basal forebrain, projecting to the pontine reticular formation, plays a central role in promoting wakefulness. Although cholinergic system activity decreases during non-rapid eye movement (REM) sleep, cholinergic signaling originating from these regions also supports the generation of REM sleep [73, 75]. This phenomenon is explained by two distinct cholinergic transmission pathways originating from the LDT/PPT. One pathway is active during both wakefulness and REM sleep, while the other pathway is only active during wakefulness [76].
It is believed that melatonin, with its protective effect on cholinergic neurons, may be beneficial, especially for dementia patients [77–79]. Extensive research has been conducted on the possible protective effects of exogenous melatonin in dementia patients, but the current understanding of the cross-talk between endogenous melatonin and cholinergic neurons is limited. Endogenous nocturnal melatonin release improves the function of nicotinic ACh receptors, according to one study [78]. The authors of this study have linked this finding to the theory that the age-related decline in melatonin release may contribute to the degeneration of cholinergic neurons [78]. On the other hand, melatonergic stimulation has been shown to increase the synaptic activity and expression of nicotinic ACh receptors. This finding provides an important clue as to the mechanisms through which melatonin protects and strengthens cholinergic neurons [78]. In addition, an experimental study suggested that melatonin may contribute to the protection of the duodenal barrier by enhancing nicotinic ACh receptor-mediated cholinergic stimulation from the vagal nerve. This finding may also be associated with the potentiating effect of melatonin on cholinergic stimulation [80]. Also, it must be noted that tacrine-melatonin hybrid therapy which is being developed against dementia, increases cholinergic transmission both by inhibiting acetylcholinesterase and strengthening cholinergic neurotransmission [81].
The observation that cholinergic agents activate specific neurons in the SCN provides insight into the relationship between the central centers that regulate circadian rhythm and cholinergic mechanisms [82]. It has been also observed that the administration of the ACh receptor agonist carbachol produces effects similar to the influence of light in the SCN [83]. This relationship, however, is believed to be indirectly related to the light-SCN relationship and originates from a modulatory function of ACh [84].
When all this information is considered, it becomes clear that ACh has a close connection with circadian rhythm and sleep. However, no direct cross-talk or projection between melatonin and the cholinergic system has been identified. Given the substantial protective and strengthening effect of melatonin on cholinergic neurons and the influence of cholinergic neurons on circadian rhythms and sleep, it is remarkable that there is no direct interaction between these two systems has been found yet. Additional research is required to investigate this uncharted area of the literature.
Histaminergic system
The tuberomamillary nucleus (TMN) of the posterior hypothalamus is the primary location of histaminergic neurons. This nucleus projects to numerous regions of the CNS, including the arousal-inducing regions of the basal forebrain and the orexin-containing lateral hypothalamus [85].
Histamine produces its effects via four G protein-coupled receptors, three of which are expressed in the CNS (H1, H2, and H3). Particularly, H1 and H3 receptors play a significant role in sleep promotion in the CNS. The majority of antihistamines induce sedation by blocking H1 receptors, whereas the H3 inverse agonist pitolisant was recently approved by the Food and Drug Administration (FDA) for treating insomnia [86].
The circadian rhythm modulates the expression of the histidine decarboxylase (HDC) gene, which encodes the most important enzyme in histamine synthesis. During active hours, HDC expression increases, whereas during rest hours, HDC expression can drop below detectable levels in certain cells [86]. The circadian changes in HDC gene expression have been observed to be absent in “Bmal1” gene knockout mice, which is one of the central genes governing the circadian rhythm [87]. Furthermore, it has been shown that in HDC knockout mice, although the expression of clock genes remains intact in the SCN, it is significantly disrupted in regions such as the cortex and striatum [88].
The disruption of the sleep-wake cycle observed in zebrafish lacking melatonin production (AANAT knockout) has been reversed by treatment with the H1 receptor antagonist pyrilamine in a study [89]. This finding suggests the presence of a central communication between histamine and melatonin [35].
Mast cell activation is an additional critical site where melatonin and histamine release intersect. It has been demonstrated that the MT1 and MT2 receptors found on mast cells have anti-inflammatory effects by preventing the release of histamine, and that the circadian rhythm influences this process [35, 90–92]. The investigation of the intersection points between the histaminergic and melatonergic systems presents a compelling subject for academic study, offering potential insights into the intricate connection between sleep and immunity.
Experimental production of dual-acting ligands that exert their effects via histamine and melatonin pathways is underway [93]. These agents possess the capacity to influence various pathologies by means of both neuropsychiatric and immunological mechanisms, thereby providing insights into certain physiopathological pathways that remain incompletely understood.
Psychopharmacological treatment approaches that effect through the melatonergic system
Conducting an analysis of the pharmacological agents that exert an influence on the melatonergic system subsequent to an exploration of the neurotransmitter systems engaged in reciprocal interactions with the melatonergic system would provide valuable insights. The agents encompassed in this category consist of ramelteon, a melatonin MT1/MT2 receptor agonist, and agomelatine, a compound that acts as both an MT1/MT2 receptor agonist and a 5HT2C serotonin receptor antagonist. Although these drugs are approved for the treatment of conditions such as insomnia and depression, their interactions with the melatonergic system are complex, suggesting the possibility of unexplored effects via alternative pathways. This section focuses on the subject of potential therapeutic applications of approved drugs (ramelteon, tasimelteon, agomelatine) and newly investigated melatonergic agents. Information regarding these pharmacological agents are summarized in Table 1.
Pharmacologic agents show effect through the melatonergic system
Pharmacologic agent | Mechanism of action | Approved indications | Research areas | References |
---|---|---|---|---|
Ramelteon | MT1 and MT2 agonism | Insomnia | Alzheimer’s disease Delirium Cancer | [94–106] |
Agomelatine | MT1 and MT2 agonism 5HT2C antagonism | Major depressive disorder | Anxiety disordersAlzheimer’s disease Cancer Endothelial dysfunction | [107–133] |
Tasimelteon | MT1 and MT2 agonism SCN pacemaker regulation | Non-24-h sleep-wake disorder | Alzheimer’s disease Sleep quality Jet lag | [134–136] |
Neu-P11/Piromelatine | MT1 and MT2 receptor agonism 5HT1A/1D receptor agonism | None | Sleep disorders Depression Anxiety Alzheimer’s disease Insulin resistance type 2 diabetes | [137–145] |
M6B, M7C | MT1 and MT2 agonism | None | Panic disorders Epilepsy | [139, 146] |
UCM765 | Selective MT2 agonism | None | Non-REM sleep disorders Anxiety | [137, 147, 148] |
TIK-301 | MT1 and MT2 agonism 5HT2B/5HT2C antagonism | Orphan drug status for circadian rhythm sleep disorders in blind individuals without retinal light exposure | Depression Epilepsy | [139, 149] |
Ramelteon
Ramelteon is a synthetic analogue of melatonin. The compound functions as an agonist on melatonin MT1 and MT2 receptors. The oral bioavailability of it is quite limited, approximately 2%. This medication is employed for the therapeutic management of insomnia and does not exhibit the development of tolerance towards its sleep latency-reducing efficacy. No withdrawal effects have been observed. The half-life is 1–2 h [94, 95]. It has been shown in animal experiments that acute use does not impair memory, learning, and motor function [96, 97]. It has also been reported in animal experiments that it does not have rewarding and addictive effects [96]. The adverse effects observed in clinical use, such as drowsiness, dizziness, and fatigue, are generally mild to moderate and have been reported in a small number of patients [95].
In Alzheimer’s patients, a significant decrease in melatonin levels and degeneration of SCN neurons have been observed [95, 98]. Moreover, a number of clinical studies have provided evidence supporting the substantial therapeutic benefits of melatonin in the management of sleep and circadian disruptions among individuals diagnosed with Alzheimer’s disease [99]. Melatonin is also well known to have significant neuroprotective effects [100]. Due to ramelteon’s higher receptor affinity and longer half-life, it has been suggested that it may have potential benefits in the treatment of dementia. According to reports in the scientific literature, ramelteon shows neuroprotective properties comparable to melatonin in Alzheimer’s disease patients [101]. On the other hand, it is suggested that ramelteon, similar to melatonin, may increase the levels of BDNF and other neurotrophic factor levels [102].
Ramelteon’s potential areas of use are not limited to dementia alone. A study has reported its potential preventive effect on the development of delirium [103]. Moreover, interestingly, ramelteon has been shown to suppress endometrial cancer cells in vitro, and in another study, both agomelatine and ramelteon have demonstrated the ability to suppress glioblastoma cells [104, 105].
In spite of ramelteon’s limited antioxidant efficacy, the findings regarding its tumor-suppressing effects are noteworthy [106]. The intracellular pathways and mechanisms through which the selective activity on MT1/MT2 receptors may exert tumor-suppressive or neurotrophic factor-enhancing effects independent of the antioxidant effect remain unclear.
Agomelatine
Agomelatine is an MT1/MT2 receptor agonist and a 5HT2C antagonist. Its half-life is 2.3 h [107]. Its bioavailability from oral route is less than 5% [108]. It is believed that the drug’s dual action on melatonin and serotonin receptors creates a synergistic effect against mood disorders. Considering the close association between circadian rhythm disturbances and depression, this relationship is particularly important [109].
Agomelatine has been shown to exhibit circadian rhythm synchronizing effects similar to melatonin, as demonstrated in animal experiments conducted in constant darkness [110–112]. It has also remarkably been reported that agomelatine can modulate disrupted sleep-wake cycles and circadian rhythms in animals even when the circadian rhythm is disrupted by depression models and not by light exposure [113]. In addition, a clinical study showed that agomelatine increased slow-wave sleep duration in depressed patients without affecting REM sleep duration [114].
In addition to its circadian rhythm-regulating properties, agomelatine is notable for its antidepressant properties. It is important to note that, unlike agomelatine, melatonin alone has no antidepressant effects [115]. Animal studies utilizing various depression models, such as the forced swim model, learned helplessness model, chronic moderate stress model, psychosocial stress model, and genetic models, have demonstrated the effectiveness of agomelatine in treating depression in a variety of experimental settings [116]. In addition, many animal studies have demonstrated anxiolytic effects at doses where antidepressant effects are observed [116, 117]. The anxiolytic effect has also been demonstrated in clinical trials [118, 119]. The antagonism of 5HT2C plays an essential role in the development of the aforementioned antidepressant and anxiolytic effects by increasing dopamine and norepinephrine in the prefrontal cortex [120].
On one hand, it has been demonstrated that agomelatine may improve cognitive performance regardless of depressive disorder [121]. This can be associated with research findings that demonstrate the potential of agomelatine to enhance neurotrophic factors [122, 123]. On the other hand, the neurogenesis enhancing effect of agomelatine may contribute to its therapeutic effects in the treatment of depression which is a disorder characterized by neuronal and glial cell loss and reduced neuroplasticity [124, 125].
Given the abundance of research supporting cognitive function enhancing effects, it is worth noting that positive outcomes have also been observed in studies regarding Alzheimer’s disease. The findings of an animal study indicate that agomelatine exhibits the capacity to mitigate depressive behaviors induced by amyloid-beta and effect the accumulation of amyloid-beta in the hippocampus [126]. Additionally, it has been demonstrated that agomelatine can reduce amyloid-beta phosphorylation in cell culture [126]. Also in clinical studies regarding dementia, positive effects of agomelatine on psychiatric symptoms have also been observed [127]. All of these findings suggest that, similar to ramelteon, agomelatine has significant therapeutic potential for Alzheimer’s disease and dementia.
It is also believed that agomelatine could also be helpful to improve specific psychiatric conditions, such as apathy and anhedonia, in addition to depression, anxiety, and concomitant sleep disorders [120]. In addition, there have been reports that agomelatine exhibits suppressive effects on cancer cells in cell culture [128–130]. It has also been reported that agomelatine reduces endothelial and mononuclear cell adhesion induced by angiotensin-2, thereby possessing a positive potential in endothelial vascular pathologies. This suggests that agomelatine may have beneficial effects on endothelial dysfunction-related conditions [131]. These findings form pharmacological clues that indicate there are still unresolved aspects of the physiology of melatonergic receptors.
In addition to all of these advantages, it is essential to remember that agomelatine may cause elevated liver enzymes and is contraindicated in patients with elevated liver enzymes [132, 133].
Tasimelteon
Tasimelteon is a melatonin analog that received FDA approval in 2014 for the treatment of non-24-h sleep-wake disorder [134]. This medication is a full agonist for melatonin MT1 and MT2 receptors and is known to reset pacemaker cells in the SCN. It has a threefold greater affinity for MT2 receptors than MT1 receptors [134]. No interactions with other receptors have been observed [135]. Based on the findings of the tasimelteon for non-24-hour sleep-wake disorder in totally blind people (SET and RESET) clinical trial, this medication is recommended for the treatment of sleep disorders in completely blind individuals. In general, it is well-tolerated and does not carry a significant risk of adverse effects [136].
Novel aspects in melatonergic system pharmacology
The brief half-life and poor oral bioavailability of melatonin limit its potential as a standalone drug. The effects of melatonin on the chronobiological cycle, sleep, neuroprotective mechanisms, cognitive function, and immunomodulation, however, have prompted decades of research into new agents that act through the melatonergic system. The objective of these efforts is to create novel agents that exert their effects via the melatonergic system [137].
The first agent among these compounds is Neu-P11, also known as piromelatine, which acts as a melatonin MT1/MT2 receptor agonist and serotonin 5HT1A/1D receptor agonist [138, 139]. Multiple animal studies have demonstrated that this agent enhances the quality of sleep, possesses antidepressant and anxiolytic properties, and increases insulin sensitivity [140, 141]. In addition, cognitive enhancement and neuroprotective effects of it have been observed, and it is believed to have treatment potential for Alzheimer’s disease [142, 143]. Furthermore, protective effects of this molecule against type 2 diabetes have been particularly highlighted in recent years [144, 145].
Another example of novel agents are melatonin analogs named M6B and M7C. It is indicated that besides their sedating and anxiolytic effects, they may also exhibit antiepileptic activity [139, 146].
UCM765 is another new agent that acts as an MT2 selective agonist, and it has been shown to enhance non-REM sleep [147]. Furthermore, another study reports that this molecule may also produce anxiolytic effects [148]. The selective agonism of the MT2 receptor, in particular its isolated effects on non-REM sleep, generates a promising research area for the treatment of sleep disorders [137].
TIK-301 is a melatonergic agent that exhibits MT1/MT2 agonism along with 5HT2B/5HT2C antagonism. It was granted orphan drug status by the FDA in 2003 for the diagnosis of circadian rhythm sleep disorders in blind individuals without retinal light exposure [139]. Additionally, it is suggested that TIK-301 exhibits antidepressant effects due to its affinity for serotonin receptors [149]. There is also a theoretical suggestion that this agent (TIK-301) may exhibit anticonvulsant effects [139].
Conclusions
The circadian rhythm is a fundamental mechanism that plays a pivotal role in regulating the physiological functions of an organism in accordance with the light-dark cycle of both central and peripheral functions. The release of melatonin from the pineal gland, which is mediated by the SCN, is a central regulator of this mechanism. The release of melatonin and the regulation of circadian rhythm exhibit a strong association with multiple neurotransmitter systems within the CNS. The melatonergic system can be influenced by various neurotransmitters, including serotonin, dopamine, norepinephrine, and histamine, which can have both stimulatory and inhibitory effects. Furthermore, a strong correlation exists between cholinergic stimulation and the SCN, although the precise details of these neuronal projections remain poorly understood. Each of these pathways presents a prospective pharmaceutical target for numerous diseases that are mediated centrally. Presently, melatonergic medications have received approval solely for the treatment of insomnia, jet lag, and depression that is accompanied by sleep disorders. However, it is worth noting that the melatonergic system has been found to potentially possess therapeutic properties in a range of conditions, including neurodegenerative diseases, neuropsychiatric disorders, dementia, autoimmune diseases, allergic diseases, and epilepsy. Therefore, the ongoing procedure of synthesizing and evaluating novel ligands in this particular area persists. This review offers an in-depth investigation of the various effects associated with the melatonergic system, as well as potential new opportunities for therapeutic interventions.
Abbreviations
AANAT: |
arylalkylamine N-acetyltransferase |
ACh: |
acetylcholine |
BDNF: |
brain-derived neurotrophic factor |
BMAL1: |
brain and muscle ARNT-like protein 1 |
CLOCK: |
circadian locomotor output cycles kaput |
CNS: |
central nervous system |
FDA: |
Food and Drug Administration |
REM: |
rapid eye movement |
SCN: |
suprachiasmatic nucleus |
SSRIs: |
selective serotonin reuptake inhibitors |
Declarations
Author contributions
UA, MCG and GP: Conceptualization, Investigation, Methodology, Writing—original draft, Writing—review & editing. CU: Conceptualization, Investigation, Methodology, Supervision, Writing—original draft, Writing—review & editing.
Conflicts of interest
The authors declare that they have no conflicts of interest.
Ethical approval
Not applicable.
Consent to participate
Not applicable.
Consent to publication
Not applicable.
Availability of data and materials
Not applicable.
Funding
Not applicable.
Copyright
© The Author(s) 2023.